Abstract
Objective
This study evaluated the effect of cyclic pre-calcification treatment on the improvement of bioactivity and osseointegration of Ti-6Al-4V mini-screws.
Methods
The experimental groups were an untreated group (UT), an anodized and heat-treated group (AH), and an anodized treatment followed by cyclic pre-calcification treatment group (ASPH). A bioactive material with calcium phosphate was coated on the mini-screws, and its effects on bioactivity and osseointegration were evaluated in in vitro and in vivo tests of following implantation in the rat tibia.
Results
As a result of immersing the ASPH group in simulated body fluid for 2 days, protrusions appearing in the initial stage of hydroxyapatite precipitation were observed. On the 3rd day, the protrusions became denser, other protrusions overlapped and grew on it, and the calcium and phosphorus concentrations increased. The removal torque values increased significantly in the following order UT group (2.08 ± 0.67 N·cm), AH group (4.10 ± 0.72 N·cm), and ASPH group (6.58 ± 0.66 N·cm) with the ASPH group showing the highest value (p < 0.05). In the ASPH group, new bone was observed that was connected to the threads, and it was confirmed that a bony bridge connected to the adjacent bone was formed.
The use of mini-screws for orthodontic treatment allows the operator to simply proceed with the implantation process and apply orthodontic force immediately after implantation. In addition, since the use of an extraoral device can be excluded, this does not depend on the patient’s cooperation.1,2 It is easy to adjust the strength of the orthodontic anchorage and the direction of traction depending on the situation, and various uses are possible through the combination of teeth intrusion, extrusion, protraction, retraction, maintenance, and intraoral devices.3 From the patient’s point of view, mini-screws are non-invasive compared to dental implants, have less discomfort than an extraoral device, and do not interfere with life because they are invisible.4,5 However, orthodontic mini-screws have low stability because they acquire retentiveness through mechanical bonding.
When titanium orthodontic mini-screws are implanted, about 10% of them fail, with most failures occurring within 1–2 months after implantation, and 90% of failures occurring within 4 months.6 To improve these problems and increase the clinical success rate, coating of the titanium orthodontic mini-screws with bioactive ceramic material or hydroxyapatite, a component of bone, has been used to impart surface activity.1,7-9 However, these methods induce osseointegration at the initial stage of implantation, and it is difficult to obtain bond strength between the coating layer and the underlying titanium. Reports have demonstrated that the coating layer that forms thickly on titanium is weak and can be easily peeled off from the surface.10,11
For this reason, several studies have investigated the effect of hydroxyapatite coating on titanium mini-screws and surface treatment methods in which the coating layer does not easily peel off. A surface treatment method of forming nanotube-structured TiO2 layers on the titanium mini-screws and then applying a nano-thick bioactive coating layer by synthetic hydroxyapatite deposition has been introduced.12,13
This study investigated the use of a bioactive surface on the Ti-6Al-4V alloy orthodontic mini-screws in terms of its ability to obtain retention through mechanical interlocking and enhancement of osseointegration in the short-term. In vitro and in vivo experiments were conducted to evaluate the effect of cyclic pre-calcification on bioactivity and osseointegration.
In this study, a Ti-6Al-4V extra low interstitials (ELI) alloy plate with a thickness of 100 μm was cut into 10 mm × 10 mm specimens and used for cytotoxicity testing and X-ray diffraction analysis. For the orthodontic mini-screws used in the in vivo test, a 4-mm Ti-6Al-4V ELI alloy rod (Fort Wayne Metals Research Products Co., FortWayne, IN, USA) was computer numerical control (CNC) machined (Cincom L20; Citizen Machinery Co., Ltd., Miyota, Japan), and mini-screws with a thread diameter of 1.4 mm × length of 3.3 mm were manufactured by a specialized company (Doiff Co., Suncheon, Korea).
To create a nanotube structured TiO2 layer on the surface of the specimens, a platinum plate and a specimen were connected to the anode and cathode of a DC power supply (Inverter Tech Co., Ltd., Gwangju, Korea), respectively. Anodization was performed for 1 hour at a voltage of 20 V and a current density of 20 mA/cm2, and aqueous electrolyte solution was used by including 1 wt.% NH4F, 20 wt.% H2O, and 79 wt.% glycerol.
Anodized specimens were immersed in 0.5 vol% silica aqueous solution for 5 minutes and dried at 100°C for 1 hour. After that, the specimens were alternately immersed in 0.05 M KH2PO4 aqueous solution and 0.01 M Ca(OH)2 aqueous solution at 90°C at 1 min/cycle, and cyclic pre-calcification was performed 20 times. Then, the specimens were raised to 500°C at a heating rate of 10°C/min for 2 hours in an electric furnace for removal of impurities and structural stabilization of the nanotube TiO2 layer and the Ca-P layer. The three types of groups are summarized in Table 1.
The crystal phase of the surface-treated mini-screws was analyzed in the 2θ range, from 10° to 90° using an X-ray diffractometer (XRD; X’PERT-PRO Powder; PANalytical Co., Almelo, The Netherlands). The morphological microstructure of the surface-treated specimens that were immersed in simulated body fluid (SBF) for 1, 2, and 3 days were analyzed with a field emission scanning electron microscope (FE-SEM; SUPRA40VP; Carl Zeiss Co., Oberkochen, Germany). The change in the concentration of the surface layer element immersed in SBF for 1, 2, and 3 days was analyzed by energy-dispersive X-ray spectroscopy.
All specimens were autoclaved at 120°C for 20 minutes, immersed in 10 mL of SBF adjusted to a pH 7.4, and maintained at 37°C and 5% CO2 incubator for 1, 2, and 3 days, respectively.
The cell culture medium was prepared by adding antibiotics 500 unit/mL (Gibco Co., Waltham, MA, USA) and fetal bovine serum to alpha minimum essential medium, and mouse-derived osteoblast cells (MC3T3-E1; American Type Culture Collection, Manassas, VA, USA) were used. All specimens were used after sterilization with ethylene oxide (EO) gas, and the cells were cultured at a temperature of 37°C and 5% CO2 incubator.
The cytotoxicity test was performed on the extraction medium of the specimens by water-soluble tetrazolium salt (WST)-8 assay (Enzo cell counting kit-8), which is one of the methylthiazolyldiphenyl-tetrazoliumbromide (MTT) tests. 1 × 104 cells were seeded in a 48 well-plate. Then, the cells were cultured for 1 and 3 days, and the media was exchanged with the extractionmedium. The medium was removed from the cultured cells, washed with phosphate-buffered saline, and 500 µL WST-8 assay reagent was added to each well and incubated for 1.5 hours. The absorbance was measured at the 450-nm wavelength using the ELISA microplate reader (Model Spectra MAX PLUS; Molecular Devices, Sunnyvale, CA, USA) after 200 µL was transferred to the 96-well plate.
Nine male Sprague-Dawley rats (8 weeks old and 230 ± 20 g) were used and bred in an animal breeding center for 1 week. This animal test was conducted in compliance with the Declaration of Helsinki. Ethical clearance was approved by the Institutional Animal Care and Use Committee of the Jeonbuk University Laboratory Animal Center (JBNU 2020-0124). All groups used six mini-screws each, and they were sterilized with EO gas for 24 hours before implantation.
The rats were given general anesthesia by intramuscular injection of 0.1 mL/kg tiletamine and zolazepam (Zoletil 50; Virbac Laboratories, Carros, France) and 0.1 mL/kg xylazine hydrochloride (Rompun, Bayer Korea, Seoul, Korea). Additionally, 0.1–0.2 mL/kg of 2% lidocaine with 1:100,000 epinephrine (lidocaine-HCl; Huons, Seoul, Korea) was injected under the skin of the surgical site for local anesthesia. An incision was made on the lateral side of the tibia below the femur on both sides of the rat, and the skin and muscle were separated. Self-tapping of the sterilized mini-screws, one each on the distal side under tibia epiphysis, was vertically implanted into the bone. The rats were sacrificed by isoflurane (Hana Pharm Co., Ltd., Hwaseong, Korea) at 4 weeks after implantation.
The removal torque of the mini-screws was measured using a digital torque gauge (9810P; Aikoh Engineering Co., Osaka, Japan) with a precision of 0.1 N.cm after sacrificing the experimental rats at 4 weeks. After measuring the removal torque values, the surface of the mini-screws was observed by FE-SEM.
The bone blocks around the mini-screws were removed from the sacrificed rats and histologically observed. After fixation in 10% formalin solution for 2 days, they were washed with water and immersed in Villanueva solution (Polysciences, Inc., Warrington, PA, USA) for 3 days to stain the bone tissue. The stained bone blocks were dehydrated by increasing the concentration of ethanol (80, 90, 95, and 100%) and 100% acetone. It was then embedded by polymerization in methyl methacrylate with 2 wt.% benzoyl peroxide (Sigma-Aldrich, St. Louis, MO, USA) at 35°C for 1 day. The final specimens were polished to 40 μm thickness and observed with an optical microscope (DM2500; Leica Microsystem, Wetzlar, Germany).
There was no difference between the 1-day immersed surface (Figure 1A and 1D) and the non-immersed ASPH surface. After 2 days of immersion, protrusions appearing in the initial stage of hydroxyapatite precipitation were observed (Figure 1B and 1E), and on the 3rd day, the protrusions became more densified, growth occurred in the thickness direction (Figure 1C and 1F), and Ca and P concentrations increased (Table 2). From the 2nd day, bone-like apatite nanocrystals were observed in the shape of a needle on the high-magnification images (Figure 1E and 1F).
The Ti peaks were commonly observed in all groups. Ti and TiO2 peaks were observed in the AH and ASPH groups. Hydroxyapatite and octa calcium phosphate peaks were additionally observed in the ASPH group.
There was a significant difference between the UT (0.462 ± 0.008) and the AH (0.314 ± 0.009) group, and a significant difference was also found between the UT and the ASPH (0.308 ± 0.011) group at 1 day (p < 0.05). On the 3rd day, there was a significant difference between the UT (1.312 ± 0.106) and the AH (0.500 ± 0.018) group, and there was a significant difference between the AH and the ASHP (1.048 ± 0.032) group (p < 0.05), but there was no significant difference between the UT and the ASPH group (p > 0.05).
The values significantly increased in the following order: UT (2.08 ± 0.67 N·cm), AH (4.10 ± 0.72 N·cm), and ASPH (6.58 ± 0.66 N·cm) groups, and the ASPH group showed the highest value (p < 0.05).
The mini-screws of the UT group showed no adhesion to bone material and interfacial fracture. Only uniform directional machining traces generated by CNC machining were observed (Figure 5A and 5D). The AH (Figure 5B and 5E) and ASPH (Figure 5C and 5F) mini-screws showed bone material that was locally attached to the surface of the screw, and cohesive and interfacial fractures were mixed. The pattern was well observed in the ASPH group.
The new bone was formed between the threads of mini-screws and the bone interface in all groups. In the UT (Figure 6A and 6D) and AH groups (Figure 6B and 6E), the new bone did not extend to the thread or partially continued along the thread. In the ASPH group (Figure 6C and 6F), new bone was observed connected to the threads, and formation of a bony bridge connected to the adjacent bone was observed.
Unlike conventional orthodontic devices, the introduction of titanium orthodontic mini-screws minimizes unwanted tooth movement and makes it possible to apply orthodontic force in various directions.1-3 However, the titanium orthodontic mini-screws have the disadvantage of being easily removed due to inadequate osseointegration.6,14 To improve the clinical success rate, a method of inducing osseointegration between the bone and the titanium mini-screws has been proposed.14
The osseointegration of metallic implants is highly dependent on the material structure and texture. Processing often changes not only surface roughness, but also chemical factors such as wettability, solid/solution residues, and surface charge due to surface oxidation. For these reasons, it takes a long time for osseointegration to occur because the surface exhibits bioinert properties.15
The following methods are applied to improve osseointegration by changing the surface of the titanium orthodontic mini-screws. The first method is to add other materials to the surface of the mini-screws. One example is coating with hydroxyapatite or bioactive ceramic particles using the physicochemical method.16,17 The second method is to remove the less reactive surface layer created during the machining process. This method includes blasting treatment of high-strength ceramic particles, chemical acid etching, and electrochemical etching treatment methods.18-20 The third method is to change the properties of the mini-screw surface layer by ion deposition, anodization, laser, and plasma treatment methods. Typically, surface treatment methods change the morphology and roughness of the mini-screw surface layer or bond the bioactive materials on the surface.7,21,22
Methods of forming the micro/nanostructures on the surface of titanium mini-screws to increase their osseointegration ability have been investigated. According to several studies, the nanostructured surface has a larger surface area than the microstructured surface, leading to better osseointegration.19,23 The nanotube TiO2 layer produced by anodizing treatment has enough space to store various chemicals, including drugs. The space of the nanotube can be used as a carrier for delivery of drugs or bioactive materials. Because the drugs or bioactive materials react on the specific target site, they can exert a great effect even with small doses. This can reduce the systemic side effects that can occur when taking a large amount of drugs.24,25
Cyclic pre-calcification is one of the surface treatment methods that has been attempted to improve the bioactivity on the surface of the bioinert titanium orthodontic mini-screws.26,27 In this method, titanium is deposited in an aqueous solution containing phosphate and calcium ions, which are the main components of hydroxyapatite. The titanium surface induces an acid-base reaction between the TiO2 film layer and the ions.28 Nguyen et al.29 conducted studies in which nanotube layers were formed on the Ti-6Al-7Nb alloy implant and then the pre-calcification cycle was performed for 20 cycles in 0.05 M NaH2PO4 aqueous solution at 80°C and Ca(OH)2 saturated aqueous solution at 90°C. As a result, the bioactivity and osseointegration of the titanium surface were improved.
The results of our study showing three times higher removal torque values in the ASPH group than the UT group after 4 weeks of mini-screw implantation into the tibia of rats were consistent with findings of other studies which presented osseointergration was improved after anodization and cyclic pre-calcification.26,29 The increased removal torque values in the ASPH group may be attributed to the nanostructured surface that promotes bonding and contact strength. A previous study also reported that nanostructured surface promotes long-term bonding and contact strength which may lead to increased removal torque values and be more conducive to bone formation.30 Optical microscope analysis showed that more new bones were generated around the threads in the ASPH group, and they were uniformly distributed. The new bones were connected in the direction of the basal bone, confirming that osseointegration had increased.31 Osseous tissue materials were also attached to the surface of the mini-screws after the removal torque was measured, which is considered to be the result of stronger bonding of the new bone to the surface of the mini-screws.
The ASPH group was immersed in the SBF solution for 1–3 days, and the protrusions shown during the hydroxyapatite precipitation stage were uniformly distributed on the surface of the mini-screws. In addition, it was confirmed that the bioactivity was improved according to ISO 23317:2014 by increasing the contents of Ca and P, which are the main components of hydroxyapatite. Similar conclusions have been reported in other studies,15 in which the developed specific surface modifications can successfully improve the bone-bonding ability of titanium.
The effect on biocompatibility was evaluated by examining the cytotoxicity of the nanotube TiO2 layer formation and cyclic pre-calcification treatment method in this study. Results of the MTT assay demonstrated that there was no significant difference between the untreated UT group and the ASPH group treated with cyclic pre-calcification, confirming that there was biocompatibility according to ISO 10993-5:2009. In this study, the bioactivity and osseointegration were improved as a result of forming the TiO2 layer on the Ti-6Al-4V orthodontic mini-screws and performing cyclic pre-calcification in KH2PO4 and Ca(OH)2 aqueous solutions.
This study had limitations. First, in the in vivo test, the results were observed only 4 weeks after the implantation of the specimens. Second, in histological analysis, in vivo bone regeneration was simply investigated using only the Villanueva staining method. Therefore, further studies on the previously described limitations are needed to understand the mechanisms of bone formation.
In this study, it was investigated that the bioactive surface of Ti-6Al-4V alloy orthodontic mini-screws obtained mechanical retention and improved osseointegration. The Ca-P bioactive material was coated on the surface of the titanium mini-screws by the cyclic pre-calcification method after the TiO2 nanotubes were formed. Hydroxyapatite was uniformly distributed on the surface of the ASPH group immersed in the SBF solution, and the Ca-P content also increased over time. The removal torque values at 4 weeks after implantation of the surface-treated titanium mini-screws in the rat tibia were the highest in the ASPH group, and new bones connected to the threads were observed. It can be concluded that ASPH groups orthodontic mini-screws can be combined with bone in a short period of time with improved bioactivity. The osseointegration of the titanium orthodontic mini-screws is considered to be enhanced by the surface treatment of the nanotubes coated with Ca-P.
ACKNOWLEDGEMENTS
This study has reconstructed the data of Hye-Ji Kim Master’s thesis submitted in 2021 (title: “Effects of cyclic pre-calcification treatment on bioactivity of Ti-6Al-4V alloy miniscrews”).
REFERENCES
1. Kakali L, Alharbi M, Pandis N, Gkantidis N, Kloukos D. 2019; Success of palatal implants or mini-screws placed median or paramedian for the reinforcement of anchorage during orthodontic treatment: a systematic review. Eur J Orthod. 41:9–20. DOI: 10.1093/ejo/cjy015. PMID: 29608666. PMID: https://www.scopus.com/inward/record.uri?partnerID=HzOxMe3b&scp=85060584713&origin=inward.
2. Hergel CA, Acar YB, Ates M, Kucukkeles N. 2019; In-vitro evaluation of the effects of insertion and sterilization procedures on the mechanical and surface characteristics of mini screws. Eur Oral Res. 53:25–31. DOI: 10.26650/eor.20197993. PMID: 31309189. PMCID: PMC6612762.
3. Oswal S, Agarkar SS, Jethe S, Yerawadekar S, Kawale P, Deshmukh S, et al. 2020; Clinical use of orthodontic mini-implants for intrusion and retraction: a systematic review. Australas Orthod J. 36:87–100. DOI: 10.21307/aoj-2020-011. PMID: 3cf6a92d071b40c78885afe2e9b622af. PMID: https://www.scopus.com/inward/record.uri?partnerID=HzOxMe3b&scp=85134233501&origin=inward.
4. Mecenas P, Espinosa DG, Cardoso PC, Normando D. 2020; Stainless steel or titanium mini-implants? Angle Orthod. 90:587–97. DOI: 10.2319/081619-536.1. PMID: 33378494. PMCID: PMC8028470. PMID: https://www.scopus.com/inward/record.uri?partnerID=HzOxMe3b&scp=85088590598&origin=inward.
5. Reynders R, Ronchi L, Bipat S. 2009; Mini-implants in orthodontics: a systematic review of the literature. Am J Orthod Dentofacial Orthop. 135:564.e1–19. discussion 564–5. DOI: 10.1016/j.ajodo.2008.09.026. PMID: 19409331. PMID: https://www.scopus.com/inward/record.uri?partnerID=HzOxMe3b&scp=65249139979&origin=inward.
6. Moon YY, Lee MH, Song KW, Lee MH, Bae TS. 2008; Characteristics of TiO2 nanotube on Ti-6Al-4V alloy. J Korean Res Soc Dent Mater. 35:339–48.
7. El-Zehary RR, Tawfik MA, Mourad SI, Enan ET. 2021; Influence of orthodontic mini-screw surface modifications on implant topography and osseointegration: an in-vivo study. Tang Egypt Dent J. 67:1291–305. DOI: 10.21608/edj.2021.63997.1512.
8. Xia L, Xie Y, Fang B, Wang X, Lin K. 2018; In situ modulation of crystallinity and nano-structures to enhance the stability and osseointegration of hydroxyapatite coatings on Ti-6Al-4V implants. Chem Eng J. 347:711–20. DOI: 10.1016/j.cej.2018.04.045. PMID: https://www.scopus.com/inward/record.uri?partnerID=HzOxMe3b&scp=85046146746&origin=inward.
9. Mehnath S, Arjama M, Rajan M, Premkumar K, Karthikeyan K, Jeyaraj M. 2020; Mineralization of bioactive marine sponge and electrophoretic deposition on Ti-6Al-4V implant for osteointegration. Surf Coat Technol. 392:125727. DOI: 10.1016/j.surfcoat.2020.125727. PMID: https://www.scopus.com/inward/record.uri?partnerID=HzOxMe3b&scp=85083313122&origin=inward.
10. Kim SP, Cho HR, Choe HC. 2021; Bioactive element coatings on nano-mesh formed Ti-6Al-4V alloy surface using plasma electrolytic oxidation. Surf Coat Technol. 406:126649. DOI: 10.1016/j.surfcoat.2020.126649. PMID: https://www.scopus.com/inward/record.uri?partnerID=HzOxMe3b&scp=85096403301&origin=inward.
11. Lin Q, Huang D, Du J, Wei Y, Hu Y, Lian X, et al. 2019; Nano-hydroxyapatite crystal formation based on calcified TiO2 nanotube arrays. Appl Surf Sci. 478:237–46. DOI: 10.1016/j.apsusc.2019.01.226. PMID: https://www.scopus.com/inward/record.uri?partnerID=HzOxMe3b&scp=85060768869&origin=inward.
12. Kodama A, Bauer S, Komatsu A, Asoh H, Ono S, Schmuki P. 2009; Bioactivation of titanium surfaces using coatings of TiO2 nanotubes rapidly pre-loaded with synthetic hydroxyapatite. Acta Biomater. 5:2322–30. DOI: 10.1016/j.actbio.2009.02.032. PMID: 19332383. PMID: https://www.scopus.com/inward/record.uri?partnerID=HzOxMe3b&scp=67349141019&origin=inward.
13. Nguyen TDT, Park IS, Lee MH, Bae TS. 2013; Enhanced biocompatibility of a pre-calcified nanotubular TiO2 layer on Ti-6Al-7Nb alloy. Surf Coat Technol. 236:127–34. DOI: 10.1016/j.surfcoat.2013.09.038. PMID: https://www.scopus.com/inward/record.uri?partnerID=HzOxMe3b&scp=84888848901&origin=inward.
14. Zhang L, Zhao Z, Li Y, Wu J, Zheng L, Tang T. 2010; Osseointegration of orthodontic micro-screws after immediate and early loading. Angle Orthod. 80:354–60. DOI: 10.2319/021909-106.1. PMID: 19905862. PMCID: PMC8973229. PMID: https://www.scopus.com/inward/record.uri?partnerID=HzOxMe3b&scp=73349094746&origin=inward.
15. Baino F, Yamaguchi S. 2020; The use of simulated body fluid (SBF) for assessing materials bioactivity in the context of tissue engineering: review and challenges. Biomimetics (Basel). 5:57. DOI: 10.3390/biomimetics5040057. PMID: 33138246. PMCID: PMC7709622. PMID: 423061ae851d4d94a7dc9fd870c08ab9. PMID: https://www.scopus.com/inward/record.uri?partnerID=HzOxMe3b&scp=85094632327&origin=inward.
16. Li Y, Li B, Song Y, Ma A, Li C, Zhang X, et al. 2019; Improved osteoblast adhesion and osseointegration on TiO2 nanotubes surface with hydroxyapatite coating. Dent Mater J. 38:278–86. DOI: 10.4012/dmj.2018-118. PMID: 30541994. PMID: https://www.scopus.com/inward/record.uri?partnerID=HzOxMe3b&scp=85064003930&origin=inward.
17. Zhou Z, Shi Q, Wang J, Chen X, Hao Y, Zhang Y, et al. 2021; The unfavorable role of titanium particles released from dental implants. Nanotheranostics. 5:321–32. DOI: 10.7150/ntno.56401. PMID: 33732603. PMCID: PMC7961127. PMID: https://www.scopus.com/inward/record.uri?partnerID=HzOxMe3b&scp=85103143661&origin=inward.
18. Moghaddam SF, Mohammadi A, Behroozian A. 2021; The effect of sandblasting and acid etching on survival rate of orthodontic miniscrews: a split-mouth randomized controlled trial. Prog Orthod. 22:2. DOI: 10.1186/s40510-020-00347-z. PMID: 33409700. PMCID: PMC7788110. PMID: 39aa49823b1b42e99727b65a5d40f704. PMID: https://www.scopus.com/inward/record.uri?partnerID=HzOxMe3b&scp=85098772550&origin=inward.
19. Cheng Y, Yang H, Yang Y, Huang J, Wu K, Chen Z, et al. 2018; Progress in TiO2 nanotube coatings for biomedical applications: a review. J Mater Chem B. 6:1862–86. DOI: 10.1039/C8TB00149A. PMID: 32254353. PMID: https://www.scopus.com/inward/record.uri?partnerID=HzOxMe3b&scp=85044770698&origin=inward.
20. Stich T, Alagboso F, Křenek T, Kovářík T, Alt V, Docheva D. 2021; Implant-bone-interface: reviewing the impact of titanium surface modifications on osteogenic processes in vitro and in vivo. Bioeng Transl Med. 7:e10239. DOI: 10.1002/btm2.10239. PMID: 35079626. PMCID: PMC8780039. PMID: 5bfc36652be74fd1abd02f70f98d8a6a. PMID: https://www.scopus.com/inward/record.uri?partnerID=HzOxMe3b&scp=85109729182&origin=inward.
21. El-Mezayen MA, Gaber KM, Refai WM. 2022; Detection of bone deposition on the surface of immediately removed or left mini-screw after canine retraction (SEM Study). Egypt Orthod J. 61:12–23. DOI: 10.21608/eos.2021.107405.1037.
22. Jiman PA, Prodan D, Moldovan M, Muntean A, Sarosi C, Tarmure V, et al. 2021; New and recovered temporary anchorage devices, in vitro assessment of structural and surface properties. Materials (Basel). 14:6271. DOI: 10.3390/ma14216271. PMID: 34771795. PMCID: PMC8584799. PMID: aaaa799a0d1f4ba28d814bbcc8c2d390. PMID: https://www.scopus.com/inward/record.uri?partnerID=HzOxMe3b&scp=85117811723&origin=inward.
23. Bucur SM, Vaida LL, Olteanu CD, Checchi V. 2021; A brief review on micro-implants and their use in orthodontics and dentofacial orthopaedics. Appl Sci. 11:10719. DOI: 10.3390/app112210719. PMID: 778f92d47d164116b344e0c7dd70f68f. PMID: https://www.scopus.com/inward/record.uri?partnerID=HzOxMe3b&scp=85119268509&origin=inward.
24. Łosiewicz B, Stróż A, Osak P, Maszybrocka J, Gerle A, Dudek K, et al. 2021; Production, characterization and application of oxide nanotubes on Ti-6Al-7Nb alloy as a potential drug carrier. Materials (Basel). 14:6142. DOI: 10.3390/ma14206142. PMID: 34683734. PMCID: PMC8538941. PMID: 422563c12efb43319e63fc84f9c6ec6b. PMID: https://www.scopus.com/inward/record.uri?partnerID=HzOxMe3b&scp=85117573517&origin=inward.
25. Wang K, Jin H, Song Q, Huo J, Zhang J, Li P. 2021; Titanium dioxide nanotubes as drug carriers for infection control and osteogenesis of bone implants. Drug Deliv Transl Res. 11:1456–74. DOI: 10.1007/s13346-021-00980-z. PMID: 33942245. PMID: https://www.scopus.com/inward/record.uri?partnerID=HzOxMe3b&scp=85105376065&origin=inward.
26. Oh EJ, Nguyen TD, Lee SY, Jeon YM, Bae TS, Kim JG. 2014; Enhanced compatibility and initial stability of Ti6Al4V alloy orthodontic miniscrews subjected to anodization, cyclic precalcification, and heat treatment. Korean J Orthod. 44:246–53. DOI: 10.4041/kjod.2014.44.5.246. PMID: 25309864. PMCID: PMC4192526. PMID: https://www.scopus.com/inward/record.uri?partnerID=HzOxMe3b&scp=84907908008&origin=inward.
27. Kim HY, Kim SC. 2016; Bone cutting capacity and osseointegration of surface-treated orthodontic mini-implants. Korean J Orthod. 46:386–94. DOI: 10.4041/kjod.2016.46.6.386. PMID: 27896213. PMCID: PMC5118218. PMID: https://www.scopus.com/inward/record.uri?partnerID=HzOxMe3b&scp=84995662772&origin=inward.
28. Nguyen TT, Jang YS, Kim YK, Kim SY, Lee MH, Bae TS. 2019; Osteogenesis-related gene expression and guided bone regeneration of a strontium-doped calcium-phosphate-coated titanium mesh. ACS Biomater Sci Eng. 5:6715–24. DOI: 10.1021/acsbiomaterials.9b01042. PMID: 33423489. PMID: https://www.scopus.com/inward/record.uri?partnerID=HzOxMe3b&scp=85075023799&origin=inward.
29. Nguyen TD, Moon SH, Oh TJ, Park IS, Lee MH, Bae TS. 2015; The effect of APH treatment on surface bonding and osseointegration of Ti-6Al-7Nb implants: an in vitro and in vivo study. J Biomed Mater Res B Appl Biomater. 103:641–8. DOI: 10.1002/jbm.b.33210. PMID: 24976109. PMID: https://www.scopus.com/inward/record.uri?partnerID=HzOxMe3b&scp=84925682824&origin=inward.
30. Johansson P, Barkarmo S, Hawthan M, Peruzzi N, Kjellin P, Wennerberg A. 2018; Biomechanical, histological, and computed X-ray tomographic analyses of hydroxyapatite coated PEEK implants in an extended healing model in rabbit. J Biomed Mater Res A. 106:1440–7. DOI: 10.1002/jbm.a.36345. PMID: 29341426. PMID: https://www.scopus.com/inward/record.uri?partnerID=HzOxMe3b&scp=85042167153&origin=inward.
31. Im C, Park JH, Jeon YM, Kim JG, Jang YS, Lee MH, et al. 2022; Improvement of osseointegration of Ti-6Al-4V ELI alloy orthodontic mini-screws through anodization, cyclic pre-calcification, and heat treatments. Prog Orthod. 23:11. DOI: 10.1186/s40510-022-00405-8. PMID: 35368222. PMCID: PMC8977256. PMID: 9a48620e4c7245dab8337a21f3e64b7e. PMID: https://www.scopus.com/inward/record.uri?partnerID=HzOxMe3b&scp=85127523040&origin=inward.
Figure 1
FE-SEM images (×5,000 in top row and ×50,000 in bottom row) of ASPH group immersed in SBF for 1 day (A, D), 2 days (B, E), and 3 days (C, F).
FE-SEM, field emission scanning electron microscope; ASPH, anodized treatment followed by cyclic pre-calcification; SBF, simulated body fluid.
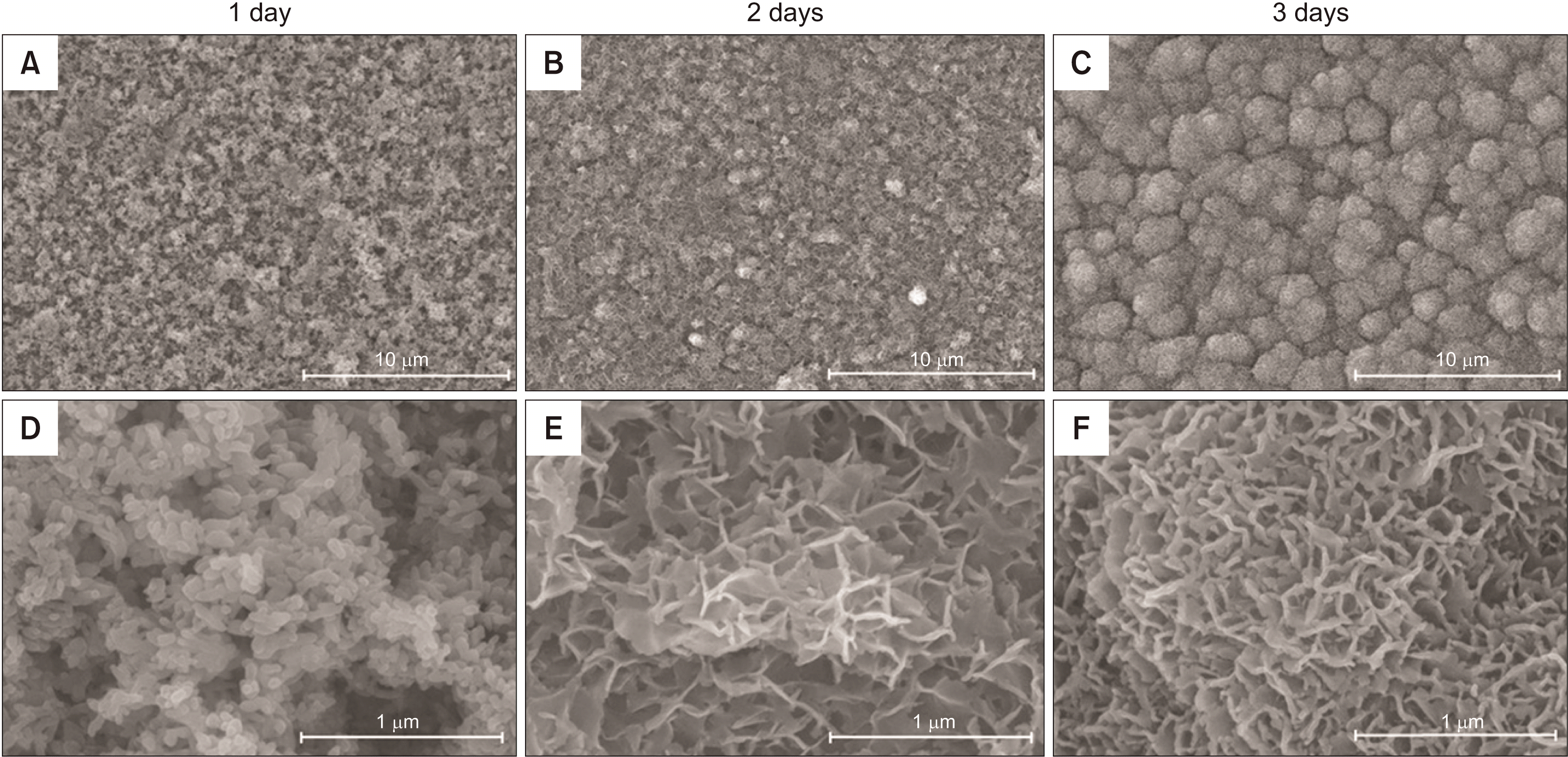
Figure 2
X-ray diffraction analysis of UT, AH, and ASPH groups.
UT, untreated group; AH, anodized and heat-treated group; ASPH, anodized treatment followed by cyclic pre-calcification group.
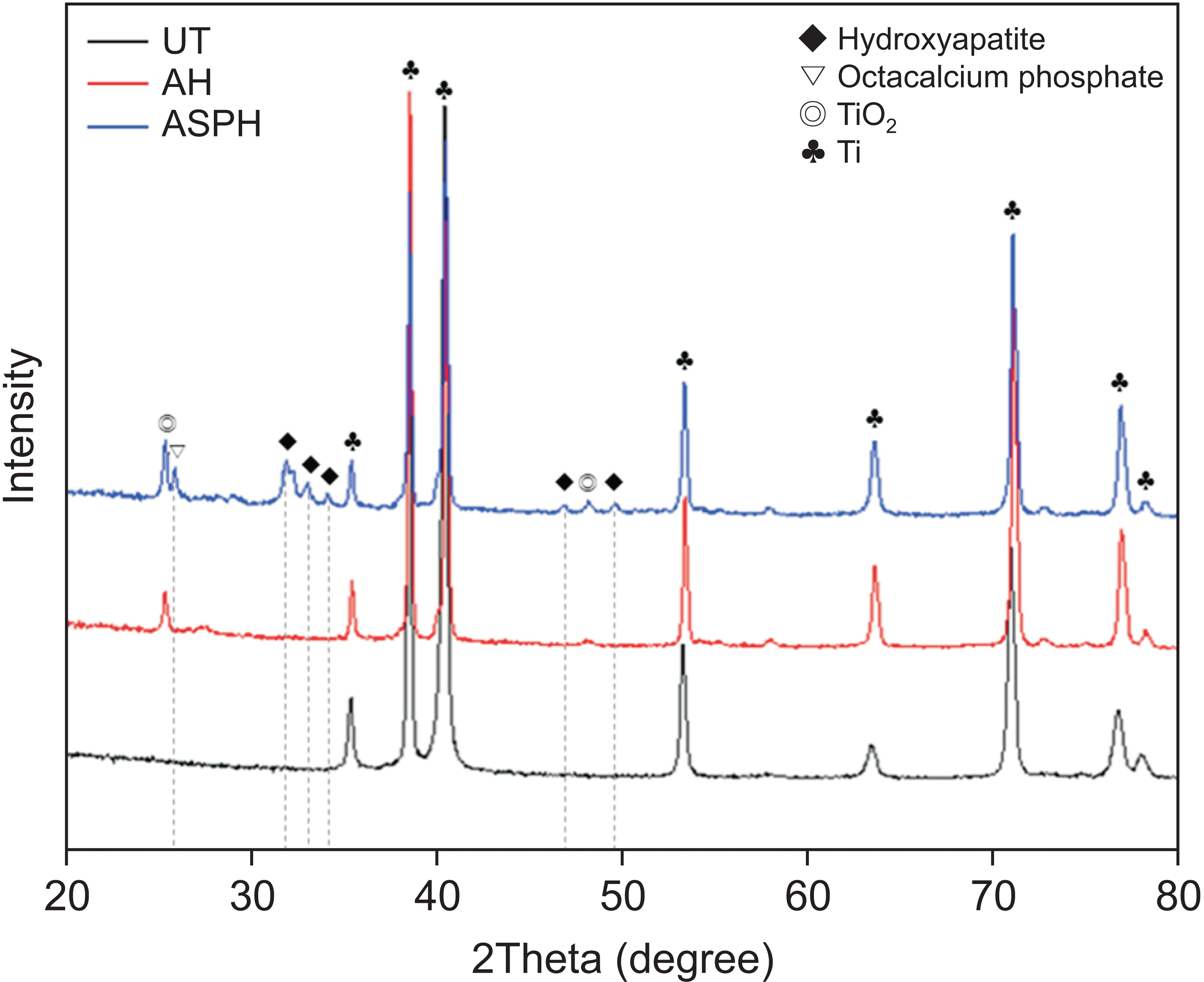
Figure 3
Cytotoxicity test of MC3T3-E1 osteoblast cells in the UT, AH, and ASPH groups for 1 and 3 days (MTT assay). The results are presented as the means ± standard deviation.
UT, untreated group; AH, anodized and heat-treated group; ASPH, anodized treatment followed by cyclic pre-calcification group; MTT, methylthiazolyldiphenyl-tetrazolium bromide.
*p < 0.05.

Figure 4
Mini-screws removal torque values of the UT, AH, and ASPH groups at 4 weeks after implantation. The results are presented as the means ± standard deviation.
UT, untreated group; AH, anodized and heat-treated group; ASPH, anodized treatment followed by cyclic pre-calcification group.
*p < 0.05.
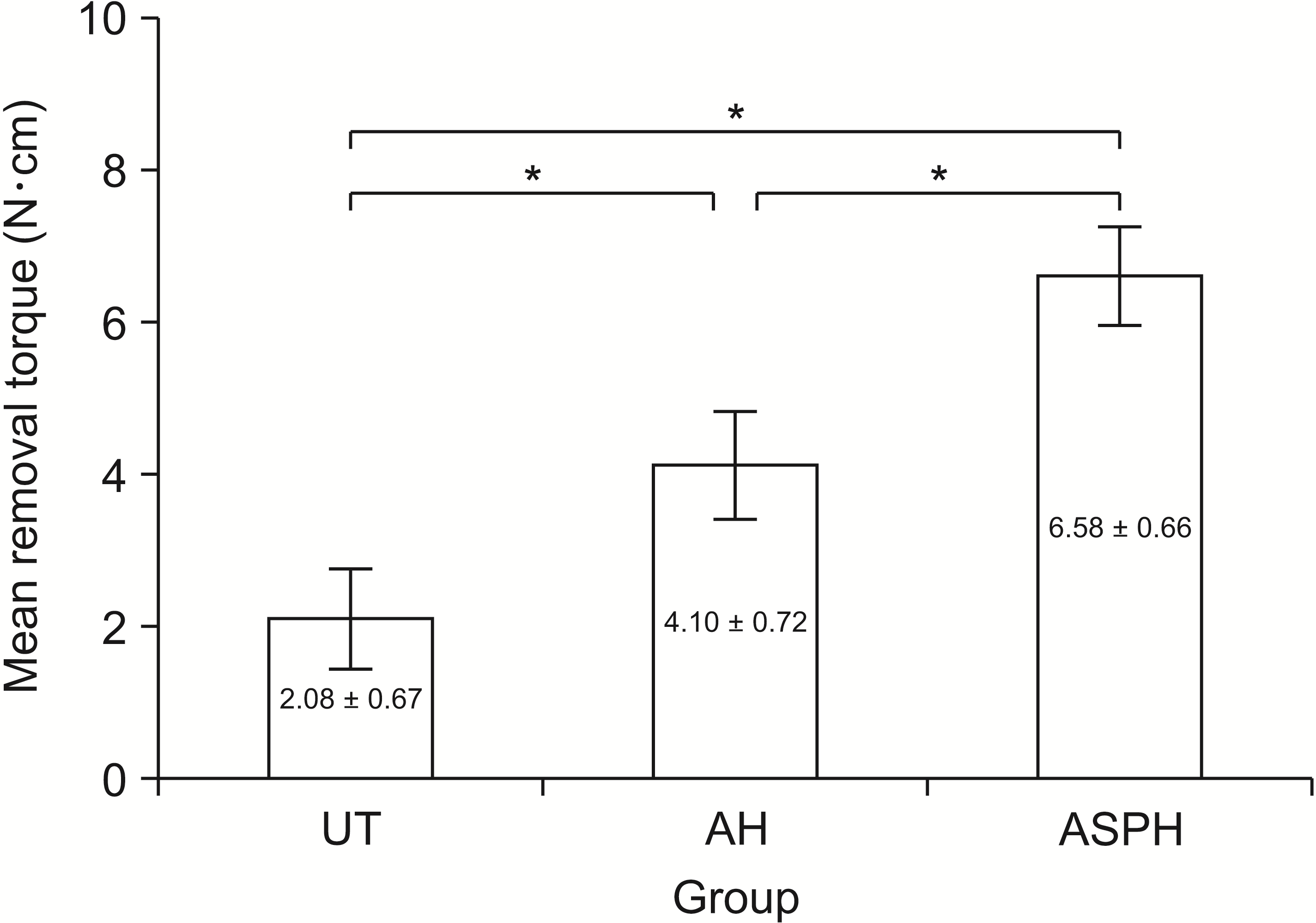
Figure 5
Surface morphology (×40) of mini-screws removed after implantation in rat tibia for 4 weeks. UT (A, D), AH (B, E), and ASPH (C, F) groups. The black squares show high-magnification images (×100).
UT, untreated group; AH, anodized and heat-treated group; ASPH, anodized treatment followed by cyclic pre-calcification group.
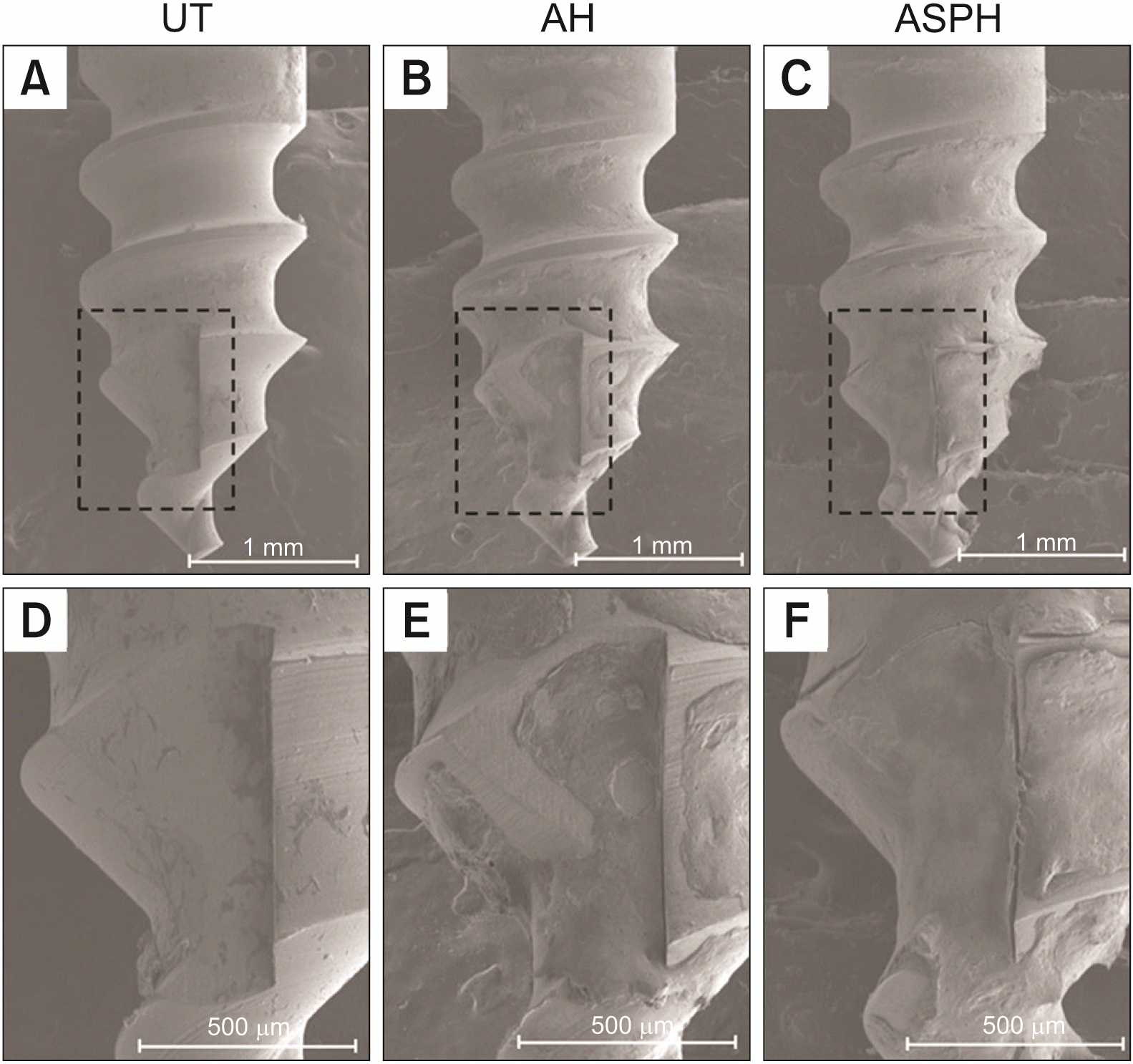
Figure 6
Optical microscope analysis (×30) after 4 weeks of mini-screws implantation. Surface morphology of UT (A, D), AH (B, E), and ASPH (C, F) group mini-screws removed after implantation in rat tibia for 4 weeks. The black squares show high-magnification images (×100). The red arrow indicates osteogenesis.
UT, untreated group; AH, anodized and heat-treated group; ASPH, anodized treatment followed by cyclic pre-calcification group.
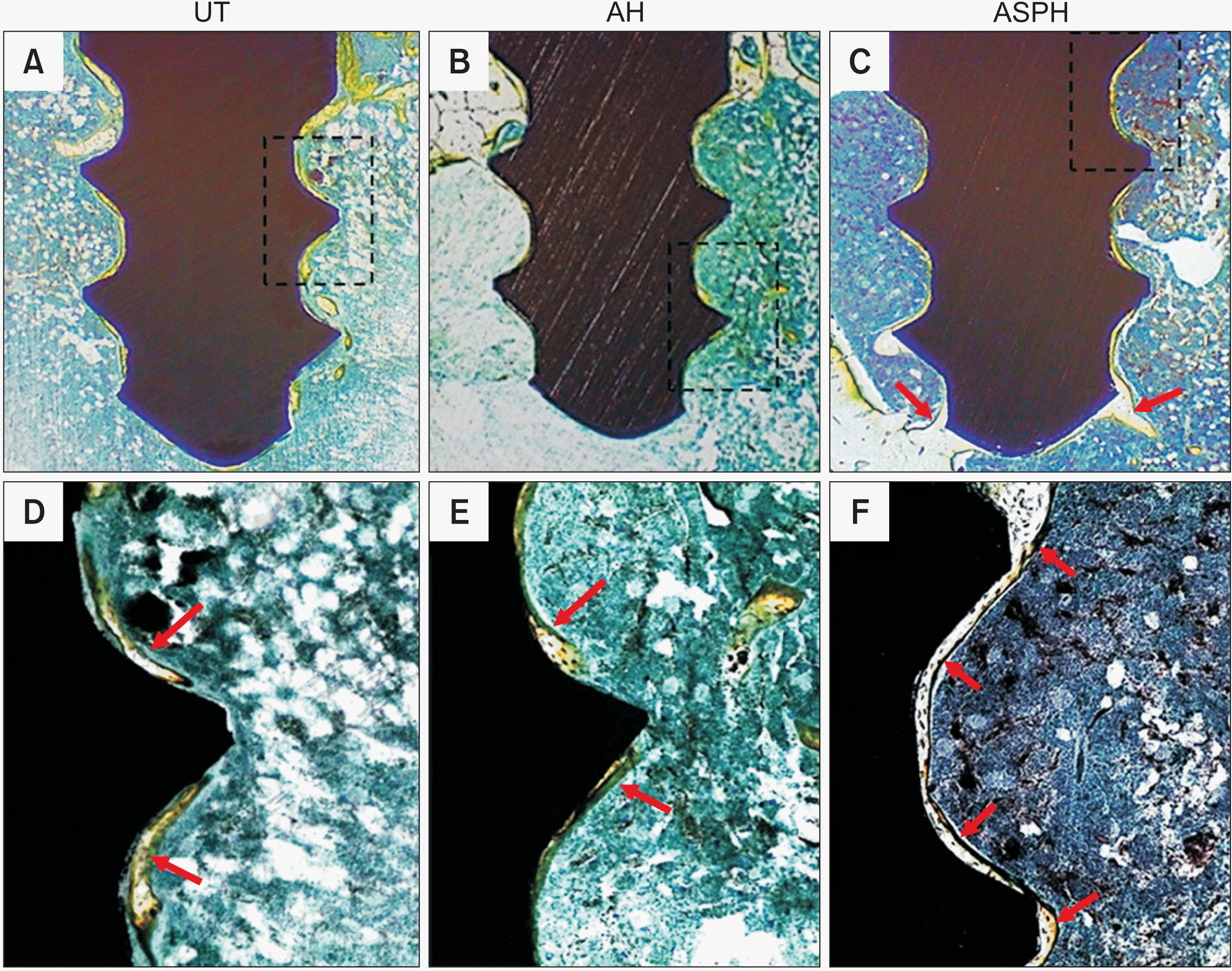