Abstract
WNT signaling plays an important role in cardiac development, but abnormal activity is often associated with cardiac hypertrophy, myocardial infarction, remodeling, and heart failure. The effect of WNT signaling on regulation of atrial natriuretic peptide (ANP) secretion is unclear. Therefore, the purpose of this study was to investigate the effect of Wnt agonist 1 (Wnta1) on ANP secretion and mechanical dynamics in beating rat atria. Wnta1 treatment significantly increased atrial ANP secretion and pulse pressure; these effects were blocked by U73122, an antagonist of phospholipase C. U73122 also abolished the effects of Wnta1-mediated upregulation of protein kinase C (PKC) β and γ expression, and the PKC antagonist Go 6983 eliminated Wnta1-induced secretion of ANP. In addition, Wnta1 upregulated levels of phospho-transforming growth factor-β activated kinase 1 (p-TAK1), TAK1 banding 1 (TAB1) and phospho-activating transcription factor 2 (p-ATF2); these effects were blocked by both U73122 and Go 6983. Wnta1-induced ATF2 was abrogated by inhibition of TAK1. Furthermore, Wnta1 upregulated the expression of T cell factor (TCF) 3, TCF4, and lymphoid enhancer factor 1 (LEF1), and these effects were blocked by U73122 and Go 6983. Tak1 inhibition abolished the Wnta1-induced expression of TCF3, TCF4, and LEF1 and Wnta1-mediated ANP secretion and changes in mechanical dynamics. These results suggest that Wnta1 increased the secretion of ANP and mechanical dynamics in beating rat atria by activation of PKC–TAK1–ATF2–TCF3/LEF1 and TCF4/LEF1 signaling mainly via the WNT/Ca2+ pathway. It is also suggested that WNT–ANP signaling is implicated in cardiac physiology and pathophysiology.
Atrial natriuretic peptide (ANP) is a member of the family of cardiac natriuretic peptides secreted mainly from the cardiac atria [1]. As a peptide hormone that regulates body fluid volume and blood pressure homeostasis, ANP also possesses anti-inflammatory, anti-oxidant, anti-ischemic/hypoxic, and anti-hypertrophic properties [2-5]. Recently, it was discovered that ANP exhibits important anti-cancer functions, including inhibition of metabolism, inflammation, differentiation, proliferation and regulation of WNT signaling and pH in cancer cells [6-8].
WNT signal transduction is an elaborate and complex aggregation of signaling pathways that regulate a wide range of cellular functions during development and in adult organisms. WNT signaling pathways comprise the canonical WNT/β-catenin pathway, non-canonical WNT/planar cell polarity (PCP) pathway, and WNT/Ca2+ dependent pathway [9]. In the canonical WNT pathway, activated WNT phosphorylates the dishevelled (DVL) protein leading to dissociation of β-catenin from its destruction complex. Subsequently, β-catenin translocates to the nucleus and interacts with transcription factors including T cell factor (TCF)/lymphoid enhancer-binding factor (LEF). After activation by phosphorylated DVL, the WNT/PCP pathway is mediated by signaling through the small GTPases RhoA and Rac and Rho-associated kinase (ROCK) or c-Jun N-terminal kinase. In the WNT/Ca2+ pathway, recruited G-proteins activate phospholipase C (PLC) leading to Ca2+ accumulation and activation of calmodulin-dependent kinase II, calcineurin, and protein kinase C (PKC) [9]. The WNT signaling pathways control cell growth, differentiation, apoptosis, and homeostatic self-renewal, but abnormalities in these pathways often lead to developmental aberrations and disease, such as tumors or neurological and bone diseases [10,11]. Recent studies have shown that abnormal activity of WNT signaling pathways is closely related to the occurrence and development of myocardial ischemic/hypoxic injury, hypertrophy, fibrosis, arrhythmia, myocardial infarction, and heart failure [9,12-15]. It was reported that siRNA knockdown of β-catenin in cardiomyocytes resulted in suppression of ANP transcription. The Anp gene promoter contains an LEF1 binding site to which β-catenin is recruited during phenylephrine stimulation [16]; however, the effect of WNT signaling on the regulation of atrial ANP secretion is not clear. The aim of this study was to investigate the effect of WNT signaling on the regulation of atrial ANP secretion using a WNT signaling agonist in isolated perfused beating rat atria.
The phospholipase C antagonist U73122, transforming growth factor-β activated kinase 1 (TAK1) inhibitor 1, and inhibitor of PKC Go 6983 were purchased from MedChemExpress (Monmouth Junction, NJ, USA). The Wnt agonist 1 (Wnta1) was purchased from APExBIO Technology LLC (Houston, TX, USA).
Sprague–Dawley rats of both sexes weighing 260–300 g each were used. The animal procedures used in the study were approved by the Animal Care and Use Committee of Yanbian University and were in accordance with the animal welfare guidelines of the US National Institutes of Health. The protocol approval number was SCXK (Ji) 2011-006. Rats were anesthetized by intraperitoneal injection of sodium pentobarbital (90 mg/kg body weight), and isolated perfused, beating left atria were prepared as previously described [17]. The atrium from each perfusion was stimulated at 1.5 Hz (0.3 ms, 30–40 V) with a luminal electrode, and the atrium was perfused with HEPES buffer solution using a peristaltic pump (1.0 ml/min) to allow atrial pacing. The HEPES buffer contained (in mM) 118 NaCl, 4.7 KCl, 2.5 CaCl2, 1.2 MgCl2, 25 NaHCO3, 10 glucose, 10 HEPES (pH 7.4 with NaOH), and 0.1% bovine serum albumin.
The rats were randomly divided into control, Wnta1, U73122, U73122 + Wnta1, Go 6983, Go 6983 + Wnta1, TAK1 inhibitor 1, and TAK1 inhibitor 1 + Wnta1 groups (n = 6 per group). The atria were perfused for 60 min to stabilize atrial mechanical dynamics and ANP secretion. The atrial perfusates were collected at 2-min intervals at 4°C to measure ANP levels. The experimental cycles in this study were 12 min each. Two control cycles were followed by an infusion of Wnta1 (10 μM) for four cycles to determine changes in atrial ANP secretion and mechanical dynamics. To explore the mechanism by which Wnta1 induced atrial ANP secretion and mechanical dynamics, another series of experiments was performed. One control cycle was followed by an introduction of a specific inhibitor for one cycle followed by the same inhibitor + Wnta1 for four cycles. The concentrations of each inhibitor were as follows (in μM): 15 U73122, 0.3 Go 6983, and 1 TAK1 inhibitor 1. Immediately after perfusion, the atrial tissue was frozen and stored at −80°C for subsequent western blotting analysis.
Preparation of protein homogenates, Western blot analysis, autoradiography, and densitometry were performed as described previously [17]. Briefly, left atrial tissue was homogenized in radioimmunoprecipitation assay lysis buffer (Solarbio Institute of Biotechnology, Shanghai, China). Protein concentrations were determined using an enhanced BCA protein assay kit (Beyotime Biotechnology, Shanghai, China). Protein extracts were boiled in Lane Maker Loading Buffer (Cwbio, Beijing, China). Electrophoresis was carried out in 8% or 10% sodium dodecyl sulfate-polyacrylamide gels. Proteins were transferred to polyvinylidene difluoride filter membranes (Beyotime Biotechnology) that were subsequently blocked with 5% nonfat dry milk in phosphate-buffered saline at room temperature. After 2 h, the membranes were incubated with primary antibodies at 4°C overnight, washed, and then incubated with a secondary antibody for 2 h at room temperature. Protein bands were visualized with the Blotting ECL Substrate (ECL Western blot kit, RB-SRCHLGT; RayBiotech Life, Peachtree Corners, GA, USA). Densitometry of the western blots was performed using the open source ImageJ software (National Institutes of Health, Bethesda, MD, USA). The antibodies used in this study were as follows: anti-β-actin (1:1,000, AP0060; Bioworld Technology, Wuhan, China), polyclonal anti-PKCβ (1:1,000, ENT3756; Elabscience, Wuhan, China), polyclonal anti-PKCγ (1:1,000, E-AB-13012; Elabscience), polyclonal TAB1 antibody (1:1,000, DF7471; Affinity, Changzhou, China), phospho-TAK1 (Thr184/Thr187) antibody (1:1,000, AF4379), polyclonal anti-TAK1 (1:500, 12330-2-AP; Proteintech, Wuhan, China), phospho-ATF2 (T69/T71) antibody (1:1,000, AP0525; ABclonal, Wuhan, China), polyclonal anti-ATF2 (1:1,000, 11908-1-AP; Proteintech), anti-LEF1 Antibody (1:1,000, DF7570; Affinity), polyclonal anti-TCF3 (1:1,000, 14519-1-AP; Proteintech), polyclonal anti-TCF4 (1:2,000, 13838-1-AP; Proteintech), and horseradish peroxidase-conjugated anti-rabbit secondary antibody (1:3,000, AP132P, Nachuan Biotech, Changchun, China).
As described previously [18], the levels of immunoreactive ANP in the perfusates were measured using the iodine [125I] Atrial Natriuretic Factor Radioimmunoassay Kit (North Institute of Biological Technology, Beijing, China). Intra-atrial pressure was recorded using a Physiograph (RM6240BD; Techman Instrument, Chengdu, China) via a pressure transducer (Statham P23Db; Statham Inc., Oxnard, CA, USA) and pulse pressure was calculated from the difference between systolic and diastolic pressures.
Changes in atrial pulse pressure (fold) = value of pulse pressure-mean value of basal pulse pressure (cm H2O)/mean value of basal pulse pressure (cm H2O).
The statistical data were analyzed with Prism software, version 7 (GraphPad Software, San Diego, CA, USA). Significant differences were compared using repeated-measures ANOVA followed by Bonferroni’s multiple comparison test. Statistical significance was defined as p < 0.05. All data are presented as means ± SEM.
To identify the effects of WNT signaling on ANP secretion and mechanical dynamics in isolated beating rat atria, Wnta1 was used in the experiments. Results showed that Wnta1 treatment significantly increased ANP secretion in a time-dependent manner (p < 0.05 vs. the control period, Fig. 1A). The atrial pulse pressure was also enhanced by Wnta1 and appeared relatively stable after reaching a peak level (p < 0.05 vs. the control period, Fig. 1B). These data hint that Wnta1 promoted ANP secretion and positive inotropic effects mainly via the WNT/Ca2+ signaling pathway in isolated beating rat atria.
To explore the mechanism of Wnta1-induced ANP secretion and changes in mechanical dynamics, experiments were performed with U73122, an antagonist of PLC. As shown in Fig. 2, the Wnta1-induced increase in ANP secretion was markedly decreased by U73122 (p < 0.05 vs. the Wnta1 period, Fig. 2A). The enhanced mechanical dynamics induced by Wnta1 were also blocked and reversed by U73122, resulting in negative inotropy (p < 0.05 vs. the control and Wnta1 periods, Fig. 2B). The data indicate that Wnta1 increased atrial ANP secretion and enhanced mechanical dynamics by activating PLC.
To clarify the downstream signaling of PLC, the expression of PKC isozymes induced by Wnta1 was determined. The data showed that Wnta1 upregulated the expression of PKCβ (Fig. 3A) and PKCγ (Fig. 3B) (p < 0.05 vs. the control group). In the presence of U73122, the expression of PKCβ and PKCγ expression was reduced to a level below the control value (p < 0.05 vs. the control and Wnta1 groups, Fig. 3A and B). The expression of PKCβ was downregulated by U73122 alone (p < 0.05 vs. the control group); however, the expression of PKCγ was slightly decreased by U73122, but not significantly. To determine the effects of Wnta1-induced PKC expression on atrial ANP secretion and mechanical dynamics, the PKC inhibitor Go 6983 was used. In the presence of Go 6983, the increase in ANP secretion induced by Wnta1 was eliminated (p < 0.05 vs. the Wnta1 period, Fig. 4A). Wnta1-augmented atrial mechanical dynamics were also blocked by Go 6983 (p < 0.05 vs. the control and Wnta1 periods, Fig. 4B). These results signify that PLC-induced PKC is involved in the regulation of Wnta1-induced atrial ANP secretion and mechanical dynamics.
Because of that PKC is involved in TAK1 activation as a signaling mediator subsequently leads to ATF-2 activation through MKK3/6-p38 mitogen-activated protein kinase, which ultimately affects cardiac hypertrophic responses [19]. Therefore, to further define the downstream mechanism involved in Wnta1-induced atrial ANP secretion and mechanical dynamics, the effects of PKC inhibition on the expression of TAB1, TAK1 and ATF2 were investigated. As shown in Fig. 5, Wnta1 significantly upregulated the expression of TAB1 (p < 0.05 vs. the control group, A and B), this was abolished by U73122 and Go 6983 pretreatment (p < 0.05 vs. the Wnta1 group, A and B). The levels of phosphor-TAK1 and total-TAK1 were markedly upregulated by Wnta1 (p < 0.05 vs. the control group, Fig. 6A–D), and upregulation were completely blocked by U73122 and Go 6983 pretreatment (p < 0.05 vs. the control and Wnta1 groups, Fig. 6A, B and C, D). Similarly, the level of phosphor-ATF2 was notably upregulated by Wnta1 (p < 0.05 vs. the control group, Fig. 7). phosphor-ATF2 upregulation was also abolished by treatment with U73122, Go 6983, and the TAK1 inhibitor (p < 0.05 vs. the control group, Fig. 7). These results suggest that, during WNT stimulation, PLC-induced PKC regulates ATF2 activity by activating TAK1.
To determine the role of TAK1 on TCF/LEF1 expression and ANP secretion, the effects of TAK1 inhibition on Wnta1-induced TCF/LEF1 expression and ANP secretion were analyzed. The expression of TCF3, TCF4, and LEF1 were upregulated by Wnta1 treatment (p < 0.05 vs. the control group, Figs. 8 and 9), and these effects were completely abolished by U73122 and Go 6983 pretreatment (p < 0.05 vs. the Wnta1 group, Figs. 8A, B, D, E and 9A, B). Comparably, treatment with the TAK1 inhibitor also abrogated the expression of TCF3, TCF4 and LEF1 (p < 0.05 vs. the Wnta1 group, Figs. 8C, F and 9C), the secretion of atrial ANP, and changes in mechanical dynamics induced by Wnta1 (p < 0.05 vs. the Wnta1 period, Fig. 10). These data demonstrate that the Wnta1-induced TCF3, TCF4, and LEF1 expression is activated by TAK1 and involved in the regulation of ANP secretion.
This study showed that Wnta1 significantly upregulated the expression of PKCβ and PKCγ isozymes leading to elevation of TAK1, ATF2, TCF3, TCF4, and LEF1 protein levels and concomitant increases in ANP secretion and mechanical dynamics. The expression of PKCβ and PKCγ induced by Wnta1 was completely abolished by the PLC inhibitor. Wnta1-induced elevation of TAK1 was blocked by an inhibitor of PKC. In addition, an inhibitor of TAK1 entirely abrogated the elevation of ATF2, TCF3, TCF4, and LEF1 protein levels induced by Wnta1, which was accompanied by inhibition of ANP secretion and mechanical dynamics. The results suggest that Wnta1 promoted ANP secretion and positive inotropy mainly via the WNT/Ca2+ signaling pathway in isolated beating rat atria (Fig. 11).
In the WNT/Ca2+ signaling pathway, WNT binding to its frizzled receptor induces activation of PLC leading to the accumulation of intracellular Ca2+ and subsequent activation of CaMKII, calcineurin, and PKC [9,13]. In addition, it has been reported that DVL-1 overexpression in mice induced by Dvl-1 transgenesis upregulated ANP expression in cardiomyocytes, and this overexpression was abolished in Camk2δγ double-knockout mice [20]. In the present study, Wnta1 significantly enhanced the mechanical dynamics, upregulated expression of PKCβ and PKCγ, and increased ANP secretion in isolated beating rat atria. The Wnta1-induced secretion of ANP and atrial mechanical dynamics were completely blocked by inhibition of PLC and PKC. These results indicate that Wnta1 promoted ANP secretion and a positive inotropic effect in atrial mechanical dynamics mainly via the WNT/Ca2+ pathway. The mechanism involved in Wnta1 activation of PKC in the present study is in line with previous studies [9,13] and consistent with investigations showing that activated PKC was effective in promoting the secretion of ANP [21,22]. Our data also support studies reported upregulation of ANP expression after activation of WNT in myocardial hypertrophic animal models [13,20].
In this study, Wnta1 upregulated the expression of TAB1 and the levels of phosphor-TAK1 and phosphor-ATF2, and which were eliminated by inhibiting PLC and PKC. TAK1 inhibition also completely abolished the Wnta1-induced upregulation of phosphor-ATF2 and the increase in atrial ANP secretion and mechanical dynamics. TAK1 is a member of the mitogen-activated protein three kinases (MAP3Ks) and is also known as MAP3K7. It has been shown that TAK1 regulates transforming growth factor-β (TGF-β) signaling by phosphorylating SMAD transcription factors and mediates the non-canonical WNT signaling process [23]. TAK1 activation requires TAK1-binding proteins (TAB1–3) and the TAK1-TAB1 interaction occurs in the kinase domain of TAK1 [24]. In addition, TAK1 activation induced by TGF-β1 resulted in activation of ATF2 in a PKC-dependent manner in cultured neonatal rat cardiomyocytes and ultimately upregulated the expression of ANP [19]. The data from the present study support these results. Recently, it has been demonstrated that TAK1 activates Toll-like receptor 9 (TLR9), which results in the direct inhibition of sarcoplasmic reticulum/ERCa2+-ATPase 2 [25-27]. In our study, the positive inotropic effect of Wnta1 on atrial mechanical dynamics was also completely eliminated by an inhibitor of TAK1. Hence, it may be possible that TLR9 activation may correlate with the action of Wnta1 on atrial dynamics, although the detailed mechanism remains to be further defined.
TCF/LEF family members act as the main downstream effectors of WNT signaling, and it has been demonstrated that, in addition to β-catenin, members of the ATF family can also activate TCF-driven transcription [28]. Moreover, it has been shown that activated LEF1 in cultured neonatal rat cardiomyocytes can directly bind to the consensus binding sequence on the Anp gene promoter to enhance transcription [16]. Our study also showed that Wnta1 treatment significantly upregulated the expression of ATF2 with concomitant increases in TCF3, TCF4, and LEF1 protein levels. Upregulation of these proteins was blocked by inhibition of TAK1, which prevented Wnta1-augmented secretion of ANP. Our results suggest that Wnta1 activated TAK1 leading to the activation of ATF2, TCF3/LEF1, and TCF4/LEF1, thereby promoting the secretion of atrial ANP. The data from our study support the aforementioned previous studies. Due to the roles of abnormal activation of WNT signaling pathways on myocardial ischemic/hypoxic injury, hypertrophy, fibrosis, arrhythmia, myocardial infarction, and heart failure [9,12-15], while ANP can prevents the binding of WNT to frizzled receptor to inhibiting WNT signaling [7], increase in ANP secretion under abnormal activation of WNT signaling is a functional manifestation of resistance to abnormal Wnt signaling.
In summary, Wnta1 promotes secretion of ANP and a positive inotropic effect on mechanical dynamics by activating ATF2, TCF3/LEF1, and TCF4/LEF1 mainly via the WNT/Ca2+ signaling pathway in isolated beating rat atria. This suggests that increase in ANP secretion under Wnt1 action is a functional manifestation of maintaining homeostasis.
Notes
REFERENCES
1. Kim HY, Cho KW, Xu DY, Kang DG, Lee HS. 2013; Endogenous ACh tonically stimulates ANP secretion in rat atria. Am J Physiol Heart Circ Physiol. 305:H1050–H1056. DOI: 10.1152/ajpheart.00469.2013. PMID: 23913708. PMID: https://www.scopus.com/inward/record.uri?partnerID=HzOxMe3b&scp=84884938581&origin=inward.


2. Kiemer AK, Vollmar AM. 2001; The atrial natriuretic peptide regulates the production of inflammatory mediators in macrophages. Ann Rheum Dis. 60(Suppl 3):iii68–iii70. DOI: 10.1136/ard.60.90003.iii68. PMID: 11890659. PMCID: PMC1766678.
3. De Vito P, Incerpi S, Pedersen JZ, Luly P. 2010; Atrial natriuretic peptide and oxidative stress. Peptides. 31:1412–1419. DOI: 10.1016/j.peptides.2010.04.001. PMID: 20385186. PMID: https://www.scopus.com/inward/record.uri?partnerID=HzOxMe3b&scp=77953124862&origin=inward.


4. Hong L, Xi J, Zhang Y, Tian W, Xu J, Cui X, Xu Z. 2012; Atrial natriuretic peptide prevents the mitochondrial permeability transition pore opening by inactivating glycogen synthase kinase 3β via PKG and PI3K in cardiac H9c2 cells. Eur J Pharmacol. 695:13–19. DOI: 10.1016/j.ejphar.2012.07.053. PMID: 22975711. PMID: https://www.scopus.com/inward/record.uri?partnerID=HzOxMe3b&scp=84867571658&origin=inward.


5. Lugnier C, Meyer A, Charloux A, Andrès E, Gény B, Talha S. 2019; The endocrine function of the heart: physiology and involvements of natriuretic peptides and cyclic nucleotide phosphodiesterases in heart failure. J Clin Med. 8:1746. DOI: 10.3390/jcm8101746. PMID: 31640161. PMCID: PMC6832599. PMID: https://www.scopus.com/inward/record.uri?partnerID=HzOxMe3b&scp=85083845505&origin=inward.


6. Mezzasoma L, Peirce MJ, Minelli A, Bellezza I. 2017; Natriuretic peptides: the case of prostate cancer. Molecules. 22:1680. DOI: 10.3390/molecules22101680. PMID: 28994721. PMCID: PMC6151559. PMID: https://www.scopus.com/inward/record.uri?partnerID=HzOxMe3b&scp=85032930690&origin=inward.


7. Serafino A, Pierimarchi P. 2014; Atrial natriuretic peptide: a magic bullet for cancer therapy targeting Wnt signaling and cellular pH regulators. Curr Med Chem. 21:2401–2409. DOI: 10.2174/0929867321666140205140152. PMID: 24524761. PMCID: PMC4063317. PMID: https://www.scopus.com/inward/record.uri?partnerID=HzOxMe3b&scp=84901939934&origin=inward.


8. Zhang J, Zhao Z, Wang J. 2014; Natriuretic peptide receptor A as a novel target for cancer. World J Surg Oncol. 12:174. DOI: 10.1186/1477-7819-12-174. PMID: 24894887. PMCID: PMC4049422. PMID: https://www.scopus.com/inward/record.uri?partnerID=HzOxMe3b&scp=84901981985&origin=inward.


9. Foulquier S, Daskalopoulos EP, Lluri G, Hermans KCM, Deb A, Blankesteijn WM. 2018; WNT signaling in cardiac and vascular disease. Pharmacol Rev. 70:68–141. DOI: 10.1124/pr.117.013896. PMID: 29247129. PMCID: PMC6040091. PMID: https://www.scopus.com/inward/record.uri?partnerID=HzOxMe3b&scp=85040003896&origin=inward.


10. Moon RT, Kohn AD, De Ferrari GV, Kaykas A. 2004; WNT and beta-catenin signalling: diseases and therapies. Nat Rev Genet. 5:691–701. DOI: 10.1038/nrg1427. PMID: 15372092. PMID: https://www.scopus.com/inward/record.uri?partnerID=HzOxMe3b&scp=4344584730&origin=inward.
11. Ng LF, Kaur P, Bunnag N, Suresh J, Sung ICH, Tan QH, Gruber J, Tolwinski NS. 2019; WNT signaling in disease. Cells. 8:826. DOI: 10.3390/cells8080826. PMID: 31382613. PMCID: PMC6721652. PMID: https://www.scopus.com/inward/record.uri?partnerID=HzOxMe3b&scp=85074564426&origin=inward.


12. Hu HH, Cao G, Wu XQ, Vaziri ND, Zhao YY. 2020; Wnt signaling pathway in aging-related tissue fibrosis and therapies. Ageing Res Rev. 60:101063. DOI: 10.1016/j.arr.2020.101063. PMID: 32272170. PMID: https://www.scopus.com/inward/record.uri?partnerID=HzOxMe3b&scp=85083282776&origin=inward.


13. Fu WB, Wang WE, Zeng CY. 2019; Wnt signaling pathways in myocardial infarction and the therapeutic effects of Wnt pathway inhibitors. Acta Pharmacol Sin. 40:9–12. DOI: 10.1038/s41401-018-0060-4. PMID: 30002488. PMCID: PMC6318317. PMID: https://www.scopus.com/inward/record.uri?partnerID=HzOxMe3b&scp=85049790426&origin=inward.


14. Stylianidis V, Hermans KCM, Blankesteijn WM. 2017; Wnt signaling in cardiac remodeling and heart failure. Handb Exp Pharmacol. 243:371–393. DOI: 10.1007/164_2016_56. PMID: 27838851. PMID: https://www.scopus.com/inward/record.uri?partnerID=HzOxMe3b&scp=85020690054&origin=inward.


15. Katoh M. 2018; Multi-layered prevention and treatment of chronic inflammation, organ fibrosis and cancer associated with canonical WNT/β-catenin signaling activation (Review). Int J Mol Med. 42:713–725. DOI: 10.3892/ijmm.2018.3689. PMID: 29786110. PMCID: PMC6034925. PMID: https://www.scopus.com/inward/record.uri?partnerID=HzOxMe3b&scp=85055010694&origin=inward.


16. Zhang CG, Jia ZQ, Li BH, Zhang H, Liu YN, Chen P, Ma KT, Zhou CY. 2009; Beta-catenin/TCF/LEF1 can directly regulate phenylephrine-induced cell hypertrophy and Anf transcription in cardiomyocytes. Biochem Biophys Res Commun. 390:258–262. DOI: 10.1016/j.bbrc.2009.09.101. PMID: 19799869. PMID: https://www.scopus.com/inward/record.uri?partnerID=HzOxMe3b&scp=70350125871&origin=inward.


17. Zhang B, Cui X, Jin HH, Hong L, Liu X, Li X, Zhang QG, Liu LP. 2017; Ginsenoside Re prevents angiotensin II-induced gap-junction remodeling by activation of PPARγ in isolated beating rat atria. Life Sci. 190:36–45. DOI: 10.1016/j.lfs.2017.09.027. PMID: 28962867. PMID: https://www.scopus.com/inward/record.uri?partnerID=HzOxMe3b&scp=85030156838&origin=inward.


18. Liu LP, Hong L, Yu L, Li HY, Ding DZ, Jin SJ, Cui X. 2012; Ouabain stimulates atrial natriuretic peptide secretion via the endothelin-1/ET(B) receptor-mediated pathway in beating rabbit atria. Life Sci. 90:793–798. DOI: 10.1016/j.lfs.2012.04.008. PMID: 22521291. PMID: https://www.scopus.com/inward/record.uri?partnerID=HzOxMe3b&scp=84860716459&origin=inward.


19. Lim JY, Park SJ, Hwang HY, Park EJ, Nam JH, Kim J, Park SI. 2005; TGF-beta1 induces cardiac hypertrophic responses via PKC-dependent ATF-2 activation. J Mol Cell Cardiol. 39:627–636. DOI: 10.1016/j.yjmcc.2005.06.016. PMID: 16125722. PMID: https://www.scopus.com/inward/record.uri?partnerID=HzOxMe3b&scp=24944542831&origin=inward.


20. Zhang M, Hagenmueller M, Riffel JH, Kreusser MM, Bernhold E, Fan J, Katus HA, Backs J, Hardt SE. 2015; Calcium/calmodulin-dependent protein kinase II couples Wnt signaling with histone deacetylase 4 and mediates dishevelled-induced cardiomyopathy. Hypertension. 65:335–344. DOI: 10.1161/HYPERTENSIONAHA.114.04467. PMID: 25489064. PMID: https://www.scopus.com/inward/record.uri?partnerID=HzOxMe3b&scp=84921539288&origin=inward.


21. Bae IS, Kim SH. 2019; Expression and secretion of an atrial natriuretic peptide in beige-like 3T3-L1 adipocytes. Int J Mol Sci. 20:6128. DOI: 10.3390/ijms20246128. PMID: 31817347. PMCID: PMC6940835. PMID: https://www.scopus.com/inward/record.uri?partnerID=HzOxMe3b&scp=85076305798&origin=inward.


22. Liu X, Zhang Y, Hong L, Han CJ, Zhang B, Zhou S, Wu CZ, Liu LP, Cui X. 2017; Gallic acid increases atrial natriuretic peptide secretion and mechanical dynamics through activation of PKC. Life Sci. 181:45–52. DOI: 10.1016/j.lfs.2017.05.024. PMID: 28535942. PMID: https://www.scopus.com/inward/record.uri?partnerID=HzOxMe3b&scp=85020054586&origin=inward.


23. Mukhopadhyay H, Lee NY. 2020; Multifaceted roles of TAK1 signaling in cancer. Oncogene. 39:1402–1413. DOI: 10.1038/s41388-019-1088-8. PMID: 31695153. PMCID: PMC7023988. PMID: https://www.scopus.com/inward/record.uri?partnerID=HzOxMe3b&scp=85074801913&origin=inward.


24. Shibuya H, Yamaguchi K, Shirakabe K, Tonegawa A, Gotoh Y, Ueno N, Irie K, Nishida E, Matsumoto K. 1996; TAB1: an activator of the TAK1 MAPKKK in TGF-beta signal transduction. Science. 24:1179–1182. DOI: 10.1126/science.272.5265.1179. PMID: 8638164. PMID: https://www.scopus.com/inward/record.uri?partnerID=HzOxMe3b&scp=0029940355&origin=inward.
25. Shintani Y, Drexler HC, Kioka H, Terracciano CM, Coppen SR, Imamura H, Akao M, Nakai J, Wheeler AP, Higo S, Nakayama H, Takashima S, Yashiro K, Suzuki K. 2014; Toll-like receptor 9 protects non-immune cells from stress by modulating mitochondrial ATP synthesis through the inhibition of SERCA2. EMBO Rep. 15:438–445. DOI: 10.1002/embr.201337945. PMID: 24610369. PMCID: PMC3989675. PMID: https://www.scopus.com/inward/record.uri?partnerID=HzOxMe3b&scp=84898605890&origin=inward.


26. Shintani Y, Kapoor A, Kaneko M, Smolenski RT, D'Acquisto F, Coppen SR, Harada-Shoji N, Lee HJ, Thiemermann C, Takashima S, Yashiro K, Suzuki K. 2013; TLR9 mediates cellular protection by modulating energy metabolism in cardiomyocytes and neurons. Proc Natl Acad Sci U S A. 110:5109–5114. DOI: 10.1073/pnas.1219243110. PMID: 23479602. PMCID: PMC3612600. PMID: https://www.scopus.com/inward/record.uri?partnerID=HzOxMe3b&scp=84875518435&origin=inward.


27. McCarthy CG, Wenceslau CF, Ogbi S, Szasz T, Webb RC. 2018; Toll-like receptor 9-dependent AMPKα activation occurs via TAK1 and contributes to RhoA/ROCK signaling and actin polymerization in vascular smooth muscle cells. J Pharmacol Exp Ther. 365:60–71. DOI: 10.1124/jpet.117.245746. PMID: 29348267. PMCID: PMC5830639. PMID: https://www.scopus.com/inward/record.uri?partnerID=HzOxMe3b&scp=85043282975&origin=inward.


28. Grumolato L, Liu G, Haremaki T, Mungamuri SK, Mong P, Akiri G, Lopez-Bergami P, Arita A, Anouar Y, Mlodzik M, Ronai ZA, Brody J, Weinstein DC, Aaronson SA. 2013; β-Catenin-independent activation of TCF1/LEF1 in human hematopoietic tumor cells through interaction with ATF2 transcription factors. PLoS Genet. 9:e1003603. DOI: 10.1371/journal.pgen.1003603. PMID: 23966864. PMCID: PMC3744423. PMID: https://www.scopus.com/inward/record.uri?partnerID=HzOxMe3b&scp=84884631810&origin=inward.


Fig. 1
Effects of Wnt agonist 1 on atrial atrial natriuretic peptide (ANP) secretion (A) and dynamics (B) in beating rat atria.
Data were expressed as means ± SE. n = 6. Cont, control; Wnta1, Wnt agonist 1; the collection time for each fraction is 2 min. *p < 0.05 vs. control.
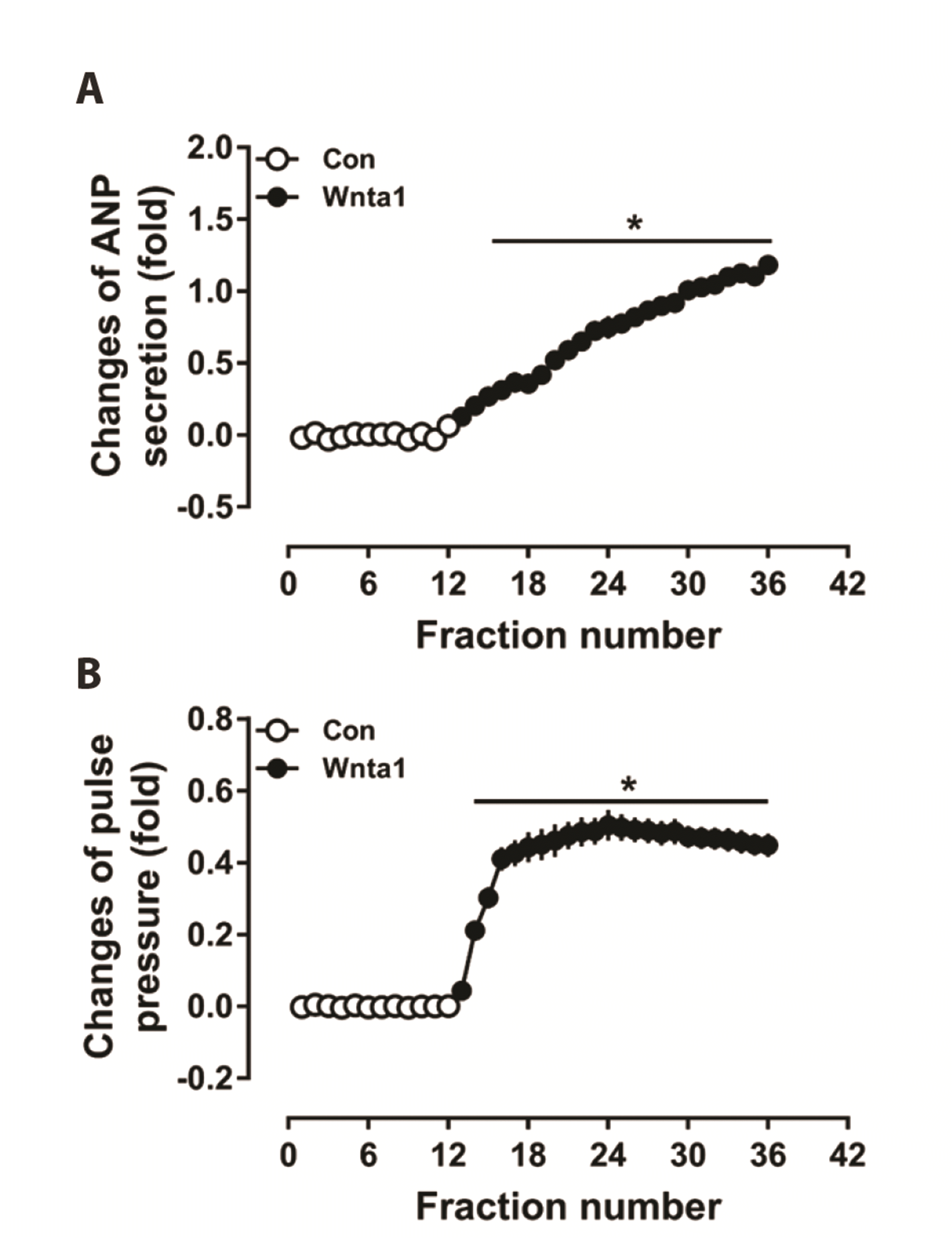
Fig. 2
Effects of phospholipase C (PLC) antagonist on Wnt agonist 1-induced atrial natriuretic peptide (ANP) secretion (A) and dynamics (B) in beating rat atria.
Data were expressed as means ± SE. n = 6. Cont, control; Wnta1, Wnt agonist 1; U, U73122; the collection time for each fraction is 2 min. *p < 0.05 vs. control, #p < 0.05 vs. Wnta1.
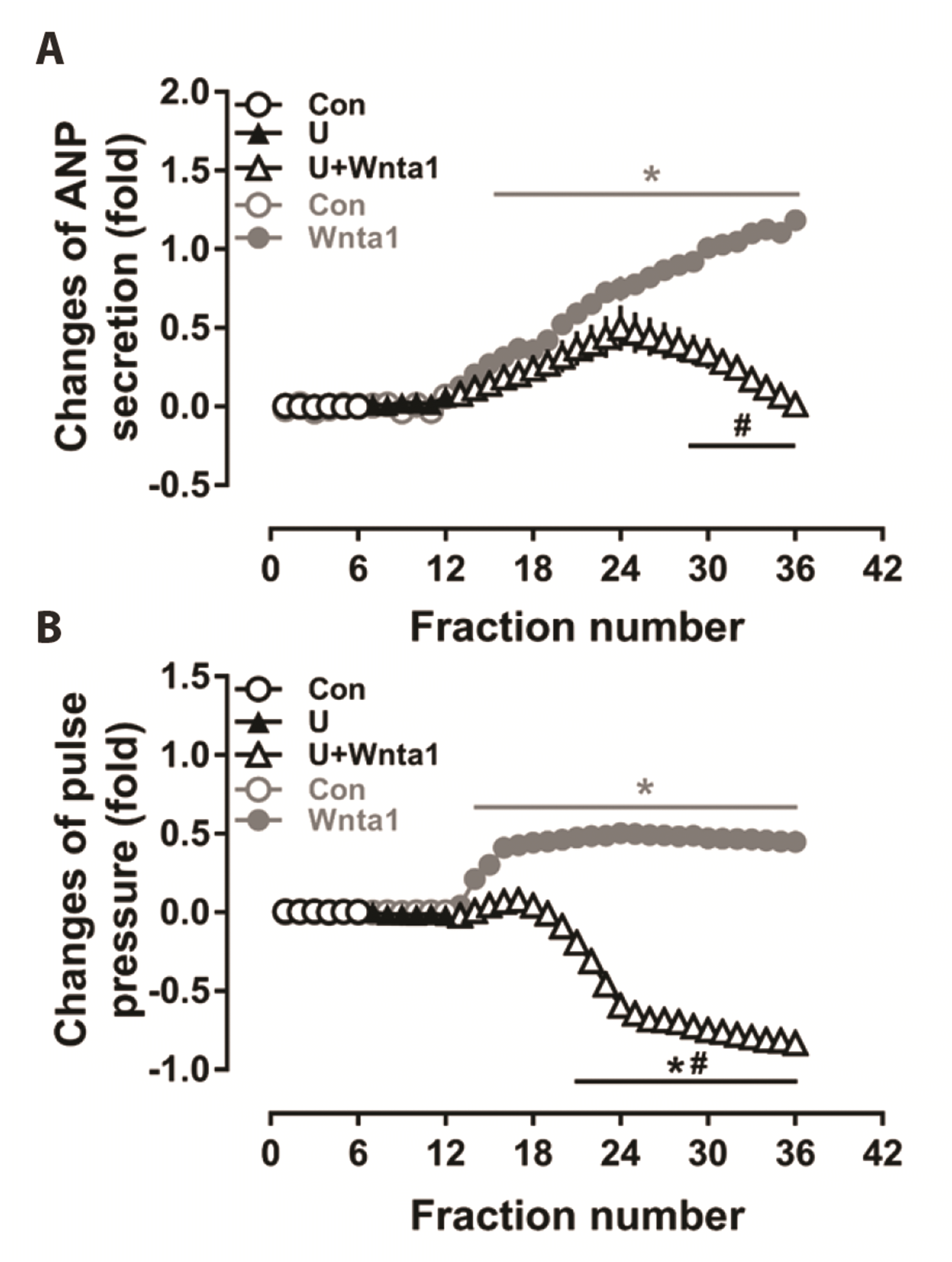
Fig. 3
Effects of Wnt agonist 1 on protein kinase C (PKC)β (A) and PKCγ (B) expression in beating rat atria.
Data were expressed as means ± SE. n = 5. Cont, control; Wnta1, Wnt agonist 1; U, U73122. *p < 0.05 vs. control, #p < 0.05 vs. Wnta1.
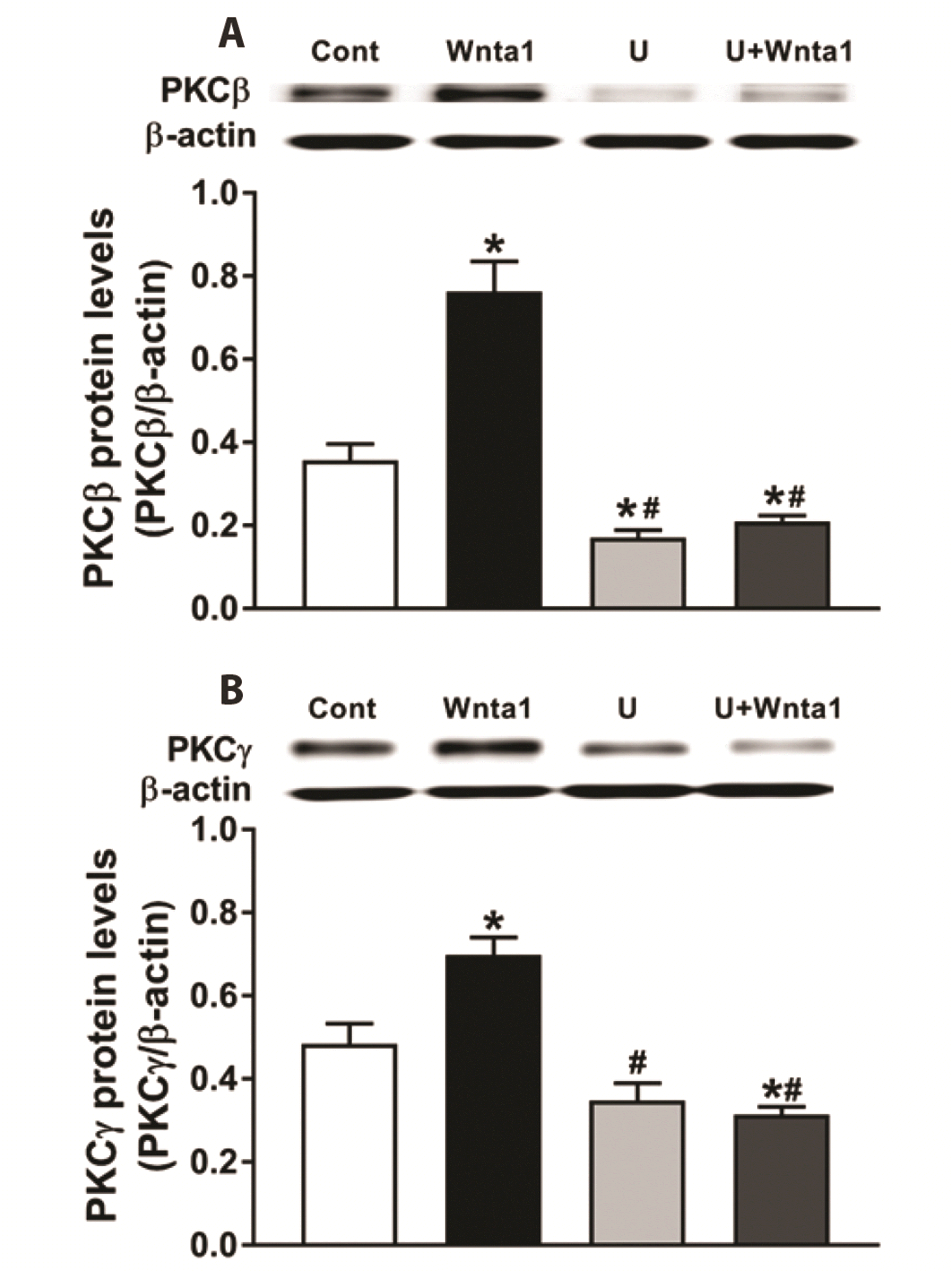
Fig. 4
Effects of protein kinase C (PKC) antagonist on Wnt agonist 1-induced atrial natriuretic peptide (ANP) secretion (A) and dynamics (B) in beating rat atria.
Data were expressed as means ± SE. n = 6. Cont, control; Wnta1, Wnt agonist 1; Go, Gö6983, an antagonist of PKC; the collection time for each fraction is 2 min. *p < 0.05 vs. control, #p < 0.05 vs. Wnta1.
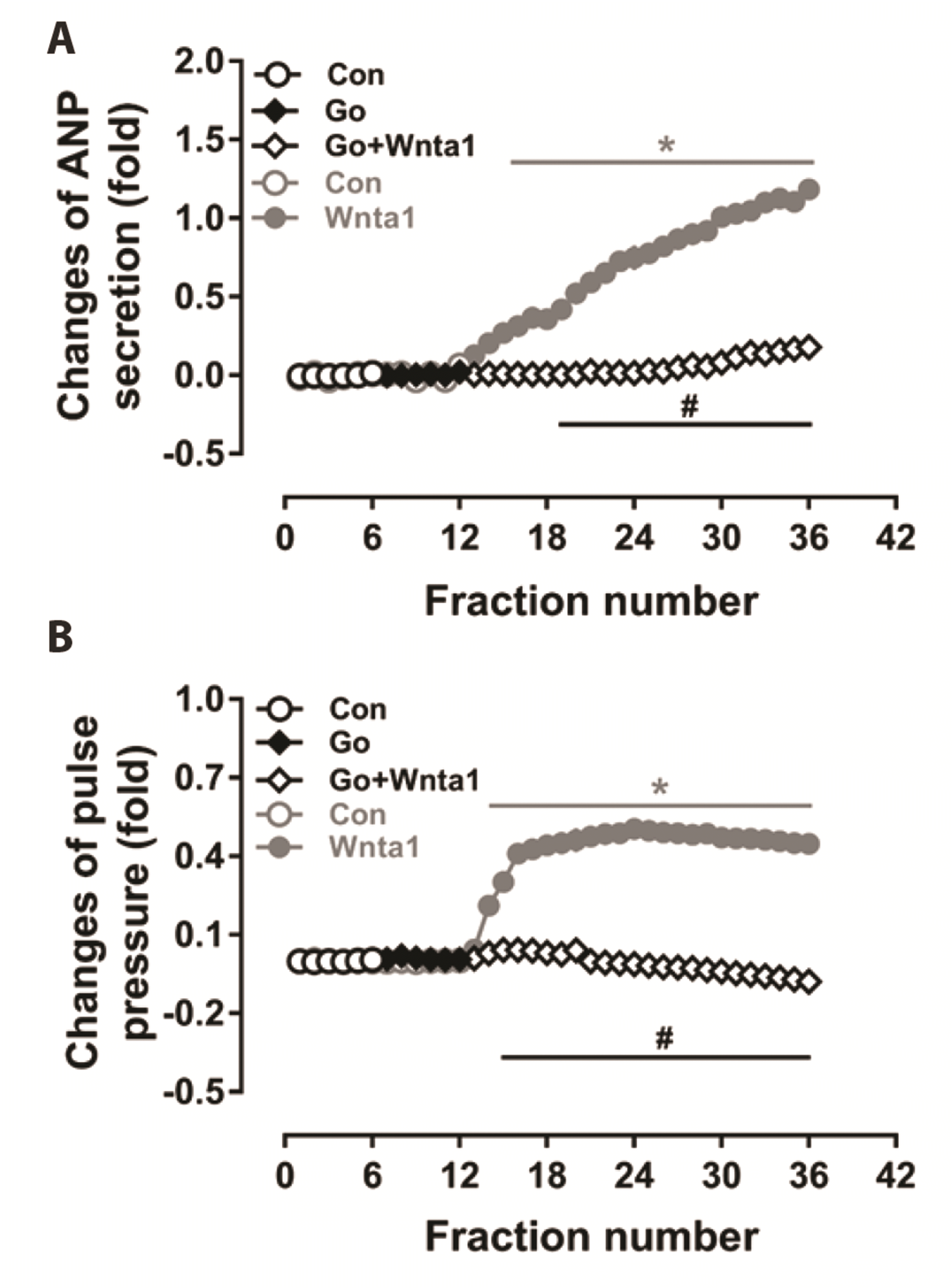
Fig. 5
Effects of phospholipase C (PLC) (A) and protein kinase C (PKC) (B) antagonists on Wnt agonist 1-induced TAK1 banding 1 (TAB1) expression in beating rat atria.
Data were expressed as means ± SE. n = 5. Cont, control; Wnta1, Wnt agonist 1; U, U73122; Go, Gö6983. *p < 0.05 vs. control, #p < 0.05 vs. Wnta1.
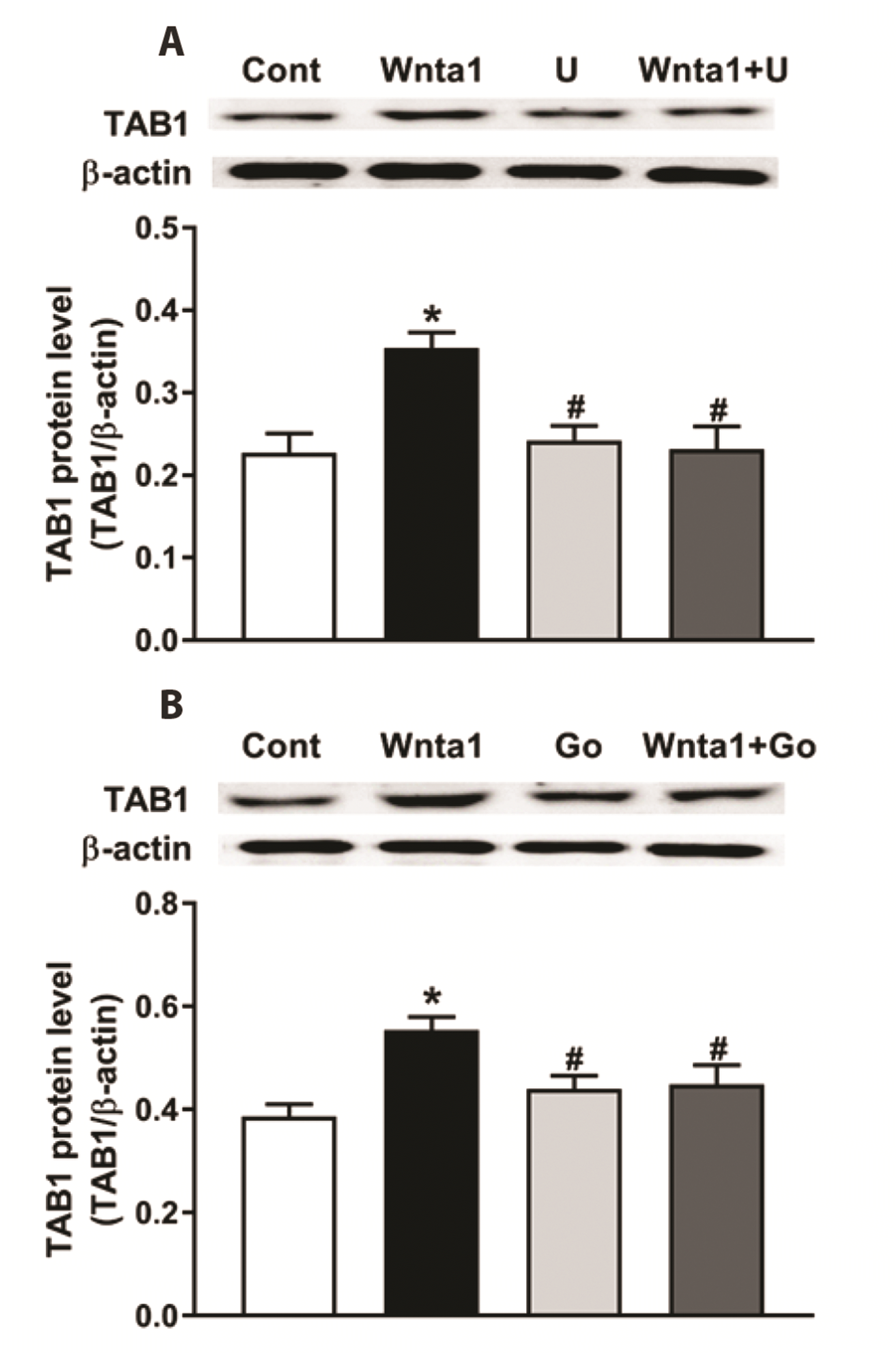
Fig. 6
Effects of phospholipase C (PLC) (A, B) and protein kinase C (PKC) (C, D) antagonists on Wnt agonist 1-induced TAK1 expression in beating rat atria.
Data were expressed as means ± SE. n = 5. Cont, control; Wnta1, Wnt agonist 1; U, U73122; Go, Gö6983; p-TAK1, phospho-TAK1; t-TAK1, total-TAK1. *p < 0.05 vs. control, #p < 0.05 vs. Wnta1.
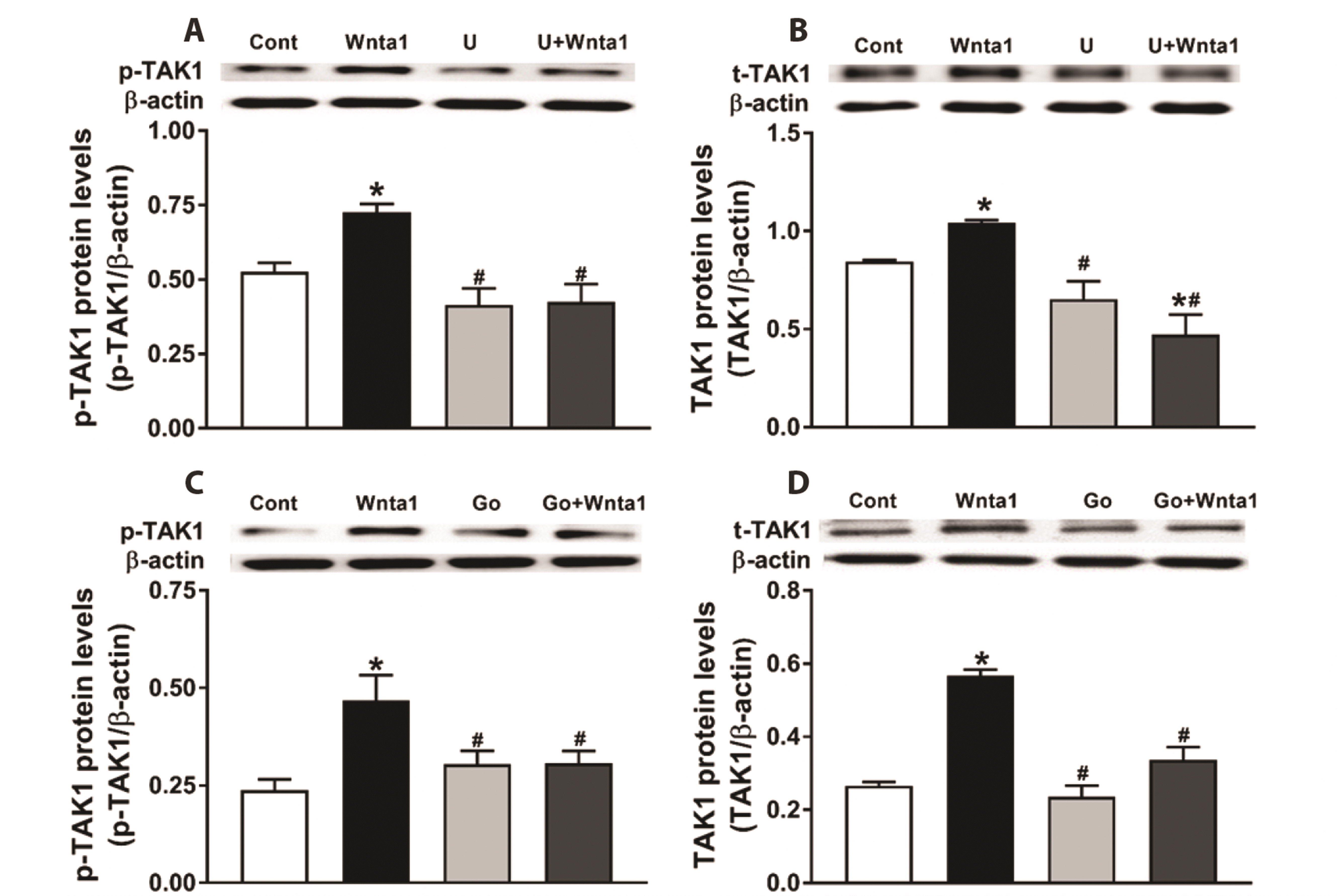
Fig. 7
Effects of phospholipase C (PLC) (A), protein kinase C (PKC) (B) and TAK1 (C) antagonists on Wnt agonist 1-induced ATF2 expression in beating rat atria.
Data were expressed as means ± SE. n = 5. Cont, control; Wnta1, Wnt agonist 1; U, U73122; Go, Gö6983, TI, TAK1 inhibitor, an inhibitor of TAK1; p-ATF2, phospho-ATF2; t-ATF2, total-ATF2. *p < 0.05 vs. control, #p < 0.05 vs. Wnta1.
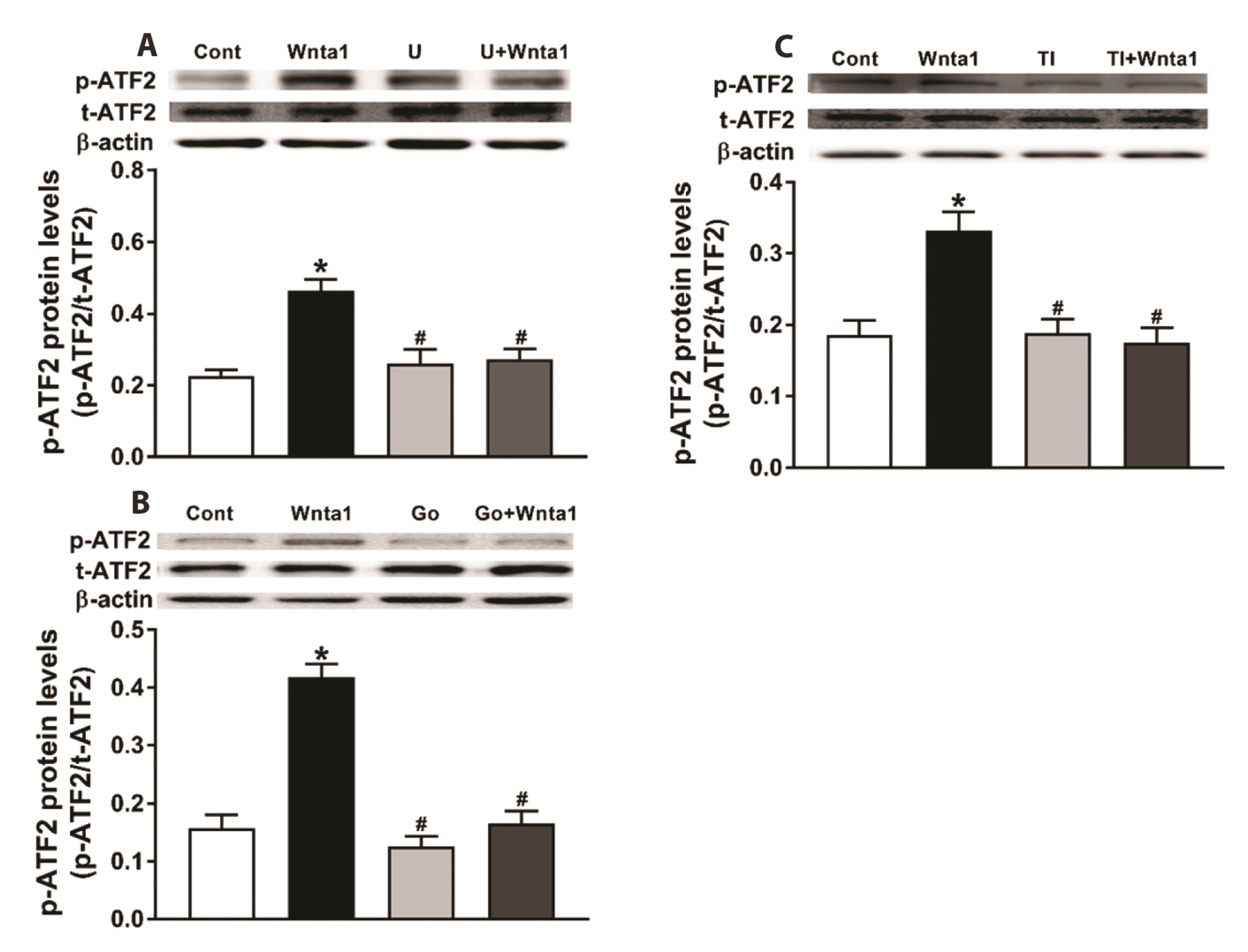
Fig. 8
Effects of phospholipase C (PLC), protein kinase C (PKC) and TAK1 antagonists on Wnt agonist 1-induced T cell factor (TCF)3 (A–C) and TCF4 (D–F) expression in beating rat atria.
Data were expressed as means ± SE. n = 5. Cont, control; Wnta1, Wnt agonist 1; U, U73122; Go, Gö6983, TI, TAK1 inhibitor. *p < 0.05 vs. control, #p < 0.05 vs. Wnta1.

Fig. 9
Effects of phospholipase C (PLC) (A), protein kinase C (PKC) (B) and TAK1 (C) antagonists on Wnt agonist 1-induced lymphoid enhancer factor 1 (LEF1) expression in beating rat atria.
Data were expressed as means ± SE. n = 5. Cont, control; Wnta1, Wnt agonist 1; U, U73122; Go, Gö6983, TI, TAK1 inhibitor. *p < 0.05 vs. control, #p < 0.05 vs. Wnta1.
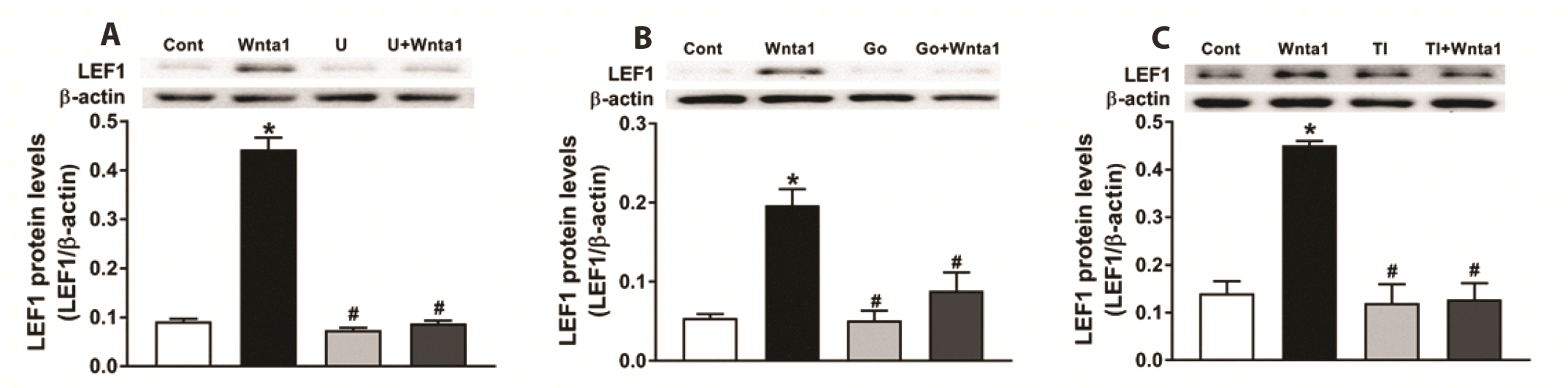