Abstract
Objective
To investigate the effects of customized biomechanical foot orthosis (BFO) on kinematic data during gait in patients with hallux valgus (HV) deformities and compare the results with those of a normal control group.
Methods
Ten patients with HV deformities and 10 healthy volunteers were enrolled in this study. HV deformity was diagnosed using biomechanical and radiological assessments by a rehabilitation physician. Patients received the customized BFO manufactured at a commercial orthosis laboratory (Biomechanics, Goyang, South Korea) according to the strictly defined procedure by a single experienced technician. The spatiotemporal and kinematic data acquired by the Vicon 3D motion capture system (Oxford Metrics, Oxford, UK) were compared between the intervention groups (control vs. HV without orthosis) and between the HV groups (with vs. without orthosis).
Results
The temporal-spatial and kinematic parameters of the HV group were significantly different from those of the control group. After applying BFO to the HV group, significantly increased ranges of plantar flexion motion and hindfoot inversion were observed. Furthermore, the HV group with BFO showed improved gait cadence, walking speed, and stride length, although the results were not statistically significant.
Hallux valgus (HV) deformity remains one of the most common and disabling pathologies of the feet [1]. A wide range of prevalence estimates for HV has been presented in various independent reports. National health surveys in the United States have reported a prevalence rate of 0.9% across all age groups [2], while a more recent survey in the United Kingdom reported a prevalence rate of 28.4% in adults [3]. Research conducted in the older population has indicated prevalence rates as high as 74% [3].
In HV, local tenderness occurs due to abnormal pressure distribution during walking. In addition, foot deformities, such as subluxation of the first metatarsophalangeal joint, can develop, which eventually results in changes in gait patterns and abnormal movement of the center of pressure [4]. As the range of motion of the first metatarsophalangeal joint increases, the weight-bearing capacity of the metatarsal head, which is applied during walking, decreases. Such abnormal weight-bearing causes pain in the metatarsal head, thus making walking difficult. The development of an abnormal gait pattern increases physical stresses on the joint, resulting in a vicious cycle of further exacerbating deformity and pain [5].
Menz and Lord [6] identified significantly reduced walking speed and step length in patients with HV. In addition, Nix et al. [1] reported that HV could reduce the ability to walk and quality of life and has been shown to increase fall risk in elderly individuals. However, because the feet are covered by shoes and socks, its early diagnosis is difficult, and appropriate treatment is difficult even if symptoms are present. Since foot deformity induces biomechanical changes in standing and gait, it can cause asymmetry of the pelvis, pain in the knee joint, and pain and deformity of the spine [4]. Therefore, appropriate intervention to prevent disability is necessary.
The main goal of HV treatment is to control pain, improve walking capacity, and restore cosmetic appearance. Surgery and wearing orthosis are the main treatment options [7]. More than 100 surgical treatment techniques have been introduced for the correction of HV. Distal metatarsal osteotomy is widely used to correct mild or moderate HV. However, recurrences or under-corrections have been reported in 10%–14% of the cases [8].
To reduce the angle of the first metatarsal great toe joint, conservative treatments such as inserting a pad or wedge and wearing a soft or hard insole are used [9]. According to Charrette [10], an HV orthosis acts as a biomechanical support meant to reduce pressure on the first metatarsal joint, preventing further degradation of mobility. A systematic review reported that deformity was reduced after wearing a foot orthosis in HV patients alongside an improvement in pain [11]. However, much less is known about the kinematic behavior of the foot affected by HV [12]. Kinematic analysis of the foot has been challenging for many years, partially because of technological limitations and also due to the intrinsic complexity of the foot.
Therefore, in this pilot study, we investigated the effects of a customized biomechanical foot orthosis (BFO) on kinematic data obtained through three-dimensional (3D) gait analysis during gait with HV deformities in comparison with a normal control group.
From March 2016 to November 2016, 20 participants were enrolled in this study. Ten subjects in the patient group were newly diagnosed with HV deformities involving pain in the outpatient department of rehabilitation medicine at Chungnam National University. The diagnostic criteria were as follows: hallux valgus angle (HVA) ≥15° and intermetatarsal angle (IMA) ≥9° on weight-bearing anteroposterior (AP) plain radiographs (Fig. 1). The control group included 10 healthy volunteers. In both groups, individuals with a history of major lower-limb musculoskeletal trauma or surgery and congenital conditions that may affect gait were excluded. Gait examinations were performed in both groups while the subjects wore shoes. Only the HV group underwent additional examination with BFO in shoes after 1-hour light walking for adaptation with the orthosis.
The study protocol was approved by the Institutional Review Board of Chungnam National University Hospital (No. IRB 2015-03-040), and informed consent was obtained from all participants.
Spatiotemporal and kinematic data were obtained using the Vicon MX system (12 infrared cameras; Vicon Motion Systems, Oxford, UK).
Twelve infrared cameras (Vicon MX) with sampling at 100 Hz were used to track the marker positions. Three walking trials were undertaken, approximately 5 m in length, with individuals walking at a self-selected speed. To quantify the foot kinematics of both groups, the Oxford foot model (OFM) was applied, as described by Stebbins et al. [13]; reflective markers with a diameter of 14 mm were positioned at anatomical landmarks.
The calculated parameters, using the OFM, were as follows:
(1) hallus relative to the forefoot : dorsiflexion/plantarflexion (sagittal plane),
(2) forefoot relative to the hindfoot: dorsiflexion/plantarflexion (sagittal plane), supination/pronation (coronal plane), abduction/adduction (transverse plane), and
(3) hindfoot relative to the tibia: dorsiflexion/plantarflexion (sagittal plane), inversion/eversion (coronal plane), adduction/abduction (transverse plane).
The relative motion for each gait phase, defined as the difference between the maximum and minimum angles during each phase, was calculated. The dorsiflexion, inversion, supination, and adduction motions are denoted by positive, and the plantarflexion, eversion, pronation, and abduction motions are denoted by negative.
A total of 15 markers were used.
- The HEE marker should be placed on the bisection of the calcaneus line, as far down the calcaneus as feasible when considering dynamic trials. It should be at the same height from the plantar surface of the foot as the P5M marker.
- The CPG marker should be placed on the line above the HEE marker. The base of the marker should be on the line, and the marker should reflect the varus/valgus alignment of the heel.
- The PCA marker should be placed on the same line above the base of the CPG marker.
- The LCL and MCL markers should be placed on the lateral and medial aspects of the calcaneus, respectively.
- The D1MT marker should be placed on the head of the first metatarsal, and the D5MT marker should be placed on the head of the fifth metatarsal.
- The P5MT marker should be placed on the base of the fifth metatarsal, at the same height from the plantar surface of the foot as the markers on the metatarsal heads.
- The P1M marker should be placed on the base of the first metatarsal.
- The HLX marker should be placed on the hallux, at the same height as the D1M marker, on the proximal end of the distal phalanx or the distal end of the medial phalanx.
- The TTU marker should be placed on the tibial tuberosity.
- The SH marker should be placed on the anterior crest of the tibia (Fig. 2).
Customized BFOs were fabricated from a neutral suspension cast using the bisection of the calcaneus according to the technique of Root et al. [14]. The BFO was manufactured at a commercial orthosis laboratory (Biomechanics, Goyang, Korea) according to a strictly defined procedure by a single experienced technician. The semirigid polypropylene material was used to fabricate the BFO, of which the control points were the poron kinetic wedge on a deflection at the first metatarsal head, the metatarsal dome, and the medial arch support. A subtalar neutral suspension cast using the calcaneus bisection was used to correct excessive pronation (Fig. 3).
To analyze the differences in the demographic and temporal parameters of both groups, the non-parametric Mann-Whitney U-test was used. For the statistical analysis of kinematic data, the relative motion occurring at each segment angle was calculated for seven gait phases [15]. Differences in kinematic data between the groups (control vs. without orthosis) and with versus without orthosis in the HV group were considered significant at a p-value of <0.05, using the non-parametric Mann-Whitney U-test.
The demographics of the study population and clinical score results are presented in Table 1. There were no significant differences in age, weight, and height between the experimental and control groups. The radiographic results of the HV group showed a mean IMA of 13.5° and an HVA of 32.3°.
The HV group demonstrated a significantly slower walking speed and cadence and shorter stride and step length, while a significant increase in double support was observed compared with that in the control group. With BFO, significant differences compared to the data without orthosis were not evident in the temporal-spatial parameters (Table 2).
The relative intersegment angle was assessed, and a comparative analysis was performed between the HV group with BFO, HV group without BFO, and control group (Table 3, Fig. 4).
The HV group demonstrated a significantly increased range of dorsiflexion in the terminal stance and terminal swing phases compared with that in the control group. After applying BFO, a significantly increased range of plantar flexion motion was observed.
In the HV group, an increased range of dorsiflexion motion during the mid-swing phase was observed compared with that in the control group. There was no significant change in the forefoot kinematics after applying BFO.
During the mid-stance, a decrease in hindfoot inversion was observed in the HV group. In addition, significant increases in hindfoot eversion during pre-swing were observed in the HV group. With BFO, a significantly increased range of hindfoot inversion was observed during the pre-swing phase.
The present study investigated the kinematic characteristics of the foot segment in patients with HV during walking and how biomechanical orthotic treatment can affect foot kinematics. When comparing the HV group to the control group, the results showed significant alterations in a number of gait parameters; some of these parameters improved, similar to a normal gait pattern, when wearing a customized BFO.
First, the measurements obtained from the control group were similar to values reported in the literature for healthy populations regarding both the spatiotemporal and angular parameters [16,17]. For the HV group, the spatiotemporal results of this study, reduced walking speed, decreased stride length, and increased cadence, were comparable to those in previously reported studies [16,17]. This result can be explained by the alteration of normal biomechanics, alteration of weight transfer, increasing energy consumption following pain, and inadequate push-off. No significant improvement in the spatiotemporal parameters was observed after wearing BFO. However, the duration of wearing BFO was not sufficient to change the gait pattern. It is necessary to perform a long follow-up study on the biomechanics and gait pattern change to draw objective conclusions.
In the HV group, we found an increased range of hallux dorsiflexion in the terminal stance and mid-swing phases compared with that in the control group. The increased dorsiflexion motion was probably due to the hallux, first metatarsal malalignment following the earlier onset of the dorsiflexion movement; therefore, inadequate push-off prevented efficient gait. Chopra et al. [18] suggested that reduced mobility at the first metatarsophalangeal joint in the terminal stance could justify the reduced toe-off pitch angle and peak swing speed along with the incomplete push-off phase observed in our patient group.
We also found a difference in the hindfoot-tibia angle during mid-stance and during the pre-swing of the stance phase. The HV group showed a decrease in hindfoot inversion during mid-stance, increased eversion, and decreased plantarflexion motion of the hindfoot, which corresponds to studies on kinematic HV deformity performed by Deschamps et al. [12]. These relative motions can be explained by excessive subtalar joint eversion, which unlocks the midtarsal joint and leads to kinematic abnormalities, mainly in the push-off period.
With the application of BFO, several kinematic deviations observed in HV were corrected. A significantly increased range of plantarflexion motion in the hallux relative to the forefoot in the terminal stance and mid-swing phase was observed. In addition, although not statistically significant, an increased range of hindfoot inversion and plantar flexion relative to the tibia was observed during the pre-swing phase. In our study, the BFO was a functional customized foot orthosis fabricated using the root technique. A subtalar neutral suspension cast using the calcaneus bisection was used to correct excessive pronation. The components included the poron wedge at the first metatarsophalangeal joint, metatarsal pad, and longitudinal arch support. Owing to these control points, it is suggested that after wearing an orthosis, gait could be similar to normal gait kinematics. In a previous study, Becerro de Bengoa Vallejo et al. [19] reported that a rootstyle orthosis induced significantly greater first metatarsal plantar flexion than walking without orthosis.
Abnormalities in spatiotemporal and kinematic gait parameters are known to be related to the risk of falls, which are more valuable than plantar pressure; therefore, efforts to improve spatiotemporal and kinematic parameters are as important as correcting the pressure distribution [15,20].
Despite the important findings, our study had some limitations. First, a limited number of female participants were included in order to generalize the results. Second, kinetic analysis was not performed; more indepth evidence can be provided for the interpretation of changes in gait biomechanics using kinetic data. Third, we attached a marker to the slipper. Fixation of a marker at an adequate anatomical landmark should address the reliability of foot motion tracking. To overcome this problem, the subjects used adequately fitted shoes and were instructed to tighten the shoelace while walking. Moreover, since our study only analyzed the immediate effect of wearing the foot orthosis, long-term follow-up observation is necessary for consideration of the improvement in pain and biomechanical improvements of the lower extremities.
In conclusion, this study showed that patients with HV had a different intersegment range of motion in contrast to healthy subjects. Our results suggest that the application of a customized BFO will be useful for improving kinematics in HV deformities.
Notes
Conceptualization: Lee JY, Ahn SY, Bok SK. Methodology: Lee JY, Bok SK. Formal analysis: Lee JY, Ahn SY, Bok SK. Funding acquisition: Ahn SY, Bok SK. Project administration: Bok SK. Visualization: Lee JY, Ahn SY. Writing - original draft: Lee JY, Ahn SY. Writing - review and editing: Lee JY, Ahn SY, Bok SK. Approval of final manuscript: all authors.
REFERENCES
1. Nix SE, Vicenzino BT, Collins NJ, Smith MD. Gait parameters associated with hallux valgus: a systematic review. J Foot Ankle Res. 2013; 6:9.
2. Adams PF, Hendershot GE, Marano MA; Centers for Disease Control and Prevention/National Center for Health Statistics. Current estimates from the National Health Interview Survey, 1996. Vital Health Stat 10. 1999; (200):1–203.
3. Nix S, Smith M, Vicenzino B. Prevalence of hallux valgus in the general population: a systematic review and meta-analysis. J Foot Ankle Res. 2010; 3:21.
4. Jang KS, Kim TW, Kim HJ. Diagnosis and Pathophysiology of hallux valgus. J Korean Foot Ankle Soc. 2014; 18:43–7.
5. Shih KS, Chien HL, Lu TW, Chang CF, Kuo CC. Gait changes in individuals with bilateral hallux valgus reduce first metatarsophalangeal loading but increase knee abductor moments. Gait Posture. 2014; 40:38–42.
6. Menz HB, Lord SR. Gait instability in older people with hallux valgus. Foot Ankle Int. 2005; 26:483–9.
7. Torkki M, Malmivaara A, Seitsalo S, Hoikka V, Laippala P, Paavolainen P. Surgery vs orthosis vs watchful waiting for hallux valgus: a randomized controlled trial. JAMA. 2001; 285:2474–80.
8. Klugarova J, Janura M, Svoboda Z, Sos Z, Stergiou N, Klugar M. Hallux valgus surgery affects kinematic parameters during gait. Clin Biomech (Bristol, Avon). 2016; 40:20–6.
9. Lee SH, Lee YC. Approach for the treatment on hallux valgus. J Korean Foot Ankle Soc. 2019; 23:143–8.
10. Charrette M. Bunion formation and orthotic support. Dyn Chiropr. 2009; 27:1–3.
11. Kwan MY, Yick KL, Yip J, Tse CY. Hallux valgus orthosis characteristics and effectiveness: a systematic review with meta-analysis. BMJ Open. 2021; 11:e047273.
12. Deschamps K, Birch I, Desloovere K, Matricali GA. The impact of hallux valgus on foot kinematics: a cross-sectional, comparative study. Gait Posture. 2010; 32:102–6.
13. Stebbins J, Harrington M, Thompson N, Zavatsky A, Theologis T. Repeatability of a model for measuring multi-segment foot kinematics in children. Gait Posture. 2006; 23:401–10.
14. Root ML, Weed JH, Orien WP. Neutral position casting techniques. Los Angeles: Clinical Biomechanics Corporation;1971.
15. Hamacher D, Singh NB, Van Dieen JH, Heller MO, Taylor WR. Kinematic measures for assessing gait stability in elderly individuals: a systematic review. J R Soc Interface. 2011; 8:1682–98.
16. Canseco K, Long J, Marks R, Khazzam M, Harris G. Quantitative characterization of gait kinematics in patients with hallux rigidus using the Milwaukee foot model. J Orthop Res. 2008; 26:419–27.
17. Canseco K, Rankine L, Long J, Smedberg T, Marks RM, Harris GF. Motion of the multisegmental foot in hallux valgus. Foot Ankle Int. 2010; 31:146–52.
18. Chopra S, Moerenhout K, Crevoisier X. Characterization of gait in female patients with moderate to severe hallux valgus deformity. Clin Biomech (Bristol, Avon). 2015; 30:629–35.
19. Becerro de Bengoa Vallejo R, Sanchez Gomez R, Losa Iglesias ME. Clinical improvement in functional hallux limitus using a cut-out orthosis. Prosthet Orthot Int. 2016; 40:215–23.
20. Callisaya ML, Blizzard L, Schmidt MD, McGinley JL, Srikanth VK. Ageing and gait variability: a population-based study of older people. Age Ageing. 2010; 39:191–7.
Fig. 2.
Gait analysis. (A) Walking at self-selected speed along a 5-m walkway. (B) The anantomical landmarks. (C) Reflective markers in patient.

Fig. 3.
Customized biomechanical foot orthosis (BFO). (A) 1st metatarsal head wedge (red circle). (B) Metatarsal dome pad (white circle). (C) Longitudinal medial arch support (yellow circle).
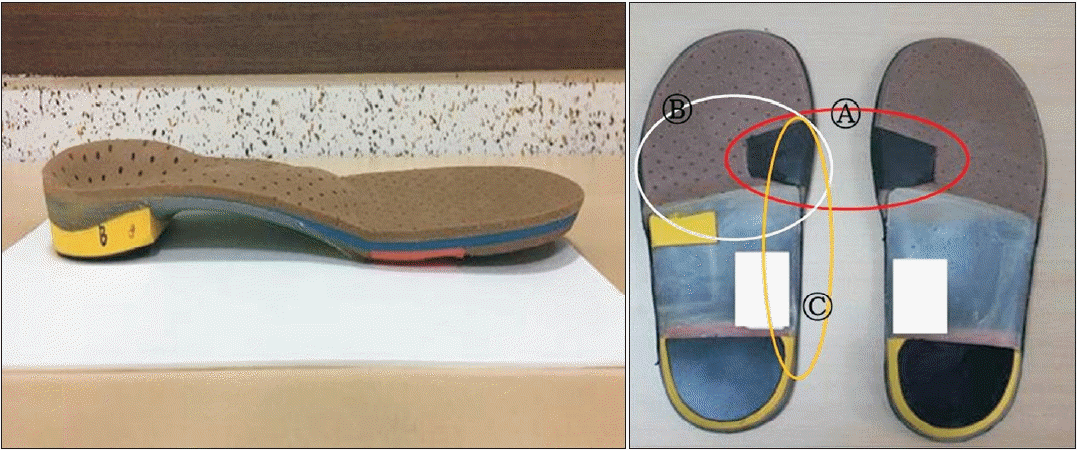
Fig. 4.
Foot kinematics during whole gait cycle. (A) hallux-forefoot dorsifexion/plantarflexion. (B) hindfoot-tibia inversion/ eversion. BFO, biomechanical foot orthosis.
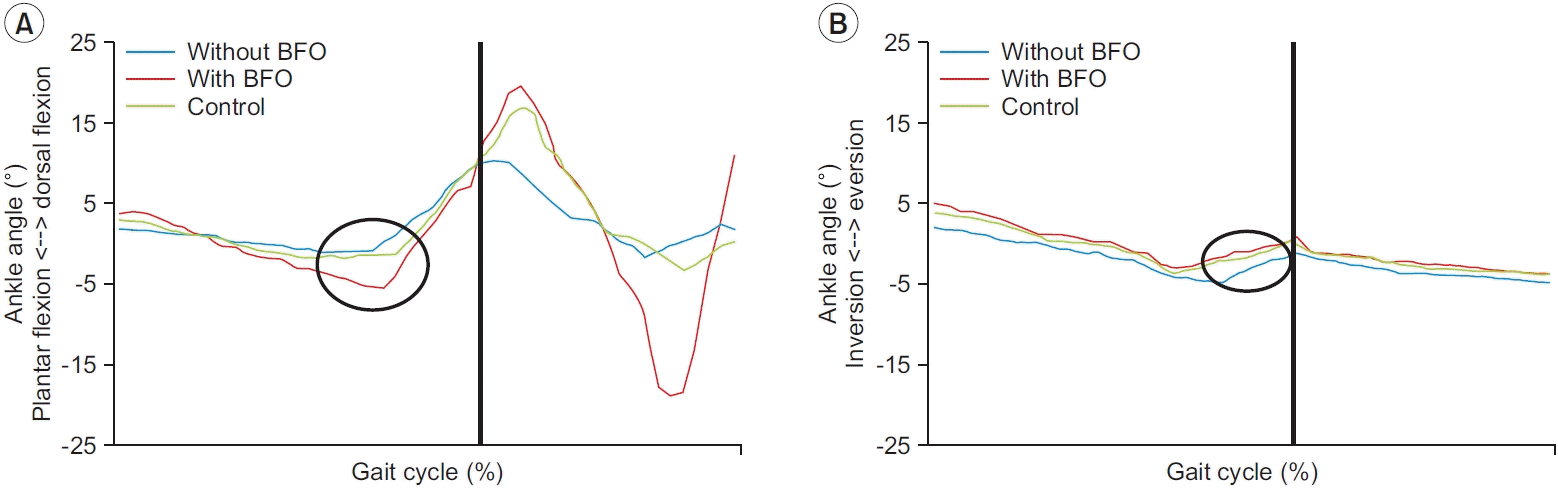
Table 1.
Demographics
Control group (n=10) | HV group (n=10) | |
---|---|---|
Sex, female | 10 | 10 |
Age (yr) | 52.0±24.6 | 59.0±8.5 |
Height (m) | 159.6±5.8 | 157.2±4.2 |
Weight (kg) | 55.2±3.0 | 58.5±2.4 |
HVA | 8.5±4.4 | 32.3±4.4* |
IMA | 6.2±1.6 | 13.5±2.3* |
Table 2.
Temporal parameters with and without application of the BFO in the hallux valgus and control groups
Control group |
HV group |
||
---|---|---|---|
Without BFO | With BFO | ||
Cadence (steps/min) | 116.00±15.2 | 96.98±9.6a) | 101.33±9.3 |
Double support (%) | 19.80±8.0 | 30.45±5.1a) | 32.97±5.3 |
Foot off (%) | 60.10±7.3 | 64.46±2.3 | 65.89±2.1 |
Step length (m) | 0.63±0.1 | 0.53±0.1a) | 0.57±0.1 |
Step time (s) | 0.52±0.1 | 0.62±0.0 | 0.59±0.0 |
Step width (m) | 0.15±0.2 | 0.14±0.0 | 0.15±0.0 |
Stride length (m) | 1.27±0.1 | 1.04±0.1a) | 1.11±0.1 |
Stride time (s) | 1.04±0.1 | 0.25±0.1 | 1.18±0.1 |
Walking speed (m/s) | 1.23±0.2 | 0.83±0.1a) | 0.93±0.1 |
Table 3.
Motion at the intersegment angles showing differences between the control and HV groups
Intersegment angle | Gait phase | Control group |
HV group |
|
---|---|---|---|---|
Without BFO | With BFO | |||
Hallux-forefoot DF/PF (°) | Terminal stance | 4.22±13.38 | 6.23±14.13a) | -10.60±11.03b) |
Terminal swing | -3.44±9.61 | 3.91±14.78a) | -29.97±10.67b) | |
Forefoot-hindfoot DF/PF (°) | Mid-swing | -2.40±3.53 | 0.44±4.96a) | 2.81±3.85 |
Hindfoot-tibia Inv/Ev (°) | Mid-stance | 2.28±3.81 | -1.63±6.73a) | 3.25±5.63 |
Pre-swing | -1.25±2.48 | -3.63±6.73a) | -1.95±5.63b) |