Abstract
Background
Augmented renal clearance (ARC; creatinine clearance [CrCl] >130 mL/min/1.73 m2) is prevalent in patients with neurological injuries and may influence their exposure to important pharmacological therapies. Little is known about the relationship between estimated and measured CrCl in this population.
Methods
This single-center, prospective, observational cohort study aimed to describe the association between ARC and estimated CrCl and neurological outcomes in a broad neurocritical care population. Prospective patient screening criteria included adults aged 18–85 years, with critical illness due to neurologic causes (such as ischemic stroke or subarachnoid hemorrhage) and lack of renal dysfunction on admission. Patients who had at least one urine CrCl measurement performed within the first 7 days of hospitalization were included. Two cohorts were evaluated: those with ARC and those without ARC.
Results
Fifty-seven patients were included, of whom 49 (86%) exhibited ARC. Subjects with ARC were more likely to be male and had a significantly higher median measured CrCl (201.7 mL/min/1.73 m2) than those without ARC (109.8 mL/min/1.73 m2). The Augmented Renal Clearance in Trauma Intensive Care (ARCTIC) score displayed the strongest association (vs. CrCl equations) with ARC development (area under the receiver operating characteristic curve, 0.648).
Neurologically injured patients are at high risk of developing augmented renal clearance (ARC) due to hemodynamic alterations, aggressive fluid resuscitation, vasopressors usage, osmotherapy agents, and increased cardiac output from sympathetic nervous system responses to critical illness [1-5]. ARC is defined in critically ill patients as a measured creatinine clearance (CrCl) ≥130 mL/min/1.73 m2 [6]. Previous studies have noted ARC in as many as 85%–100% of patients with severe neurological injury [2,3]. This is notable when dosing patients on renally-eliminated medications, particularly those with unavailable routine serum concentration monitoring. Most medications have specific dosing recommendations for patients who exhibit reduced renal function, but recommendations for patients with so-called normal renal function (typically an estimated CrCl >60 mL/min/1.73 m2) do not address patients with ARC. Patients admitted with neurological critical illness have extensive pharmacotherapy needs pertaining to the management of infection, intracranial pressure, seizures, hemodynamics, and thromboembolic events, of which the expected pharmacokinetic/pharmacodynamic relationship is impacted by ARC. Thus, the presence of ARC may be more than a curious observation but a factor that may influence the response to important therapies and could substantially impact the optimization of pharmacotherapy [7,8].
Despite previous studies demonstrating a significant prevalence of ARC in critically ill patients, its diagnosis remains challenging due to difficulties in directly measuring renal function. If a direct assessment of CrCl is not available, serum creatinine-based CrCl estimation equations can assist in the diagnosis; however, these equations have performed poorly in identifying ARC [9,10]. Simple, bedside diagnostic tools have been developed, including the Augmented Renal Clearance in Trauma Intensive Care (ARCTIC) score, which considers easily retrievable patient factors like age and serum creatinine; however, the ARCTIC score has not been extensively validated in the neurocritical care population [11].
This study aimed to describe the association between measured CrCl values and commonly used CrCl estimation equations in neurocritical care patients with ARC [11-17]. In addition, this study evaluated the association between ARC and neurological outcomes at discharge. The primary study hypothesis was that commonly used CrCl estimation equations lack the ability to accurately predict actual CrCl in neurocritical care patients with ARC.
This single-center, prospective, observational cohort study was conducted at an 865-bed tertiary academic medical center, which is also a Joint Commission accredited Comprehensive Stroke Center and level 1 trauma center. The center is a regional hub for the care of patients with complex neurological illnesses. Adult patients (aged 18–85 years) admitted to the neuroscience intensive care unit with a diagnosis of subarachnoid hemorrhage (SAH), traumatic brain injury (TBI), acute ischemic stroke (AIS), and intracerebral hemorrhage (ICH), and had an indwelling urinary catheter in place at the time of screening were included. Study participants were excluded for incarceration, pregnancy, anticipated intensive care unit length of stay <72 hours, or serum creatinine >1.5 mg/dL at the time of screening. The study population included patients treated from May 2017 to April 2019. The management of SAH, TBI, AIS, and ICH was generally consistent with the published guidelines [18-21].
The study timeframe included the first 7 days of ICU stay for CrCl measurement and the time from admission to discharge for neurologic outcome. The unit protocol for 8-hour urine creatinine collection was performed to measure the CrCl level within the first few days of admission. Up to three 8-hour urine collections were performed during this 7-day period. The standard sampling procedure for an 8-hour urine creatinine collection included documentation of collection time, urine volume, and storage on ice throughout the duration of urine collection. The clinical laboratory measured urine volume and performed the urine and serum creatinine assays by colorimetric assay using the Jaffe method [22]. Pertinent clinical and demographic data were collected to describe study outcomes. The severity of illness measures varies based on the admission diagnosis. An etiology-specific severity measure was recorded for each patient: Hunt-Hess score (SAH), admission GCS score (TBI), National Institutes of Health (NIH) Stroke Scale (AIS), and ICH score [23-26]. While consecutive patients were screened for the need to measure urine CrCl, factors such as moribund state, long-standing or severe pre-existing disease that may affect renal function (such as diabetes mellitus), or other clinically relevant issues may have led investigators to forego CrCl measurement in specific patients. This resulted in the non-consecutive inclusion of patients in the overall cohort.
Cohorts for comparison were derived from patients with and without ARC, defined as at least one instance of a measured CrCl of ≥130 mL/min/1.73 m2 by an 8-hour urine creatinine collection. The measured CrCl was calculated based on the urine creatine clearance equation standardized to the body surface area (Fig. 1). The primary study outcome was the predictive ability and diagnostic accuracy of the estimation equations compared with the measured CrCl [11-17]. The sensitivity, specificity, positive predictive value (PPV), and negative predictive value (NPV) of each diagnostic test (ARCTIC score and the various estimated CrCl) in the whole cohort and according to subgroup (SAH, TBI, AIS, ICH) were calculated. The secondary outcomes were the overall prevalence of ARC in the study population and the neurological outcome at discharge (measured using the discharge modified Rankin Scale [mRS]). The mRS was derived from comments regarding patient functional status from the physical therapist and the provided notes at discharge. Poor outcome was defined as an mRS score of 4–6 (moderate disability to death), whereas an mRS of 1–3 was considered a good outcome.
Continuous variables were tested for distribution using histogram visualization and the Shapiro-Wilk test. Continuous variables with a normal distribution are presented as mean (standard deviation), and non-normally distributed continuous variables are presented as median (interquartile range [IQR]). These were analyzed using the Student t-test or Wilcoxon rank-sum test, respectively. Categorical variables were presented as frequencies and proportions and analyzed using the Pearson’s chi-square or Fisher’s exact test, as appropriate. The diagnostic test performance for ARC, comparing the measured CrCl (gold standard) to the ARCTIC score and serum creatinine-based CrCl equations, was performed using 2×2 tables with calculations of sensitivity, specificity, PPV, and NPV, and visualization of receiver operating characteristic (ROC) curves. ROC curves were constructed using multiple CrCl equations, and the areas were compared using the DeLong test. Percent agreement between the measured CrCl and ARCTIC score was assessed with the Κappa statistic [27]. Missing data were omitted from specific calculations and noted when appropriate. All statistical tests were performed using Stata/IC 14.2 (StataCorp., College Station, TX, USA).
A total of 57 patients were included, of which 49 of 57 (86%) exhibited ARC via measured CrCl on at least one study day. All patients had at least one 8-hour urine collection. The demographic data of the cohort are presented in Table 1. The mean age in those with ARC was 49.7 years (13.5) versus 56 years (13.74) in those without ARC, P=0.231. Patients with ARC were more likely to be male (51% vs. 12.5%, P=0.043). Overall, admission diagnoses included 63.2% SAH, 14% ICH, 14% TBI, and 8.8% AIS. No differences were identified in the primary neurological diagnoses between patients with and without ARC (P=0.981) (Table 1). Between the two cohorts, however, with the number of patients in the study, the balance based on the severity of illness is difficult to assess. Overall, patients with SAH had a median Hunt and Hess score of 3 (IQR, 2–3), patients with ICH had a median ICH score of 2 (IQR, 2–3), and patients with TBI had a median admission Glasgow Coma Scale score of 7 (IQR, 5–10). Patients with AIS had a median NIH Stroke score of 15 (IQR, 13–18). These metrics suggest high severity of illness across the spectrum of diagnoses.
Patients with ARC had a significantly higher median measured CrCl of 201.7 mL/min/1.73 m2 (IQR, 172.1–250.8) compared to those without ARC, 109.8 mL/min/1.73 m2 (IQR, 100.6–123.3) (P<0.0001). Overall, male patients exhibited a higher median measured CrCl compared to female patients, 213.5 mL/min/1.73 m2 (IQR, 158–273) versus 175.6 mL/min/1.73 m2 (IQR, 138.9–200.1), respectively (P=0.006). The creatinine-based estimation equations routinely underestimated the measured CrCl (Tables 1 and 2). For example, the CrCl estimated by Cockcroft-Gault underestimated the measured CrCl in each of the four disease states (Fig. 2). The diagnostic performances of the creatinine-clearance-based equations are presented in Table 2. Overall, each equation was performed with a high sensitivity and PPV (>80%) and a low specificity and NPV (<25%), with the ARCTIC score displaying the strongest association with measured CrCl. Using the standard pre-defined cutoff of ARCTIC score ≥6, 79.6% (45/49) of patients in the ARC group were at high risk for ARC, and 50% (4/8) of the non-ARC group were at high risk for ARC (P=0.071). The PPV and NPV for the ARCTIC score were 91% and 71%, respectively. The Κappa statistic for percent agreement between ARCTIC score and estimated CrCl was 0.31 (31% agreement), denoting a fair strength of agreement outside of chance between the two assessments. There was no difference among the areas under the ROC curve for all equations and the ARCTIC score (P=0.74) (Fig. 3).
There was no difference in neurologic outcome, as determined by the discharge mRS score (Table 1). The median mRS in the ARC group was 4 (IQR, 4–5) compared with that in those without ARC 4 (IQR, 4–6) (P=0.428). A poor outcome was observed in 47 of 49 (95.9%) subjects in the ARC group and 8 of 8 (100%) subjects in those without ARC (P=1.000).
The prevalence of ARC in our broad neurocritical care population was 86%, which is similar to previous reports in both general critical care populations and other specific neurocritical care cohorts, such as TBI, ICH, and SAH [2,3,5]. The severity of illness was high in the current study at baseline and the discharge mRS in this study was not different in patients with or without ARC. The high incidence of poor outcome is commensurate with the relatively high admission severity of illness for the current study population, as well as the screening criteria favoring patients with an anticipated prolonged ICU stay. Patients with neurological critical illness appear to be at a high risk for developing ARC early in the course of illness, as suggested by the high prevalence in the current study.
The gold standard for identifying ARC is the prospective collection of urine samples for creatinine analysis. Although feasible at most institutions, limitations exist, including inconvenience due to collection time, specimen processing and storage requirements, and reduced reliability in patients who do not have an in-dwelling catheter. Therefore, in most clinical settings, there is a reliance on serum creatinine-based CrCl equations to provide insights into a patient’s renal function. However, these equations consistently fail to fully characterize glomerular filtration in patients with ARC, as illustrated in the current study and others [2,10]. For example, the current study demonstrated an overall sensitivity of 79.6% and specificity of only 25% for the Cockcroft-Gault equation to predict the presence of ARC (the Cockcroft-Gault equation is the equation used in nearly all package insert dosing recommendations).
The ARCTIC score, validated to identify ARC in trauma patients, is a tool that uses readily available patient-specific data, which may be useful in the neurocritical care population [11]. The performance of ARCTIC in this neurocritical care population is comparable to the original findings by Barletta et al. [11], where the ARCTIC score demonstrated acceptable sensitivity (0.843), specificity (0.682), PPV (0.843), and NPV (0.682). In the current study, the ARCTIC score had a numerically higher area under the ROC than the Cockcroft-Gault, Modification of Diet in Renal Disease [MDRD], Jelliffe, Hull, CKD-EPI, and Davis-Chandler equations for predicting ARC occurrence; however, this difference was not statistically significant. The relative inaccuracy of the CrCl equations in the critical care population is a known limitation. The current analysis corroborates prior work that also assessed the discrepancies found in estimating CrCl using equations versus measuring the urine CrCl in ARC and high CrCl settings [10]. More research using the ARCTIC score is needed in a broader neurocritical care population to validate the usefulness of this predictor for the risk of ARC. A different approach that would likely require additional patients to be studied would be the development of an adjustment to the Cockcroft-Gault equation (or creation of a new equation) to estimate renal clearance more accurately in this population. A larger and more diverse dataset is necessary to accomplish this. Until a reliable approach is determined, however, institutions may opt to directly measure renal clearance via urine collection as the most accurate method in high-risk patients, despite the associated difficulties.
While ARC has been described consistently in the critical care population, it is not well known whether the severity of illness is a differentiating factor for exhibiting ARC or whether ARC influences outcomes. Many iatrogenic factors that contribute to the ARC, such as the need for fluid resuscitation, vasopressors, and osmotherapy agents, are more extensively used in patients with increasing severity of illness [28]. Therefore, it may be that the presence of ARC is a marker of the severity of illness and possibly a harbinger of poor outcomes. Hypermetabolic and catabolic states such as TBI, polytrauma, sepsis, and burns are all associated with ARC, perhaps as a physiological response to handling the solute load in a hypercatabolic state [29-33].
ARC may result in suboptimal exposure to many integral therapies used in neurocritical patents such as antiepileptic agents and antimicrobials (renally eliminated solutes) [8,34-36]. This may lead to complications such as breakthrough seizures and infection persistence or recurrence [8,34]. Evaluating outcomes in neurocritical care patients with more diversity in severity and etiology of illness may help to better define any relationship with ARC and outcome. Future studies should also evaluate outcomes specific to medication that might be affected by ARC (for example, renally eliminated medications such as levetiracetam, and the incidence of seizures.
As previously noted, the incidence of ARC in neurocritical care patients with TBI, SAH, and ICH has been considerably described in the literature [2,3,5]. However, ARC has not yet been reported in patients with AIS, which is notable in this study. The majority of patients with AIS in the current study had large middle cerebral artery strokes, with one patient presenting with venous sinus thrombosis and concomitant ICH. This represents a subset of ischemic stroke that may exhibit elevated intracranial pressure, a pronounced swelling period early after ictus, increased intensive care unit needs, and an increased risk of complications such as seizures. While aspects of critical care such as fluid resuscitation or use of vasopressors are usually not necessary after a large AIS, we hypothesize that the vigorous swelling pattern typical of middle cerebral artery stroke, the common use of permissive hypertension early in care, and the use of hypertonic solutions to prevent or treat hyponatremia and cerebral edema may have contributed to the presence of ARC in these patients. Although AIS patients comprise a small percentage of the total population, reporting this group’s susceptibility to ARC is valuable. However, further research in this population is necessary to better describe the physiological and iatrogenic factors that may promote ARC after AIS.
One important limitation of this study is that the sample size was too small to compare the outcomes in patients with and without ARC. The small sample size also limits the use of more sophisticated statistical tests. Continued evaluation of ARC and clinical outcomes is needed to better define any association between the two variables. In addition, not all patients underwent multiple urine CrCl measurements. Future studies using serial collections of the same patient would provide more insight into the clinical progression of ARC throughout a patient’s critical illness. Additionally, future studies that collect data on a wider breadth of neurological diagnoses, such as AIS or status epilepticus, would be valuable to expand these conclusions. The degree of illness in this study’s patient population was also particularly severe; therefore, further studies with a broader range of severity would be worthwhile. Finally, further pharmacokinetic studies in patients with ARC could precisely describe how the aforementioned renally cleared medications are affected throughout the course of a patient’s critical illness. Underexposure to vital therapies, such as agents for seizure prevention or treatment (for example, levetiracetam), or antimicrobials for hospital-acquired infections (for example, beta-lactams) can adversely affect patient responses [34-36].
In the present study, the prevalence of ARC in a broad neurocritical care population appeared to be high, at 86%. The ARCTIC score had improved sensitivity and specificity for diagnosing ARC when compared to common serum creatinine-based estimation equations, but did not exhibit a strong performance in predicting measured CrCl. If feasible, prospective urine collection and creatinine measurements remain accurate in directly detecting the presence of ARC. In this study, ARC was not associated with inferior outcomes compared with those who did not have ARC. Given the high likelihood of ARC in the neurocritical care population and the potential that it may affect common pharmacotherapy options, clinicians should consider prospective monitoring of ARC in at-risk patients.
Notes
REFERENCES
1. Cook AM, Arora S, Davis J, Pittman T. Augmented renal clearance of vancomycin and levetiracetam in a traumatic brain injury patient. Neurocrit Care. 2013; 19:210–4.


2. May CC, Arora S, Parli SE, Fraser JF, Bastin MT, Cook AM. Augmented renal clearance in patients with subarachnoid hemorrhage. Neurocrit Care. 2015; 23:374–9.


3. Udy A, Boots R, Senthuran S, Stuart J, Deans R, Lassig-Smith M, et al. Augmented creatinine clearance in traumatic brain injury. Anesth Analg. 2010; 111:1505–10.


4. Udy AA, Jarrett P, Lassig-Smith M, Stuart J, Starr T, Dunlop R, et al. Augmented renal clearance in traumatic brain injury: a single-center observational study of atrial natriuretic peptide, cardiac output, and creatinine clearance. J Neurotrauma. 2017; 34:137–44.


5. Morbitzer KA, Jordan JD, Dehne KA, Durr EA, Olm-Shipman CM, Rhoney DH. Enhanced renal clearance in patients with hemorrhagic stroke. Crit Care Med. 2019; 47:800–8.


6. Udy AA, Baptista JP, Lim NL, Joynt GM, Jarrett P, Wockner L, et al. Augmented renal clearance in the ICU: results of a multicenter observational study of renal function in critically ill patients with normal plasma creatinine concentrations. Crit Care Med. 2014; 42:520–7.
7. Udy AA, Roberts JA, De Waele JJ, Paterson DL, Lipman J. What’s behind the failure of emerging antibiotics in the critically ill?: understanding the impact of altered pharmacokinetics and augmented renal clearance. Int J Antimicrob Agents. 2012; 39:455–7.


8. Udy AA, Varghese JM, Altukroni M, Briscoe S, McWhinney BC, Ungerer JP, et al. Subtherapeutic initial β-lactam concentrations in select critically ill patients: association between augmented renal clearance and low trough drug concentrations. Chest. 2012; 142:30–9.


9. Grootaert V, Willems L, Debaveye Y, Meyfroidt G, Spriet I. Augmented renal clearance in the critically ill: how to assess kidney function. Ann Pharmacother. 2012; 46:952–9.


10. Baptista JP, Neves M, Rodrigues L, Teixeira L, Pinho J, Pimentel J. Accuracy of the estimation of glomerular filtration rate within a population of critically ill patients. J Nephrol. 2014; 27:403–10.


11. Barletta JF, Mangram AJ, Byrne M, Sucher JF, Hollingworth AK, Ali-Osman FR, et al. Identifying augmented renal clearance in trauma patients: Validation of the Augmented Renal Clearance in Trauma Intensive Care scoring system. J Trauma Acute Care Surg. 2017; 82:665–71.
12. Davis GA, Chandler MH. Comparison of creatinine clearance estimation methods in patients with trauma. Am J Health Syst Pharm. 1996; 53:1028–32.
13. Cockcroft DW, Gault MH. Prediction of creatinine clearance from serum creatinine. Nephron. 1976; 16:31–41.


14. Jelliffe RW. Letter: Creatinine clearance: bedside estimate. Ann Intern Med. 1973; 79:604–5.
15. Hull JH, Hak LJ, Koch GG, Wargin WA, Chi SL, Mattocks AM. Influence of range of renal function and liver disease on predictability of creatinine clearance. Clin Pharmacol Ther. 1981; 29:516–21.


16. Levey AS, Coresh J, Greene T, Stevens LA, Zhang YL, Hendriksen S, et al. Using standardized serum creatinine values in the modification of diet in renal disease study equation for estimating glomerular filtration rate. Ann Intern Med. 2006; 145:247–54.


17. Levey AS, Stevens LA, Schmid CH, Zhang YL, Castro AF 3rd, Feldman HI, et al. A new equation to estimate glomerular filtration rate. Ann Intern Med. 2009; 150:604–12.
18. Carney N, Totten AM, O’Reilly C, Ullman JS, Hawryluk GW, Bell MJ, et al. Guidelines for the management of severe traumatic brain injury, fourth edition. Neurosurgery. 2017; 80:6–15.


19. Connolly ES Jr, Rabinstein AA, Carhuapoma JR, Derdeyn CP, Dion J, Higashida RT, et al. Guidelines for the management of aneurysmal subarachnoid hemorrhage: a guideline for healthcare professionals from the American Heart Association/American Stroke Association. Stroke. 2012; 43:1711–37.


20. Hemphill JC 3rd, Greenberg SM, Anderson CS, Becker K, Bendok BR, Cushman M, et al. Guidelines for the management of spontaneous intracerebral hemorrhage: a guideline for healthcare professionals from the American Heart Association/American Stroke Association. Stroke. 2015; 46:2032–60.
21. Jauch EC, Saver JL, Adams HP Jr, Bruno A, Connors JJ, Demaerschalk BM, et al. Guidelines for the early management of patients with acute ischemic stroke: a guideline for healthcare professionals from the American Heart Association/American Stroke Association. Stroke. 2013; 44:870–947.
22. Clarke JT. Colorimetric determination and distribution of urinary creatinine and creatine. Clin Chem. 1961; 7:371–83.


23. Hunt WE, Hess RM. Surgical risk as related to time of intervention in the repair of intracranial aneurysms. J Neurosurg. 1968; 28:14–20.


25. Hemphill JC 3rd, Bonovich DC, Besmertis L, Manley GT, Johnston SC. The ICH score: a simple, reliable grading scale for intracerebral hemorrhage. Stroke. 2001; 32:891–7.
26. Goldstein LB, Bertels C, Davis JN. Interrater reliability of the NIH stroke scale. Arch Neurol. 1989; 46:660–2.
27. Landis JR, Koch GG. The measurement of observer agreement for categorical data. Biometrics. 1977; 33:159–74.


28. Udy AA, Roberts JA, Lipman J. Implications of augmented renal clearance in critically ill patients. Nat Rev Nephrol. 2011; 7:539–43.


29. Baptista JP, Sousa E, Martins PJ, Pimentel JM. Augmented renal clearance in septic patients and implications for vancomycin optimisation. Int J Antimicrob Agents. 2012; 39:420–3.
30. Udy AA, Roberts JA, Shorr AF, Boots RJ, Lipman J. Augmented renal clearance in septic and traumatized patients with normal plasma creatinine concentrations: identifying at-risk patients. Crit Care. 2013; 17:R35.


31. Udy AA, Jarrett P, Stuart J, Lassig-Smith M, Starr T, Dunlop R, et al. Determining the mechanisms underlying augmented renal drug clearance in the critically ill: use of exogenous marker compounds. Crit Care. 2014; 18:657.


32. Garrelts JC, Peterie JD. Altered vancomycin dose vs. serum concentration relationship in burn patients. Clin Pharmacol Ther. 1988; 44:9–13.


33. Lott RS, Uden DL, Wargin WA, Strate RG, Zaske DE. Correlation of predicted versus measured creatinine clearance values in burn patients. Am J Hosp Pharm. 1978; 35:717–20.


34. Carrie C, Bentejac M, Cottenceau V, Masson F, Petit L, Cochard JF, et al. Association between augmented renal clearance and clinical failure of antibiotic treatment in brain-injured patients with ventilator-acquired pneumonia: a preliminary study. Anaesth Crit Care Pain Med. 2018; 37:35–41.


35. Carrié C, Petit L, d'Houdain N, Sauvage N, Cottenceau V, Lafitte M, et al. Association between augmented renal clearance, antibiotic exposure and clinical outcome in critically ill septic patients receiving high doses of β-lactams administered by continuous infusion: a prospective observational study. Int J Antimicrob Agents. 2018; 51:443–9.
Fig. 1.
Creatinine clearance calculations. CrCl, creatinine clearance; SCr, serum creatinine (mg/dl); BSA, body surface area (m2).
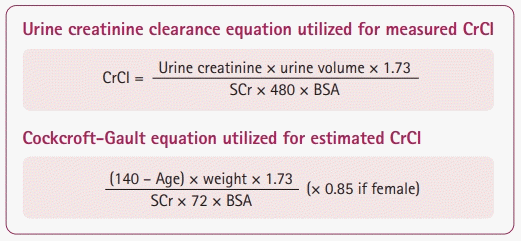
Fig. 2.
Estimated versus measured creatinine clearance (CrCl) by disease state. Comparison of creatinine clearance values (estimated and measured) by disease state. This graph depicts the creatinine clearance values of all patients with or without augmented renal clearance. ICH, intracerebral hemorrhage; SAH, subarachnoid hemorrhage; TBI, traumatic brain injury; AIS, acute ischemic stroke. P>0.05 for all comparisons between estimated and measured CrCl.

Fig. 3.
Receiver operating characteristic (ROC) curves of various creatinine clearance equations (≥130 mL/min/m2) and Augmented Renal Clearance in Trauma Intensive Care (ARCTIC) score (≥6) (P-value for comparison, 0.74). MDRD, Modification of Diet in Renal Disease; CKD-EPI, Chronic Kidney Disease Epidemiology Collaboration.

Table 1.
Baseline characteristics
Values are presented as mean±standard deviation, number (%), or median (interquartile range).
ARC, augmented renal clearance; ICH, intracerebral hemorrhage; SAH, subarachnoid hemorrhage; TBI, traumatic brain injury; AIS, acute ischemic stroke; GCS, Glasgow Coma Score; NIHSS, National Institutes of Health Stroke Score; AIS, acute ischemic stroke; CrCl, creatinine clearance; ARTIC, Augmented Renal Clearance in Trauma Intensive Care; mRS, modified Rankin Scale.