Abstract
Olfactory disorders one of the most frequent distinctive symptoms of COVID-19 infection. COVID-19-induced olfactory disorder can be classified as post-infectious olfactory dysfunction (PIOD). The effect of drugs on olfactory disorder following upper respiratory infection, including PIOD, has not been clearly established, which adds to the difficulty with treating the disorder. However, the effect of olfactory training on PIOD has been confirmed by numerous studies. As such, olfactory training is gaining attention, and has taken on greater importance, as the sole treatment for COVID-19–induced olfactory disorder in this pandemic age. This review describes the effect of olfactory training for COVID-19–induced olfactory disorder by analyzing the relevant literature.
Olfactory disorder is a commonly found upper respiratory condition; its prevalence stands at around 5% for the general population, and up to 24.5% in the population aged 50 years and over [1]. Olfactory disorder increases the risk of accidents as the patient cannot smell gas or food. It may also adversely affect the patient’s dietary and psychological life [2,3]. Despite these adverse effects on the patient’s quality of life, due to the lack of awareness on its seriousness, olfactory disorder is often ignored and left undiagnosed. The cause of olfactory disorder varies, from upper respiratory infection, paranasal sinus diseases, trauma, and congenital anomaly, each requiring different prognosis and treatment [4].
Coronavirus disease (COVID-19) is an acute respiratory disease caused by the severe acute respiratory syndrome coronavirus 2 (SARS-CoV-2). The disease rapidly spread across the world after the first confirmed case was reported in December 2019, and the World Health Organization declared a global pandemic in March 2020. The most common early symptoms of COVID-19 include fever, cough, throat pain, breathing difficulty, and headache [5]. However, some COVID-19 patients only experienced olfactory disorder, and up to 47.85% of COVID-19 patients were found to have olfactory disorder [6]. In response, the American Academy of Otolaryngology and Head and Neck Surgery proposed adding olfactory disorder to the list of distinguishing symptoms of COVID-19 [7]. In fact, a study found that people who confirmed positive in the reverse-transcription polymerase chain reaction test were 6.74 times more likely to experience olfactory disorder than the control group [8], and the degree of recent smell dysfunction in an individual with an upper respiratory symptom was reported as the most reliable COVID-19 predictor [9].
Many researchers proposed theories about the mechanism of olfactory disorder caused by COVID-19. However, none of them have been clearly substantiated. A histopathologic examination of a COVID-19 patient’s olfactory epithelium indicated an increase in proinflammatory cytokine, leukocyte infiltration, atrophic nasal mucosa, and marked destruction of olfactory epithelium [10-12]. It is unclear whether these symptoms were directly caused by the virus, or mediated by the damage to non-neuronal cells. The uncertainty about the cause of COVID-19–induced olfactory disorder makes the disease difficult to treat. However, it can be said with certainty that SARS-CoV-2 is one of the many viruses that may cause inflammation in the nasal cavity, and COVID-19–induced olfactory disorder can be classified as post-infectious olfactory dysfunction (PIOD) among many causes of olfactory disorder.
Olfactory training utilizes neuroplasticity to lower the olfactory threshold through repeated exposure to aromas. Hummel et al. (2009) [13] proposed an olfactory training method involving exposure to four aromas. Since then, many researchers confirmed the effectiveness of the method for patients and health individuals alike. Olfactory training is one of the most attractive treatment options for olfactory disorder, as it causes little side effect and can be performed by patients themselves at low costs. In addition, olfactory disorder serves as an evidence-based treatment option for PIOD, for which treatment has not been clearly identified [14,15]. It has taken on greater influence during the COVID-19 pandemic.
This review explores the relevant literature to outline the mechanism and effect of olfactory disorder, and describe the role and effect of olfactory training for COVID-19–induced olfactory disorder in this pandemic age.
Olfactory epithelium is plastic, which means the functions of damaged olfactory epithelium can be restored through neurogenesis. Before the 1980s, it was a widely held opinion that adults do not produce new nerve cells. However, multiple studies using adult rodents found nerve generation in the olfactory bulb [16,17]. Some researchers reported functional and structural changes in olfactory nerves caused by aromatic stimulus. They found that simple exposure to aroma causes changes in the activity of rodents’ olfactory mucosa, thereby lowering the threshold for the aromas that stimulated it [18,19]. In addition, the number of aroma-specific sensory nerve cells increased in olfactory-trained mice, as well as the size of the glomerulus in the olfactory bulb [20]. A 2004 study using human subjects reported that repeated exposure to androstenone lowered the androstenone threshold in both those who can detect odor and those who cannot [21]. The findings indicate the plasticity of the olfactory system, and serve as the evidentiary foundation of olfactory training currently performed on people with olfactory disorders.
Some studies confirmed changes in the olfactory nerves, while others used functional magnetic resonance imaging (fMRI) to verify the involvement of brain regions in the olfactory training mechanism. A study reported that continued exposure to a specific odor increases the reaction of the piriform cortex and the orbitofrontal cortex [22]. Another study compared the fMRI results of olfactory disorder patients before and after a twelve-week olfactory training program, which showed the restoration of functional connection in the non-olfactory areas of the brain, which were disorderly connected before the training [23]. In a study where healthy individuals went through olfactory training for only one of their nostrils, the participants showed significant volume increase in both olfactory bulbs [24]. These findings led some researchers infer a top-down process from the brain to olfactory nerves. These and other researchers reported that various stages of the olfactory training system are involved in olfactory training, and it is difficult to explain the changes brought on by olfactory training with a single theory about its mechanism. A large number of issues still remain to be explained through lab studies.
Many studies reported the positive effect of olfactory training on COVID-19–induced olfactory disorder. In order to qualitatively and quantitatively analyze the degree of the effect and identify clinical evidence of the effect, this study searched for academic articles on the effect of olfactory training for meta-analysis.
This study reviewed all English literature published at PubMed, Scopus, Embase, Web of Science, Google Scholar, and the Cochrane database in or before December 2021. The search words used included COVID-19, SARS-CoV-2, anosmia, hyposmia, olfactory, smell, olfactory disorders, olfactory dysfunctions, recovery, olfactory test, olfactory training, and treatment. Two researchers independently reviewed the titles and abstracts of all literature identified through the search, and excluded those not related to olfactory training for COVID-19 patients. For articles of which exclusion could not be determined based on abstracts, the researchers checked to the full text of the literature to decide whether to exclude them. The researchers also excluded the studies on patients who had had long-term olfactory disorder before COVID-19 or had chronic olfactory disorder after COVID-19 (30 days or longer), shortlisting three studies (Fig. 1).
The researchers extracted the following information from the selected literature and organized them in a standardized format: number of patients, olfactory score, prevalence or percentage of olfactory disorder after COVID-19 diagnosis, and p-values representing inter-group differences before and after olfactory training [25]. The change between olfactory scores before and after olfactory disorder and the percentage of olfactory disorder were selected as the assessment variables [26-28]. The risk of bias analysis used Cochrane’s risk of bias tool [29].
The effect sizes of the shortlisted studies were analyzed using the R software (R Foundation for Statistical Computing, Vienna, Austria). The effect sizes of continuous olfactory score variables such as self-reported olfactory scores, visual analogue scale and sniff stick test scores were analyzed using standard mean difference (SMD). Categorical variables were analyzed using odds ratio (OR). In addition, a sensitivity test was performed to analyze the effect of each study on the overall findings of the meta-analysis.
One-hundred nine patients from the three studies were included in the analysis. The characteristics and bias assessment of each study are represented in Table 1. As the number of studies was not high enough for a funnel plot or regression-based methods, this study did not assess the publication bias. In addition, in order to analyze their sensitivity, this assessed changes in the total estimates by repeating meta-analysis with a different study excluded in each session. The findings are summarized below.
Compared with pre-training, the olfactory training significantly increased the olfactory scores (SMD=1.7779, 95% confidence interval [1.0077; 2.5481], p<0.001, I2=87.9%) (Fig. 2) and significantly lowered the percentage of olfactory disorder (OR=0.0115 [0.0022; 0.0598], p<0.001, I2=0.0%) (Fig. 3). However, heterogeneity among the studies was confirmed in the analysis of olfactory scores before and after the olfactory training (I2>50%). Regarding the heterogeneity, Kasiri et al. [28] was found to show different pre and post-training olfactory scores than the other studies. The SMD from Kasiri et al. [28] was markedly lower than the combined SMD of the three studies (0.99 vs. 1.78). When Kasiri et al. [28] was excluded from the meta-analysis, the I2 dropped below 50%, which suggests that Kasiri et al. is the cause of the heterogeneity. However, the SME from Kasiri et al. [28] indicated significant effect of olfactory training. The findings suggest that, despite partial heterogeneity, olfactory training can improve olfactory disorder.
For a meta-analysis of the effect of olfactory training, the effect of the treatment may be accurately analyzed by comparing a treatment group (who went through olfactory training) and a control group (who did not go through olfactory training). However, according to the latest trends, most patients with olfactory dysfunction go through olfactory training, which prevents a comparison with a control group. The three analyzed studies were not designed to analyze the effect of olfactory training, although a treatment group who received nasal spray and olfactory training was compared with a control group who only received olfactory training in order to analyze the effect of nasal spray on olfactory dysfunction. As such, in order to single out the effect of olfactory training, this study meta-analyzed the findings for the olfactory training-only group before and after the treatment.
Seventy percent of patients with post–COVID-19 olfactory dysfunction were found to restore their olfactory functions without treatment. As such, it appears that the method used in this study may be affected by natural course and other factors. In other words, without a control group, improvement in the olfactory function in olfactory training-only group does not serve as sufficient evidence to conclude that olfactory training improved the olfactory function of patients with post-COVID-19 olfactory dysfunction.
The global COVID-19 pandemic raised public interest in PIOD, olfactory dysfunction, and olfactory training. The classic olfactory training designed by Hummel requires at least twelve weeks to complete. Each patient is exposed to a set of four aromas twice per day. The four aromas are: phenyl ethyl alcohol (rose), eucalyptol (eucalyptus), citronellal (lemon), and eugenol (cloves) [13]. A patient receives four brown vials (50 mL in total), and each vial emits one of the four aromas. The vial contains a cotton ball soaked with 1 mL of the aroma-emitting essence to prevent the liquid from flowing, and each vial is labeled with the name of the aroma. Each day, a patient smells each aroma for around ten seconds once in the morning and again in the evening, repeating the process until the patient smells all four aromas. In order to focus on the training, patients were required to assess and record their overall olfactory function on Sundays. In Hummel et al.’s study [13], the patients’ olfactory function was assessed using the Sniffin’ Sticks test kit before and after the twelve-week training.
Medications currently prescribed for PIOD patients include local and systemic glucocorticoid, alpha lipoic acid, and caroverin [30,31]. The effect of nasal steroid spray on the olfactory function of COVID-19 patients can be explained through a large-scale randomized controlled trial [26,32]. Kasiri et al. [28] suggested that, compared with the olfactory training-only option, the combination of olfactory training and the mometasone furoate nasal spray is more effective in improving the olfactory function of COVID-19 patients with anosmia. On the other hand, Abdelrahman Ahmed analyzed 50 patients complaining of post–COVID-19 olfactory dysfunction by dividing them into the training-only group and the mometasone furoate nasal spray group, and failed to find significant difference in olfactory function in the third week. As such, Abdelalim et al. [26] reported that the nasal steroid spray does not hold advantage over olfactory training in the treatment of post–COVID-19 olfactory disorder. The same conclusion was reported by Rashid et al. [32], who conducted a placebo-controlled trial with 356 COVID-19 and olfactory disorder patients. The patients were divided into two groups: a group of 138 patients who applied betamethasone nasal spray, and another group of 138 patients who applied saline nasal spray. The study failed to identify significant difference between the two groups after 30 days.
Vaira et al. [12] and Le Bon et al. [33] recently conducted small-scale studies that analyzed the clinical benefits of adding oral steroid and nasal steroid spray to olfactory training for COVID-19 patients with olfactory disorder. In Vaira et al.’s study [12], 9 patients who experienced olfactory disorder for 30 days or longer used oral and nasal steroid during their olfactory training, while the control group only went through olfactory training. The findings showed that the patients treated with nasal and oral corticosteroid reported significantly improved olfactory scores than the control group on Day 20 and 40 [12]. Le Bon et al. [33] treated 9 COVID-19 patients with one month or longer olfactory dysfunction with oral steroid and olfactory training, while the control group (18 patients with olfactory disorder for an average 5 weeks) only received olfactory training. The findings indicated a higher level of olfactory score improvement in the group treated with oral steroid. However, while both Vaira et al. [12] and Le Bon et al. [33] found significant difference in identification scores after a month of treatment, the difference was no longer significant after two months. The findings suggest that glucocorticoid accelerates the recovery of olfactory function by accelerating the elevation to the normal threshold and reducing the occurrence of parosmia. However, the group treated with two-month training and systemic steroid or the group with training and nasal steroid did not significantly differ from the training-only group in terms of the percentage of patients who recovered normal olfactory function. This finding requires long-term tracking. The fast recovery reported by the oral steroid group appears to be related with the effect of corticosteroid to accelerate epithelium regeneration and reduce inflammation on the olfactory mucosa. The use of oral corticosteroid for post–COVID-19 olfactory disorder has sparked controversies over the possibility of exacerbation caused by immunosuppression, delayed elimination of the virus, and other side effects. Patients using oral steroids reported adverse side effects such as insomnia, headache, and high blood pressure, of which prevalence rates were similar to those reported by other researchers. The use of oral corticosteroid in COVID-19–related olfactory disorder patients requires further studies [27,34].
Despite some discrepancies among the studies included in the analysis, a number of recent meta-analysis studies have shown the benefits of olfactory training for patients with olfactory disorders from various causes [35,36]. In addition, numerous studies found that olfactory training is more effective for disorder caused by PIOD than olfactory disorders from other causes [15,37-41]. In a systemic review, Harless and Liang [30] failed to find any evidence for the use of drug as the sole treatment for olfactory disorder, which highlights the use of non-drug options such as olfactory training. In a meta-analysis, minimal clinically important difference in threshold discrimination identification (TDI) scores of PIOD patients treated with olfactory training was almost three times higher than that of control group [42]. According to the study, PIOD patients showed reduced metabolism in the olfactory center of their brains, and olfactory training induces changes in the functional connection in olfactory senses, somatic senses, and the integrated pathways [43,44]. The effectiveness of drug treatment has not been proven to an extent that justifies it as a treatment option for PIOD, which supports the increased interest in olfactory training as a treatment option.
COVID-19–induced olfactory disorder can be classified as PIOD, and olfactory disorder is known to improve the olfactory function of COVID-19 patients. The relevant studies need optimization and standardization to account for slight differences in their training protocols. Given the findings on the improvement of olfactory function in patients treated with systemic and/or nasal steroid relative to the training-only group, the benefits of steroid for patients with COVID-19–related olfactory disorder have not been substantiated, and requires further studies.
Olfactory training must be considered as a treatment option for olfactory dysfunction in PIOD and COVID-19 patients. The training should be preceded by otorhinolaryngologic examination to identify other causes, and accompany an appropriate olfactory test to determine the exact degree of olfactory dysfunction and assess the effect of olfactory training.
Notes
Availability of Data and Material
All data generated or analyzed during the study are included in this published article.
Author Contributions
Conceptualization: Conceptualization: Jin Kook Kim, Byung Guk Kim. Formal analysis: Se Hwan Hwang. Investigation: Se Hwan Hwang, Ji-Sun Kim. Methodology: all authors. Project administration: Jin Kook Kim, Byung Guk Kim. Supervision: Jin Kook Kim, Byung Guk Kim. Validation: all authors. Visualization: Se Hwan Hwang. Writing—original draft: Se Hwan Hwang, Ji- Sun Kim, Bo Yoon Choi. Writing—review & editing: all authors.
ACKNOWLEDGMENTS
Prepared by the Olfactory and Gustatory Research Society with support from the Korean Rhinologic Society.
References
1. Brämerson A, Johansson L, Ek L, Nordin S, Bende M. Prevalence of olfactory dysfunction: the Skövde population-based study. Laryngoscope. 2004; 114(4):733–7.


2. Croy I, Nordin S, Hummel T. Olfactory disorders and quality of life--an updated review. Chem Senses. 2014; 39(3):185–94.


4. Temmel AF, Quint C, Schickinger-Fischer B, Klimek L, Stoller E, Hummel T. Characteristics of olfactory disorders in relation to major causes of olfactory loss. Arch Otolaryngol Head Neck Surg. 2002; 128(6):635–41.


5. Wiersinga WJ, Rhodes A, Cheng AC, Peacock SJ, Prescott HC. Pathophysiology, transmission, diagnosis, and treatment of coronavirus disease 2019 (COVID-19): a review. JAMA. 2020; 324(8):782–93.


6. Saniasiaya J, Islam MA, Abdullah B. Prevalence of olfactory dysfunction in coronavirus disease 2019 (COVID‐19): a meta‐analysis of 27,492 patients. Laryngoscope. 2021; 131(4):865–78.
7. Kowalski LP, Sanabria A, Ridge JA, Ng WT, de Bree R, Rinaldo A, et al. COVID-19 pandemic: effects and evidence-based recommendations for otolaryngology and head and neck surgery practice. Head Neck. 2020; 42(6):1259–67.
8. Menni C, Valdes AM, Freidin MB, Sudre CH, Nguyen LH, Drew DA, et al. Real-time tracking of self-reported symptoms to predict potential COVID-19. Nat Med. 2020; 26(7):1037–40.


9. Gerkin RC, Ohla K, Veldhuizen MG, Joseph PV, Kelly CE, Bakke AJ, et al. Recent smell loss is the best predictor of COVID-19 among individuals with recent respiratory symptoms. Chem Senses. 2021; 46:bjaa081.
10. Kirschenbaum D, Imbach LL, Ulrich S, Rushing EJ, Keller E, Reimann RR, et al. Inflammatory olfactory neuropathy in two patients with COVID-19. Lancet. 2020; 396(10245):166.


11. Torabi A, Mohammadbagheri E, Akbari Dilmaghani N, Bayat AH, Fathi M, Vakili K, et al. Proinflammatory cytokines in the olfactory mucosa result in COVID-19 induced anosmia. ACS Chem Neurosci. 2020; 11(13):1909–13.


12. Vaira LA, Hopkins C, Petrocelli M, Lechien JR, Cutrupi S, Salzano G, et al. Efficacy of corticosteroid therapy in the treatment of long- lasting olfactory disorders in COVID-19 patients. Rhinology. 2021; 59(1):21–5.


13. Hummel T, Rissom K, Reden J, Hähner A, Weidenbecher M, Hüttenbrink KB. Effects of olfactory training in patients with olfactory loss. Laryngoscope. 2009; 119(3):496–9.


14. Konstantinidis I, Tsakiropoulou E, Constantinidis J. Long term effects of olfactory training in patients with post-infectious olfactory loss. Rhinology. 2016; 54(2):170–5.


15. Damm M, Pikart LK, Reimann H, Burkert S, Göktas Ö, Haxel B, et al. Olfactory training is helpful in postinfectious olfactory loss: a randomized, controlled, multicenter study. Laryngoscope. 2014; 124(4):826–31.


16. Luskin MB. Restricted proliferation and migration of postnatally generated neurons derived from the forebrain subventricular zone. Neuron. 1993; 11(1):173–89.


17. Lois C, Alvarez-Buylla A. Long-distance neuronal migration in the adult mammalian brain. Science. 1994; 264(5162):1145–8.


18. Youngentob SL, Kent PF. Enhancement of odorant-induced mucosal activity patterns in rats trained on an odorant identification task. Brain Res. 1995; 670(1):82–8.


19. Wang HW, Wysocki CJ, Gold GH. Induction of olfactory receptor sensitivity in mice. Science. 1993; 260(5110):998–1000.


20. Jones SV, Choi DC, Davis M, Ressler KJ. Learning-dependent structural plasticity in the adult olfactory pathway. J Neurosci. 2008; 28(49):13106–11.


21. Wang L, Chen L, Jacob T. Evidence for peripheral plasticity in human odour response. J Physiol. 2004; 554(Pt 1):236–44.


22. Li W, Luxenberg E, Parrish T, Gottfried JA. Learning to smell the roses: experience-dependent neural plasticity in human piriform and orbitofrontal cortices. Neuron. 2006; 52(6):1097–108.


23. Kollndorfer K, Kowalczyk K, Hoche E, Mueller CA, Pollak M, Trattnig S, et al. Recovery of olfactory function induces neuroplasticity effects in patients with smell loss. Neural Plast. 2014; 2014:140419.


24. Negoias S, Pietsch K, Hummel T. Changes in olfactory bulb volume following lateralized olfactory training. Brain Imaging Behav. 2017; 11(4):998–1005.


25. Kim DH, Kim SW, Stybayeva G, Lim SY, Hwang SH. Predictive value of olfactory and taste symptoms in the diagnosis of COVID-19: a systematic review and meta-analysis. Clin Exp Otorhinolaryngol. 2021; 14(3):312–20.


26. Abdelalim AA, Mohamady AA, Elsayed RA, Elawady MA, Ghallab AF. Corticosteroid nasal spray for recovery of smell sensation in COVID-19 patients: a randomized controlled trial. Am J Otolaryngol. 2021; 42(2):102884.


27. Saussez S, Vaira LA, Chiesa-Estomba CM, Bon SL, Horoi M, Deiana G, et al. Short-term efficacy and safety of oral and nasal corticosteroids in COVID-19 patients with olfactory dysfunction: a European multicenter study. Pathogens. 2021; 10(6):698.


28. Kasiri H, Rouhani N, Salehifar E, Ghazaeian M, Fallah S. Mometasone furoate nasal spray in the treatment of patients with COVID-19 olfactory dysfunction: a randomized, double blind clinical trial. Int Immunopharmacol. 2021; 98:107871.


29. Higgins JP, Altman DG, Gøtzsche PC, Jüni P, Moher D, Oxman AD, et al. The Cochrane Collaboration's tool for assessing risk of bias in randomised trials. BMJ. 2011; 343:d5928.


30. Harless L, Liang J. Pharmacologic treatment for postviral olfactory dysfunction: a systematic review. Int Forum Allergy Rhinol. 2016; 6(7):760–7.


31. Seo BS, Lee HJ, Mo JH, Lee CH, Rhee CS, Kim JW. Treatment of postviral olfactory loss with glucocorticoids, Ginkgo biloba, and mometasone nasal spray. Arch Otolaryngol Head Neck Surg. 2009; 135(10):1000–4.


32. Rashid RA, Zgair A, Al-Ani RM. Effect of nasal corticosteroid in the treatment of anosmia due to COVID-19: a randomised double-blind placebo-controlled study. Am J Otolaryngol. 2021; 42(5):103033.


33. Le Bon SD, Konopnicki D, Pisarski N, Prunier L, Lechien JR, Horoi M. Efficacy and safety of oral corticosteroids and olfactory training in the management of COVID-19-related loss of smell. Eur Arch Otorhinolaryngol. 2021; 278(8):3113–7.


34. Mazzatenta A, Neri G, D'Ardes D, De Luca C, Marinari S, Porreca E, et al. Smell and taste in severe CoViD-19: self-reported vs. testing. Front Med (Lausanne). 2020; 7:589409.


35. Sorokowska A, Drechsler E, Karwowski M, Hummel T. Effects of olfactory training: a meta-analysis. Rhinology. 2017; 55(1):17–26.


36. Pekala K, Chandra RK, Turner JH. Efficacy of olfactory training in patients with olfactory loss: a systematic review and meta-analysis. Int Forum Allergy Rhinol. 2016; 6(3):299–307.


37. Qiao XF, Wang GP, Li X, Bai YH, Zheng W. Analysis of the clinical effect of olfactory training on olfactory dysfunction after upper respiratory tract infection. Acta Otolaryngol. 2019; 139(7):643–6.


38. Oleszkiewicz A, Hanf S, Whitcroft KL, Haehner A, Hummel T. Examination of olfactory training effectiveness in relation to its complexity and the cause of olfactory loss. Laryngoscope. 2018; 128(7):1518–22.


39. Patel ZM. The evidence for olfactory training in treating patients with olfactory loss. Curr Opin Otolaryngol Head Neck Surg. 2017; 25(1):43–6.


40. Altundag A, Cayonu M, Kayabasoglu G, Salihoglu M, Tekeli H, Saglam O, et al. Modified olfactory training in patients with postinfectious olfactory loss. Laryngoscope. 2015; 125(8):1763–6.


41. Fleiner F, Lau L, Göktas Ö. Active olfactory training for the treatment of smelling disorders. Ear Nose Throat J. 2012; 91(5):198–215.


42. Kattar N, Do TM, Unis GD, Migneron MR, Thomas AJ, McCoul ED. Olfactory training for postviral olfactory dysfunction: systematic review and meta-analysis. Otolaryngol Head Neck Surg. 2021; 164(2):244–54.


Fig. 2.
Post-treatment (olfactory training) versus pre-treatment with SMD. SMD of olfactory score (total: number of participants per group). SD, standard deviation; SMD, standard mean difference; CI, confidence interval.
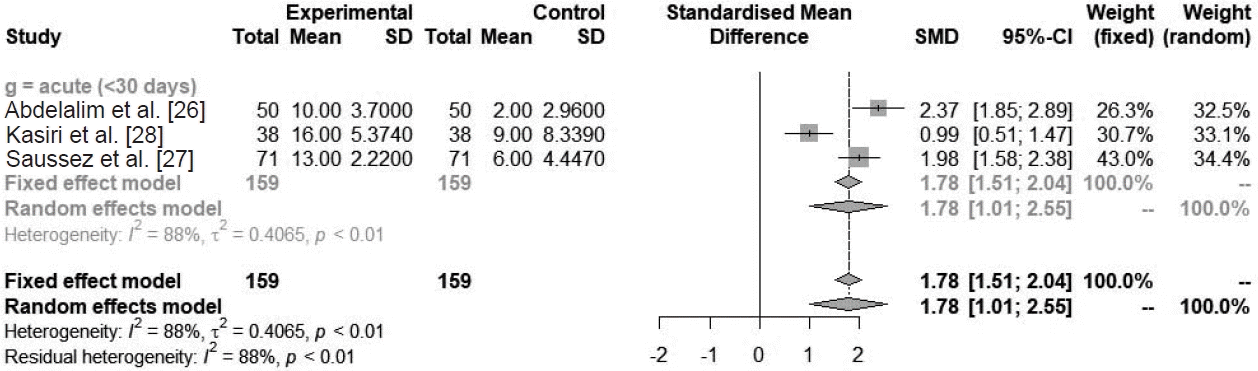
Fig. 3.
Post-treatment (olfactory training) versus pre-treatment with OR. Percentage of olfactory dysfunction (total: number of participants per group). OR, odds ratio; CI, confidence interval.
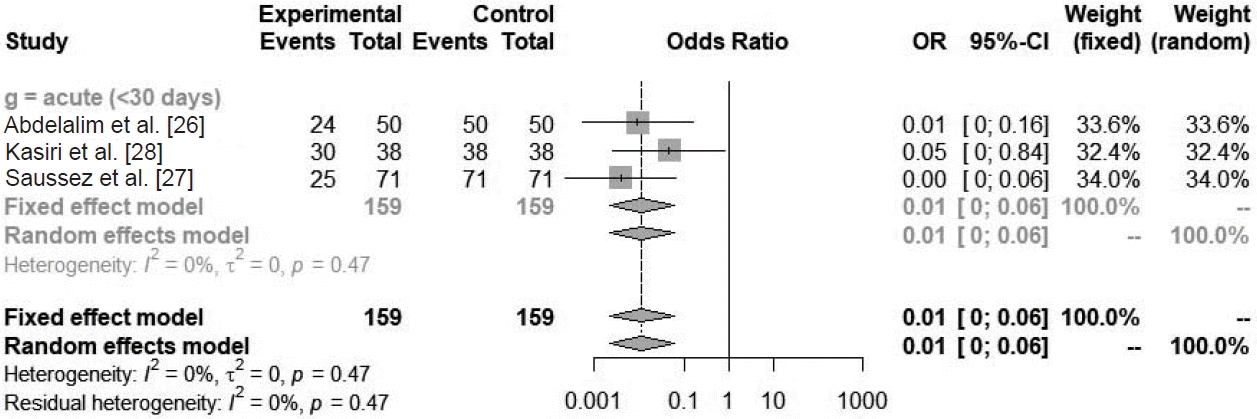
Table 1.
Summary of included studies and risk of bias assessment
Study (year) | Sample size | Age (yr)/sex (M:F) | Study design | Duration of olfactory dysfunction | Outcome measure analyzed |
---|---|---|---|---|---|
Kasiri et al. [28] 2021 | 38 | 33.2±8.5/19:19 | Prospective clinical trial | Severe microsmia or anosmia within two weeks | Olfactory score (UPSIT) |
Rate of olfactory dysfunction | |||||
Abdelalim et al. [26] 2021 | 50 | 30.0 (IQR 22.5–39.0)/22:28 | Prospective, randomized, controlled trial | Olfactory disorder with onset of less than 2 weeks | Olfactory score (self- reporting score) |
Rate of olfactory dysfunction | |||||
Saussez et al. [27] 2021 | 71 | 43.5±14.1/31:40 | Prospective cohort | Olfactory disorder with onset of less than 2weeks | Olfactory score (Sniffin’ Sticks tests) |
Rate of olfactory dysfunction |