Abstract
Purpose
Methods
Results
References


























Fig. 1
Flow diagram of the study. RLN, recurrent laryngeal nerve; TT, total thyroidectomy; L, lobectomy; NAR, nerve at risk; IONM, intraoperative nerve monitoring; LOS, loss of signal; EMG, electromyography.
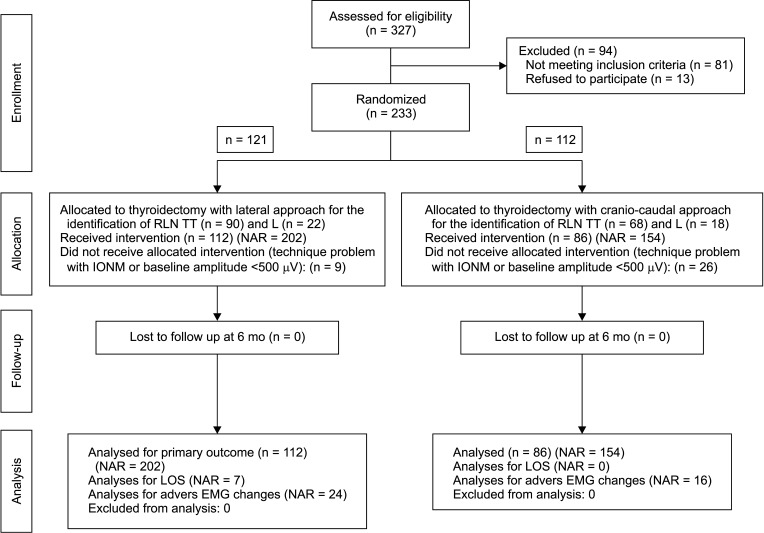
Fig. 2
(A) Visual identification of the recurrent laryngeal nerve (RLN) at the lower border of the cricopharyngeal muscle and the posterior aspect of the upper parathyroid gland in the craniocaudal approach. (B) It shows RLN entrapment at the ligament of Berry (LOB). The RLN is shown at the tip of the handheld probe. Nerve monitoring was very helpful to map the course of the nerve between the fibrous and fascial layers of the LOB.
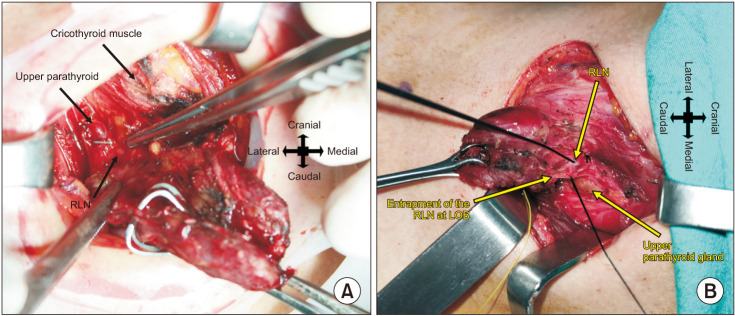
Fig. 3
Electromyographic (EMG) data showing repeated adverse EMG changes, combined event, and loss of signal. The blue line represents amplitude over time and the green line represents latency over time. In this patient, the recurrent laryngeal nerve had entrapment at the ligament of Berry and extralaryngeal branching, and the thyroid lobe volume was 40 cm3. EMG tracing shows a gradual decrease in the amplitude concordant with the increase in the latency period regarding the baseline values. The final amplitude was <100 µV. VN, vagal nerve.
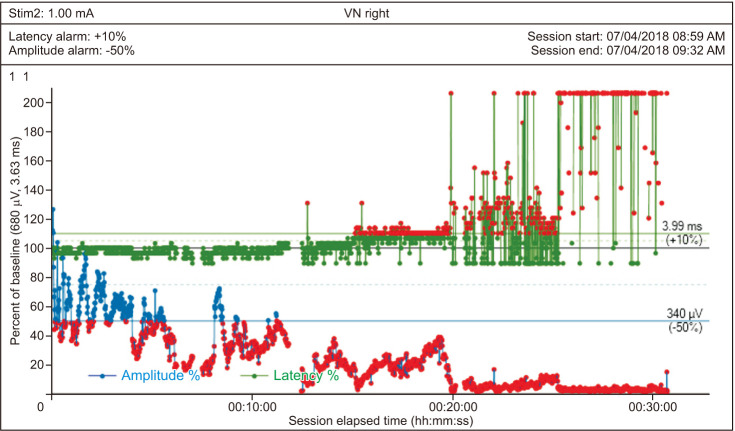
Fig. 4
It shows the possible mechanisms of recurrent laryngeal nerve (RLN) injury during the anteromedial traction of an enlarged thyroid lobe during the lateral approach to the RLN. In the RLNs with entrapment at the ligament of Berry (LOB), a stretch injury may develop by direct distress of the stretching ligament on the RLN (A) or by pulling down the distal part of the RLN which is already excessively stretched through the LOB during anteromedial retraction of the thyroid lobe (B). This patient is not one of the patients who are operated on during the study period but we preferred to use this photograph as it is descriptive for possible RLN injury during the unavoidable excessive anteromedial traction of an enlarged thyroid lobe in the lateral approach. No RLN injury occurred in this patient.
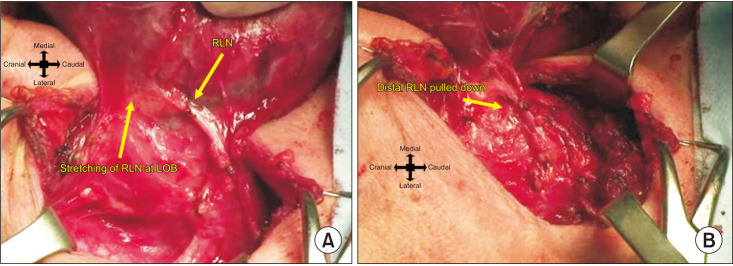
Table 2
Intraoperative and postoperative findings in group 1 and 2
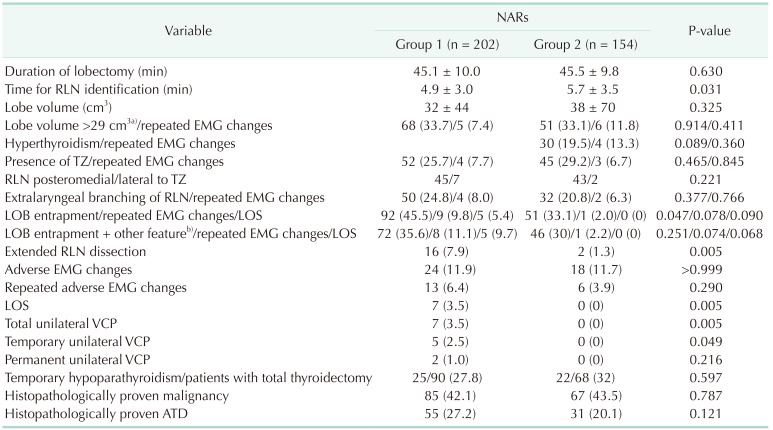
Values are presented as mean ± standard deviation, number (%), or number only.
Group 1, lateral approach; group 2, craniocaudal approach.
NAR, nerve at risk; RLN, recurrent laryngeal nerve; EMG, electromyography; TZ, tubercle of Zuckerkandl; LOB, ligament of Berry; LOS, loss of signal; VCP, vocal cord palsy; ATD, autoimmune thyroid disease.
a)The cutoff value of thyroid volume to predict repeated adverse EMG changes which was identified by receiver operating characteristic curve analysis. b)LOB entrapment accompanied by 1 or more of the following features: the TZ, extralaryngeal branching, ATD, hyperthyroidism, or lobe volume of >29 cm3.
Table 3
Presurgical dissection and postsurgical dissection amplitude and latency values in groups 1 and 2
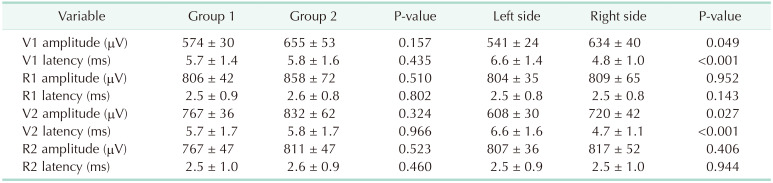
Table 4
Correlation between the adverse electromyography changes and clinicopathological and anatomical features
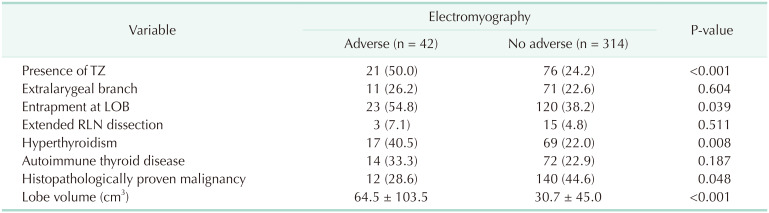
Table 5
Correlation between the repeated adverse electromyographic changes and clinicopathological and anatomical features and loss of signal

Values are presented as number (%), mean ± standard deviation, or median (range).
TZ, tubercle of Zuckerkandl; LOB, ligament of Berry; ATD, autoimmune thyroid disease.
a)LOB entrapment accompanied by 1 or more of the following features: the TZ, extralaryngeal branching, ATD, hyperthyroidism, or lobe volume of >29 cm3. b)The cutoff value of lobe volume to predict repeated adverse electromyographic changes which is identified by receiver operating characteristic curve analysis.
Table 6
Correlation between LOS and clinicopathological and anatomical features
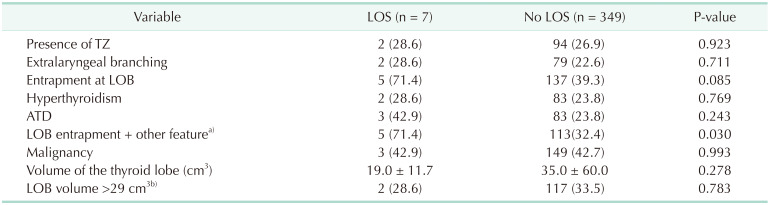
Values are presented as number (%) or mean ± standard deviation.
LOS, loss of signal; TZ, tubercle of Zuckerkandl; LOB, ligament of Berry; ATD, autoimmune thyroid disease.
a)LOB entrapment accompanied by 1 or more of the following features: the TZ, extralaryngeal branching, ATD, hyperthyroidism, or lobe volume of >29 cm3. b)The cutoff value of lobe volume to predict repeated adverse electromyographic changes by receiver operating characteristic analysis.