Abstract
It has been demonstrated that APPL1 (adaptor protein, phosphotyrosine interacting with PH domain and leucine zipper 1) is involved in the regulation of several growth-related signaling pathways and thus closely associated with the development and progression of some cancers. Diallyl trisulfide (DAT), a garlic-derived bioactive compound, exerts selective cytotoxicity to various human cancer cells through interfering with pro-survival signaling pathways. However, whether and how DAT affects survival of human hepatocellular carcinoma (HCC) cells remain unclear. Herein, we tested the hypothesis of the involvement of APPL1 in DAT-induced cytotoxicity in HCC HepG2 cells. We found that Lys 63 (K63)-linked polyubiquitination of APPL1 was significantly decreased whereas phosphorylation of APPL1 at serine residues remained unchanged in DAT-treated HepG2 cells. Compared with wild-type APPL1, overexpression of APPL1 K63R mutant dramatically increased cell apoptosis and mitigated cell survival, along with a reduction of phosphorylation of STAT3, Akt, and Erk1/2. In addition, DAT administration markedly reduced protein levels of intracellular TNF receptor-associated factor 6 (TRAF6). Genetic inhibition of TRAF6 decreased K63-linked polyubiquitination of APPL1. Moreover, the cytotoxicity impacts of DAT on HepG2 cells were greatly attenuated by overexpression of wild-type APPL1. Taken together, these results suggest that APPL1 polyubiquitination probably mediates the inhibitory effects of DAT on survival of HepG2 cells by modulating STAT3, Akt, and Erk1/2 pathways.
Hepatocellular carcinoma (HCC) ranks as the fifth most common tumor and the third most common cause of death from cancers in the world. The incidence and mortality of HCC have remained largely stable in Asian countries in past two decades and increased in Europe and United States in the last years [1,2]. Currently, surgical resection and liver transplantation are the best treatment option for the patients with HCC, but can only be applied to about 15% of the patients [3-5]. Despite the non-surgical treatment has made some progress in recent years, the median survival time of the patients with unresectable and metastatic HCC remains still only a few months. Therefore, screening novel medications from traditional herbs or foods to prevent HCC development and progression will undoubtedly provide creative therapy strategies for non-surgical treatment of HCC.
Diallyl trisulfide (DAT) is a major constituent of oil-soluble sulfur compounds derived from garlic (Allium sativum L. family Liliaceae), a well-known traditional medicine for a long time [6]. A growing number of studies have demonstrated that DAT possesses multiple pharmacological functions. DAT has been found to ameliorate myocardial ischemia-reperfusion injury and carbon tetrachloride-caused liver damage [7,8], protect cardiac myocyte against high glucose-induced apoptosis [9], augment ischemia-induced angiogenesis [10], attenuate ethanol-induced hepatic steatosis and n-hexane-induced neurotoxicity [11,12], and suppress the adipogenesis of 3T3-L1 preadipocyte [13], through its inhibitory effects on oxidative stress, endoplasmic reticulum stress [7,10], inflammatory responses [14], and so on. Recently, accumulating studies have shown its impacts on carcinomas or cancer cell lines. DAT can effectively promote apoptosis of cancer cells and attenuate growth and metastasis of tumors, including breast cancer [15], lung carcinoma [16], gastric cancer [17], colon tumor [18,19], prostate cancer [20,21], osteosarcoma [22]and etc. DAT has also exhibited significant anti-tumor effect in orthotopic murine HCC model by suppressing cell proliferation and inducing cell apoptosis [23]. However, the molecular mechanisms underlying DAT action are largely ill-defined.
APPL1 (adaptor protein containing PH domain, PTB domain and leucine zipper motif 1) is a multifunctional adaptor protein, which has been shown to regulate cell proliferation, adiponectin signal, and insulin sensitivity through actively participating in various stages of signaling pathways [24,25]. Recent studies have confirmed that APPL1 protein is highly expressed in some types of tumor tissues such as breast cancer, HCC, and invasive prostate cancer [26-28]. Knockdown of APPL1 protein inhibits TGFβ-induced invasion of human prostate cancer PC-3U cells and breast cancer MDA-MB-231 cells [27], and significantly enhances the sensitivity of pancreatic cancer cells to radiation therapy [29]. Our previous study has also evidenced that APPL1 promotes leptin-induced cell proliferation and migration in HCC HepG2 cells and breast cancer MCF-7 cells [28]. These findings strongly suggest that APPL1 has a positive effect on the growth and metastasis of certain tumors under a variety of pathophysiological conditions, but the detailed mechanism still remains largely unresolved.
The present study aims to observe the potential impacts of DAT on survival of HepG2 cells and to elucidate its underlying mechanism, particularly the possible novel role of APPL1 in mediating DAT action. We experimentally evidenced that the reduction of TNF receptor associated factor 6 (TRAF6)-medicated Lys 63 (K63)-linked polyubiquitination of APPL1 is responsible for the cytotoxicity of DAT on HepG2 cells.
The antibodies used in this study include: anti-APPL1 (#3858), anti-STAT3 (#9139), anti-phospho-STAT3 Try705 (#9145), anti-Akt (#4691), anti-phospho-Akt Ser473 (#9271), anti-Erk (#4695), and anti-phospho-Erk Thr202/Tyr204 (#4370) antibodies, Cell Signaling Technology (Beverly, MA, USA); anti-phospho -(Ser/Thr) (ab17464), anti-TRAF6 (ab94720), anti-ubiquitin (linkage-specific K63) (ab179434), and anti-ubiquitin (linkage-specific K48) (ab190061) antibodies, Abcam Inc. (Cambridge, MA, USA). Diallyl trisulfide (DAT, SMB00289) and 3-(4,5-dimethylthiazol-2-yl)-2,5-diphenyltetrazolium bromide (MTT, M2128) were from Sigma-Aldrich (St. Louis, MO, USA). Protein A-Sepharose beads was purchased from Amersham-Pharmacia Biotech (Piscataway, NJ, USA).
Human hepatocellular carcinoma HepG2 cells (HB-8065) and normal murine hepatocyte AML12 (alpha mouse liver 12) cells (CRL-2254) were purchased from American Type Culture Collection (ATCC, Rockville, MD, USA). HepG2 cells were routinely cultured in Dulbecco’s Modified Eagle Medium (DMEM) supplemented with 10% fetal bovine serum (FBS) and 1% penicillin/streptomycin. AML12 cells were grown in a mixture of DMEM and Ham's F12 medium supplemented with 0.005 mg/ml insulin, 0.005 mg/ml transferrin, 5 ng/ml selenium, 40 ng/ml dexamethasone, and 10% FBS. All cells were incubated in humidified atmosphere containing 5% CO2 and 95% air at 37°C.
Human APPL1-encoding plasmid (pcDNA3/APPL1), adenovirus encoding human full length APPL1, and adenovirus encoding β-galactosidase (β-gal) were gift from Drs. Feng Liu and Lily Q. Dong (University of Texas Health Science Center at San Antonio, San Antonio, TX, USA). K63R mutation of APPL1 was generated by single-stranded site-directed mutagenesis by standard molecular cloning techniques [30], using the following oligonucleotides: forward 5’-CAACACACCTGACCTCAAGGCTTTTAAAAGAATATG-3’ and reverse 5’-CATATTCTTTTAAAAGCCTTGAGGTCAGGTGTGTTG-3’ [31]. Adenovirus encoding APPL1 K63R mutant was generated as perilously described [32]. Recombinant adenoviruses were packaged and amplified in HEK-293T cells, followed by purification using affinity column chromatography.
For virus infection, cells were incubated in serum-free medium containing adenoviruses (MOI:50) for 6 h, and then grown in complete medium for another 36 h. After 42 h of infection, the cells were pending for experimental study. Adenovirus carrying β-gal served as a negative control.
SiRNA transfection was performed as our previous described [33]. SiRNA sequences used to silence expressions of human TRAF6 (NM_145803) were as following: 5’-CATCAGAGAACAGATGCCTAATCAT-3’, and scrambled control 5’-CATGAGCAAAGACGTATCTACACAT-3’. The knockdown efficiency was assessed by western blot.
Cell viabilities were determined by MTT assay as our previously described [28,34,35]. Briefly, 5 × 103 cells/well were grown in DMEM with 10% FBS. After treatment with different concentration of DAT for the desired time, the medium was replaced by 100 μl of fresh DMEM (no phenol red) with 10 µl of the 12 mM MTT stock solution. And the cells were incubated at 37°C for 3 h. The medium was then carefully removed and 150 µl of MTT solvent (containing 4 mM HCl, 0.1% Nonidet P-40, all in isopropanol) was added into each well. The plate was covered with tinfoil and agitated on an orbital shaker for 20 min. An ELISA (enzyme-linked immunosorbent assay) plate reader (BioTek, Winooski, VT, USA) was used to determine absorbance at 590 nm with a reference filter of 620 nm.
Cell proliferation was evaluated by a BrdU Cell Proliferation Assay Kit (ab287841; Abcam) according to the protocol provided by the manufacturers.
Apoptosis was induced by serum deprivation for 60 h [36]. The cells were fixed with 3.8% paraformaldehyde for 20 min, and then stained with the terminal deoxyribonucleotidyl transferase (TdT)-mediated dUTP-digoxigenin nick end labeling (TUNEL) reagent (Roche, Basel, Switzerland) for in situ apoptosis detection, according to the manufacturer’s instruction. Positive and negative controls were pretreated with 10 U/ml DNase or incubated without TdT, respectively. Apoptotic cells were detected under an Olympus FluoView FV1000 Confocal Microscope (Olympus, Tokyo, Japan).
Western blot and immunoprecipitation were carried out as our previously described [28,34,35]. Briefly, cell lysate was extracted with lysis buffer (containing 50 mM HEPES, pH 7.6, 150 mM NaCl, 1% Triton X-100, 10 mM NaF, 20 mM Na4P2O7, 20 mM β-glycerol phosphate, 1 mM Na3VO4, 10 mg/ml leupeptin, 10 mg/ml aprotinin, and 1 mM PMSF). Protein concentration was determined by bicinchoninic acid assay. For immunoprecipitation, cell lysates were centrifuged at 14,000 g for 10 min at 4°C, and the supernatants were incubated overnight with specific antibodies bound to Protein A-Sepharose beads at 4°C. After incubation, the beads were washed extensively with ice-cold wash buffer (containing 50 mM HEPES, pH 7.6, 150 mM NaCl, and 0.1% Triton X-100). Proteins bound to the beads were eluted by heating at 95°C for 10 min in SDS-PAGE sample loading buffer. The proteins were separated by SDS-PAGE gel, transferred to a nitrocellulose membrane, incubated with specific primary antibody for overnight, and detected with alkaline phosphatase- or horseradish peroxidase-conjugated secondary antibodies by using Colorimetric AP Systems or a VersaDoc Image System. Proteins levels were normalized with β-tubulin levels or an internal reference protein from the same samples using Quantity One software (Bio-Rad, Philadelphia, PA, USA).
All data are expressed as means ± standard deviation (SD). Statistical comparison was performed using Tukeye Kramer post-hoc test or independent samples t-test after ANOVA. Differences were considered significant at p < 0.05. The quantification of the relative increase in protein expression and phosphorylation statuses was normalized with control protein expression in each experiment. All figures were representative of at least three independent experiments.
To observe the potential impact of DAT on survival of cancer cells, HepG2 cells were cultured in DMEM supplemented with 10% FBS in the presence of 0, 25, 50, 100, and 200 µM DAT for 24 h (Fig. 1A), or 100 µM DAT for 0, 12, 24, and 48 h (Fig. 1B). MTT assay was performed to determine cell viability. As shown in Fig. 1A and 1B, DAT administration significantly reduced viabilities of HepG2 cells in dose- and time-dependent manners. Since that the reduction of cell viabilities has statistical significance with 100 µM DAT treatment for 24 h, the same dose and treatment durations of DAT was used to investigate mechanism underlying DAT function.
To confirm whether DAT has a selective cytotoxicity to cancer cells, the similar experiments were carried out on normal murine hepatocyte AML12 cells. We found that the cell viabilities of AML12 remained unchanged under treatment with DAT (Supplementary Fig. 1), suggesting that DAT selectively kills cancerous cells with no harmful effect on normal cells. To observe the impact of DAT on cell proliferation, HepG2 cells were treated with 100 µM of DAT for 24 h. we found that DAT treatment markedly suppressed cell proliferation (Fig. 1C). When serum-starved HepG2 cells were treated with 100 µM DAT for 24 h, we found that DAT supplementation significantly increased cell apoptosis (Fig. 1D, E). Consistent with this result, we also found that the levels of cleaved caspase-3, a marker protein of cell apoptosis, were markedly increased in DAT-treated cells (Fig. 1F).
Taken together, these results suggest that DAT treatment inhibits survival and proliferation of HepG2 cells.
To elucidate the potential mechanism underlying DAT action, HepG2 cells cultured in DMEM supplemented with 10% FBS were treated with 100 µM DAT for 0, 12, 24, and 48 h. As shown in Fig. 2, DAT supplementation significantly attenuated phosphorylation of STAT3 (Fig. 2A), Akt (Fig. 2B), and Erk1/2 (Fig. 2C). Given that the activities of these kinases are represented by their phosphorylation, our results suggest that DAT possesses the inhibiting properties against STAT3, Akt, and Erk1/2 signaling pathways.
Our previous study has shown the involvement of APPL1 in the regulation of leptin-stimulated proliferation and migration of cancer cells through modulating STAT3, Akt, and Erk1/2 signaling pathways [28]. We thus investigated whether DAT administration affects APPL1 expression. HepG2 cells were cultured in DMEM supplemented with 10% FBS and then treated with 100 µM DAT for 0, 12, 24, and 48 h. We found that DAT treatment did not change abundance of intracellular APPL1 protein (Fig. 3A). When APPL1 was immunoprecipitated and then detected by a specific anti-serine or anti-ubiquitin (linkage-specific K63 or K48) antibody, we found that the serine phosphorylation of APPL1 also remained unchanged (Fig. 3B). Surprisingly, DAT administration significantly reduced K63-linked polyubiquitination of APPL1 in a time-dependent manner in HepG2 cells (Fig. 3C, D) whereas K48-linked polyubiquitination of APPL1 was not detected (data not shown). Both TRAF6 and Nedd4 (neural precursor cell expressed, developmentally down-regulated protein 4) have been reported to act as E3 ligases, responsible for K63-linked polyubiquitination of APPL1 [31,37]. Therefore, we checked the levels of intracellular Nedd4 and TRAF6. As expected, DAT treatment reduced TRAF6 expression in a time-dependent manner (Fig. 3E, F) whereas Nedd4 levels remained unchanged (Fig. 3E). To further confirm the function of TRAF6 in mediating APPL1 ubiquitination, TRAF6 was silenced by siRNA technique. Compared with siRNA control, TRAF6 knockdown significantly reduced APPL1 polyubiquitination at K63 (Fig. 3G). These results indicate that DAT supplementation mitigates TRAF6-mediated K63-linked polyubiquitination of APPL1.
To figure out the critical role of K63-linked polyubiquitination of APPL1 in regulating survival of cancer cells, HepG2 cells were infected with adenovirus encoding human full length APPL1 or its K63R mutant (replacement of Lys63 with arginine), followed by incubation with DMEM supplemented with 10% FBS for 24 h. Compared with overexpression of wild-type APPL1, overexpression of K63R mutant largely abolished basal phosphorylation of STAT3, Akt, and Erk1/2 (Fig. 4), as demonstrated by representative images of phosphorylated proteins (Fig. 4A) and its quantitative analysis (Fig. 4B). Using the same strategy as described above, we also found that K63R mutant significantly decreased cell viability (Fig. 5A) and proliferation (Fig. 5B), increased cell apoptosis (Fig. 5C, D) and expression of cleaved caspase-3 (Fig. 5E). These results suggest that K63-linked polyubiquitination of APPL1 is a key player in the regulation of survival and proliferation of HepG2 cancer cells probably through modulating phosphorylation of STAT3, Akt, and Erk1/2.
To further confirm the pivotal role of APPL1 in DAT-induced cytotoxicity on HepG2 cells, APPL1 recovery experiments were performed. The cells overexpressed with wild-type APPL1 were incubated with DMEM supplemented with or without 10% FBS (for MTT and TUNEL assay, respectively) and then treated with 100 µM DAT for 24 h. As shown in Fig. 6, APPL1 overexpression significantly restored DAT-reduced phosphorylation of STAT3, Akt, and Erk1/2, as demonstrated by the representative images of phosphorylated proteins (Fig. 6A) and its quantitative analysis (Fig. 6B). Consistent with these findings, HepG2 cells with APPL1 overexpression exhibited an obvious increase in cell viability (Fig. 7A) and proliferation (Fig. 7B), a reduction in cell apoptosis (Fig. 7C, D) and the expression of cleaved caspase-3 (Fig. 7E), when compared with cells infected with β-gal control adenovirus. These results further indicate that APPL1 plays an important role in DAT-suppressed survival and proliferation of HepG2 cancer cells.
The previous studies have evidenced that DAT has the potential to be developed as an anti-cancer agent. The mechanisms underlying this action are largely associated with down-regulation of STAT3, Akt, and MAPK signaling pathways [20,38-41]. Consistent with these findings, our results demonstrated that DAT supplementation significantly promoted cell apoptosis and reduced viability and proliferation of HepG2 cells (Fig. 1), accompanied with inactivation of STAT3, Akt, and Erk1/2 (Fig. 2). Therefore, our results further confirm that the ability to suppress these signaling pathways is a therapeutic advantage for this antitumor drug.
We have shown previously that APPL1 positively mediates leptin signaling leading to leptin-stimulated proliferation and migration of both HepG2 and MCF-7 cancer cells by directly binding to both leptin receptor and STAT3 [28]. Overexpression or suppression of APPL1 promotes or attenuates, respectively, basal and leptin-induced phosphorylation of STAT3, Akt, and Erk1/2 in these two cancer cell lines, along with enhanced or mitigated cell proliferation and migration [28]. Given that phosphorylation of both Akt and Erk1/2 are suppressed by STAT3 inhibition whereas STAT3 phosphorylation is not affected by the treatment with specific inhibitors of Akt or Erk1/2, STAT3 should be an upstream kinase for both ERK1/2 and Akt [28,42]. These findings strongly suggest that APPL1-mediated STAT3 activation plays a critical role in hepatocellular carcinogenesis. Indeed, overexpression of APPL1 greatly restored DAT-reduced phosphorylation of STAT3, Akt, and Erk1/2, and significantly abrogated cytotoxicity of DAT on HepG2 cells (Fig. 7). However, our results also found that intracellular APPL1 levels remained unchanged in DAT-treated HepG2 cells (Fig. 3A), suggesting that APPL1-mediated cytotoxicity of DAT is modulated by its protein modification but not by its protein abundance.
The function of APPL1 has been reported to be regulated by its phosphorylation at serine residues including Ser401, Ser430, and Ser707 [43-46]. Among them, Ser401 and Ser430 have been found to mediate the crosstalk between the insulin and adiponectin pathways [44,45] whereas Ser707 involves in the regulation of neuroprotective AKT signaling pathway [46]. In the present study, APPL1 was immunoprecipitated and its phosphorylation at serine residues were detected using specific anti-serine antibody, due to lack of commercial phospho-antibodies against specific serine residues of APPL1. Our results found that the serine phosphorylation of APPL1 remained unchanged (Fig. 3B), indicating that APPL1-mediated cytotoxicity of DAT is independent on its phosphorylation at serine residues.
Protein ubiquitination is also one of most important post-translational modifications. Ubiquitin can be attached to specific lysine (K) residues including K48 and K63 in target proteins through three sequential enzymatic reactions catalyzed by an ubiquitin-activating enzyme (E1), an ubiquitin-conjugating enzyme (E2), and an ubiquitin ligase (E3). It is well established that K48-linked polyubiquitination mainly targets the substrate proteins for proteasome-mediated degradation and thus regulates protein stability [47,48]. However, K63-linked ubiquitylation is essential for endocytosis, protein sorting or membrane trafficking events, DNA damage response, and activation of various signaling pathways [31,49-52]. APPL1 has been found to undergo K63-linked polyubiquitination and thus plays a pivotal role in insulin action in hepatocytes and TGFβ type I receptor-intracellular domain-induced progression of prostate tumor [27,31,37], partly through the modulation of the membrane targeting of APPL1 and Akt [31] as well as the promotion of TGFβ-induced nuclear transport of the TGFβ type I receptor intracellular domain [27], respectively. In the present study, we found that DAT administration significantly reduced K63-linked polyubiquitination of APPL1 (Fig. 3C, D). Replacement of Lys63 with arginine (K63R) dramatically reduced phosphorylation of STAT3, Akt, and Erk1/2 (Fig. 4), enhanced cell apoptosis (Fig. 5C–E), and reduced cell viability (Fig. 5A) and proliferation (Fig. 5B), when comparing with wild-type APPL1. Combined with our results showing that DAT mitigated K63-linked polyubiquitination of APPL1 (Fig. 3C), these findings strongly suggest that cytotoxicity of DAT on HepG2 cells is probably regulated by ubiquitin modification of APPL1.
Previous studies have identified Nedd4 and TRAF6 as crucial E3 ligases for APPL1 ubiquitination [31,37]. TRAF6 are also known to be over-expressed in cancer tissues and may contribute to tumorigenesis and cancer metastasis including HCC [53,54]. Thus, TRAF6 might become a predictive and therapeutic biomarker for HCC. In the present study, we found that the levels of intracellular TRAF6 but not Nedd4 were reduced by DAT administration in a time-dependent manner (Fig. 3E, F). This result is consistent with a previous study showing that DAT induces proteasomal degradation of TRAF6 in primary effusion lymphoma [55]. In addition, genetic inhibition of TRAF6 significantly attenuated K63-linked polyubiquitination of APPL1 (Fig. 3G). Hence, our results indicate that reduction of TRAF6 by DAT is responsible for downregulation of K63-linked polyubiquitination of APPL1.
Constitutive activation of STAT3, Akt, and Erk1/2 has been found in human HCC tissues and may provide novel and promising therapeutic targets for HCC treatment [56,57], Consistently, HepG2 cells display a high basal phosphorylation of these signaling molecules [28,56,57]. In addition, our previous study has confirmed that the protein levels and phosphorylation statues of APPL1 are highly expressed in human HCC tissues and that APPL1 is positively involved in the regulation of phosphorylation of STAT3, Akt, and Erk1/2 in HepG2 cells [28]. In the present study, we found that DAT treatment significantly inhibited phosphorylation of STAT3, Akt, and Erk1/2 in a time-dependent manner (Fig. 2). Given that 1) DAT downregulated K63-linked polyubiquitination of APPL1 with the same administration duration (Fig. 3) as DAT did on phosphorylation of these signaling molecules (Fig. 2), and 2) overexpression of APPL1 WT upregulated phosphorylation of these proteins, which was diminished in the cells with APPL1 K63R mutation (Fig. 4), it would be rational to conclude that DAT-suppressed polyubiquitination of APPL1 is responsible for DAT-suppressed phosphorylation of STAT3, Akt, and Erk1/2.
Taken together, the present in vitro study identifies that the inhibitory effects of DAT on TRAF6-mediated K63-linked polyubiquitination of APPL1 and subsequent hypophosphorylation of STAT3, Akt, and Erk1/2 may account for its cytotoxicity on HepG2 cells (Fig. 8). However, the clinical or in vivo significance of K63-linked polyubiquitination of APPL1 in mediating DAT’s cytotoxicity against HCC remains unclear and needs more studies.
Supplementary data including one figure can be found with this article online at https://doi.org/10.4196/kjpp.2022.26.6.457.
REFERENCES
1. Balogh J, Victor D 3rd, Asham EH, Burroughs SG, Boktour M, Saharia A, Li X, Ghobrial RM, Monsour HP Jr. 2016; Hepatocellular carcinoma: a review. J Hepatocell Carcinoma. 3:41–53. DOI: 10.2147/JHC.S61146. PMID: 27785449. PMCID: PMC5063561.


2. Chacko S, Samanta S. 2016; "Hepatocellular carcinoma: a life-threatening disease". Biomed Pharmacother. 84:1679–1688. DOI: 10.1016/j.biopha.2016.10.078. PMID: 27823920. PMID: https://www.scopus.com/inward/record.uri?partnerID=HzOxMe3b&scp=85001969730&origin=inward.


3. Best J, Schotten C, Theysohn JM, Wetter A, Müller S, Radünz S, Schulze M, Canbay A, Dechêne A, Gerken G. 2017; Novel implications in the treatment of hepatocellular carcinoma. Ann Gastroenterol. 30:23–32. DOI: 10.20524/aog.2016.0092. PMID: 28042235. PMCID: PMC5198244. PMID: https://www.scopus.com/inward/record.uri?partnerID=HzOxMe3b&scp=85008180773&origin=inward.
4. Dutta R, Mahato RI. 2017; Recent advances in hepatocellular carcinoma therapy. Pharmacol Ther. 173:106–117. DOI: 10.1016/j.pharmthera.2017.02.010. PMID: 28174094. PMCID: PMC5777523. PMID: https://www.scopus.com/inward/record.uri?partnerID=HzOxMe3b&scp=85012930297&origin=inward.


5. Sapisochin G, Bruix J. 2017; Liver transplantation for hepatocellular carcinoma: outcomes and novel surgical approaches. Nat Rev Gastroenterol Hepatol. 14:203–217. DOI: 10.1038/nrgastro.2016.193. PMID: 28053342. PMID: https://www.scopus.com/inward/record.uri?partnerID=HzOxMe3b&scp=85008330472&origin=inward.


6. Adaki S, Adaki R, Shah K, Karagir A. 2014; Garlic: review of literature. Indian J Cancer. 51:577–581. DOI: 10.4103/0019-509X.175383. PMID: 26842201. PMID: https://www.scopus.com/inward/record.uri?partnerID=HzOxMe3b&scp=84958178966&origin=inward.


7. Yu L, Li S, Tang X, Li Z, Zhang J, Xue X, Han J, Liu Y, Zhang Y, Zhang Y, Xu Y, Yang Y, Wang H. 2017; Diallyl trisulfide ameliorates myocardial ischemia-reperfusion injury by reducing oxidative stress and endoplasmic reticulum stress-mediated apoptosis in type 1 diabetic rats: role of SIRT1 activation. Apoptosis. 22:942–954. DOI: 10.1007/s10495-017-1378-y. PMID: 28455824. PMID: https://www.scopus.com/inward/record.uri?partnerID=HzOxMe3b&scp=85018294902&origin=inward.


8. Zhu X, Zhang F, Zhou L, Kong D, Chen L, Lu Y, Zheng S. 2014; Diallyl trisulfide attenuates carbon tetrachloride-caused liver injury and fibrogenesis and reduces hepatic oxidative stress in rats. Naunyn Schmiedebergs Arch Pharmacol. 387:445–455. DOI: 10.1007/s00210-014-0959-3. PMID: 24557053. PMID: https://www.scopus.com/inward/record.uri?partnerID=HzOxMe3b&scp=84899965294&origin=inward.


9. Tsai CY, Wen SY, Shibu MA, Yang YC, Peng H, Wang B, Wei YM, Chang HY, Lee CY, Huang CY, Kuo WW. 2015; Diallyl trisulfide protects against high glucose-induced cardiac apoptosis by stimulating the production of cystathionine gamma-lyase-derived hydrogen sulfide. Int J Cardiol. 195:300–310. DOI: 10.1016/j.ijcard.2015.05.111. PMID: 26056963. PMID: https://www.scopus.com/inward/record.uri?partnerID=HzOxMe3b&scp=84937611169&origin=inward.


10. Hayashida R, Kondo K, Morita S, Unno K, Shintani S, Shimizu Y, Calvert JW, Shibata R, Murohara T. 2017; Diallyl trisulfide augments ischemia-induced angiogenesis via an endothelial nitric oxide synthase-dependent mechanism. Circ J. 81:870–878. DOI: 10.1253/circj.CJ-16-1097. PMID: 28216514. PMID: https://www.scopus.com/inward/record.uri?partnerID=HzOxMe3b&scp=85019773175&origin=inward.


11. Chen LY, Chen Q, Cheng YF, Jin HH, Kong DS, Zhang F, Wu L, Shao JJ, Zheng SZ. 2016; Diallyl trisulfide attenuates ethanol-induced hepatic steatosis by inhibiting oxidative stress and apoptosis. Biomed Pharmacother. 79:35–43. DOI: 10.1016/j.biopha.2016.01.009. PMID: 27044810. PMID: https://www.scopus.com/inward/record.uri?partnerID=HzOxMe3b&scp=84961894562&origin=inward.


12. Wang S, Li M, Wang X, Li X, Yin H, Jiang L, Han W, Irving G, Zeng T, Xie K. 2017; Diallyl trisulfide attenuated n-hexane induced neurotoxicity in rats by modulating P450 enzymes. Chem Biol Interact. 265:1–7. DOI: 10.1016/j.cbi.2017.01.013. PMID: 28115069. PMID: https://www.scopus.com/inward/record.uri?partnerID=HzOxMe3b&scp=85010399976&origin=inward.


13. Lii CK, Huang CY, Chen HW, Chow MY, Lin YR, Huang CS, Tsai CW. 2012; Diallyl trisulfide suppresses the adipogenesis of 3T3-L1 preadipocytes through ERK activation. Food Chem Toxicol. 50:478–484. DOI: 10.1016/j.fct.2011.11.020. PMID: 22137902. PMID: https://www.scopus.com/inward/record.uri?partnerID=HzOxMe3b&scp=84863298355&origin=inward.


14. Zhang F, Zhang Y, Wang K, Liu G, Yang M, Zhao Z, Li S, Cai J, Cao J. 2016; Protective effect of diallyl trisulfide against naphthalene-induced oxidative stress and inflammatory damage in mice. Int J Immunopathol Pharmacol. 29:205–216. DOI: 10.1177/0394632015627160. PMID: 26813860. PMCID: PMC5806724. PMID: https://www.scopus.com/inward/record.uri?partnerID=HzOxMe3b&scp=84966701534&origin=inward.


15. Kiesel VA, Stan SD. 2017; Diallyl trisulfide, a chemopreventive agent from Allium vegetables, inhibits alpha-secretases in breast cancer cells. Biochem Biophys Res Commun. 484:833–838. DOI: 10.1016/j.bbrc.2017.01.184. PMID: 28161636. PMID: https://www.scopus.com/inward/record.uri?partnerID=HzOxMe3b&scp=85011878196&origin=inward.


16. Jiang X, Zhu X, Liu N, Xu H, Zhao Z, Li S, Li S, Cai J, Cao J. 2017; Diallyl trisulfide inhibits growth of NCI-H460 in vitro and in vivo, and ameliorates cisplatin-induced oxidative injury in the treatment of lung carcinoma in xenograft mice. Int J Biol Sci. 13:167–178. DOI: 10.7150/ijbs.16828. PMID: 28255269. PMCID: PMC5332871. PMID: https://www.scopus.com/inward/record.uri?partnerID=HzOxMe3b&scp=85011831927&origin=inward.


17. Jiang XY, Zhu XS, Xu HY, Zhao ZX, Li SY, Li SZ, Cai JH, Cao JM. 2017; Diallyl trisulfide suppresses tumor growth through the attenuation of Nrf2/Akt and activation of p38/JNK and potentiates cisplatin efficacy in gastric cancer treatment. Acta Pharmacol Sin. 38:1048–1058. DOI: 10.1038/aps.2016.176. PMID: 28344324. PMCID: PMC5519247. PMID: https://www.scopus.com/inward/record.uri?partnerID=HzOxMe3b&scp=85021820280&origin=inward.


18. Hosono T, Fukao T, Ogihara J, Ito Y, Shiba H, Seki T, Ariga T. 2005; Diallyl trisulfide suppresses the proliferation and induces apoptosis of human colon cancer cells through oxidative modification of beta-tubulin. J Biol Chem. 280:41487–41493. DOI: 10.1074/jbc.M507127200. PMID: 16219763. PMID: https://www.scopus.com/inward/record.uri?partnerID=HzOxMe3b&scp=29244484434&origin=inward.


19. Lai KC, Hsu SC, Yang JS, Yu CC, Lein JC, Chung JG. 2015; Diallyl trisulfide inhibits migration, invasion and angiogenesis of human colon cancer HT-29 cells and umbilical vein endothelial cells, and suppresses murine xenograft tumour growth. J Cell Mol Med. 19:474–484. DOI: 10.1111/jcmm.12486. PMID: 25403643. PMCID: PMC4407594. PMID: https://www.scopus.com/inward/record.uri?partnerID=HzOxMe3b&scp=84921786219&origin=inward.


20. Xiao D, Choi S, Johnson DE, Vogel VG, Johnson CS, Trump DL, Lee YJ, Singh SV. 2004; Diallyl trisulfide-induced apoptosis in human prostate cancer cells involves c-Jun N-terminal kinase and extracellular-signal regulated kinase-mediated phosphorylation of Bcl-2. Oncogene. 23:5594–5606. DOI: 10.1038/sj.onc.1207747. PMID: 15184882. PMID: https://www.scopus.com/inward/record.uri?partnerID=HzOxMe3b&scp=3342895063&origin=inward.


21. Xiao D, Lew KL, Kim YA, Zeng Y, Hahm ER, Dhir R, Singh SV. 2006; Diallyl trisulfide suppresses growth of PC-3 human prostate cancer xenograft in vivo in association with Bax and Bak induction. Clin Cancer Res. 12:6836–6843. DOI: 10.1158/1078-0432.CCR-06-1273. PMID: 17121905. PMID: https://www.scopus.com/inward/record.uri?partnerID=HzOxMe3b&scp=33845299153&origin=inward.


22. Li Y, Zhang J, Zhang L, Si M, Yin H, Li J. 2013; Diallyl trisulfide inhibits proliferation, invasion and angiogenesis of osteosarcoma cells by switching on suppressor microRNAs and inactivating of Notch-1 signaling. Carcinogenesis. 34:1601–1610. DOI: 10.1093/carcin/bgt065. PMID: 23430952. PMID: https://www.scopus.com/inward/record.uri?partnerID=HzOxMe3b&scp=84880320805&origin=inward.


23. Zhang ZM, Yang XY, Deng SH, Xu W, Gao HQ. 2007; Anti-tumor effects of polybutylcyanoacrylate nanoparticles of diallyl trisulfide on orthotopic transplantation tumor model of hepatocellular carcinoma in BALB/c nude mice. Chin Med J (Engl). 120:1336–1342. DOI: 10.1097/00029330-200708010-00008. PMID: 17711740. PMID: https://www.scopus.com/inward/record.uri?partnerID=HzOxMe3b&scp=34548296990&origin=inward.


24. Deepa SS, Dong LQ. 2009; APPL1: role in adiponectin signaling and beyond. Am J Physiol Endocrinol Metab. 296:E22–E36. DOI: 10.1152/ajpendo.90731.2008. PMID: 18854421. PMCID: PMC2636986. PMID: https://www.scopus.com/inward/record.uri?partnerID=HzOxMe3b&scp=58249105213&origin=inward.


25. Liu Z, Xiao T, Peng X, Li G, Hu F. 2017; APPLs: more than just adiponectin receptor binding proteins. Cell Signal. 32:76–84. DOI: 10.1016/j.cellsig.2017.01.018. PMID: 28108259. PMID: https://www.scopus.com/inward/record.uri?partnerID=HzOxMe3b&scp=85010297595&origin=inward.


26. Johnson IR, Parkinson-Lawrence EJ, Keegan H, Spillane CD, Barry-O'Crowley J, Watson WR, Selemidis S, Butler LM, O'Leary JJ, Brooks DA. 2015; Endosomal gene expression: a new indicator for prostate cancer patient prognosis? Oncotarget. 6:37919–37929. DOI: 10.18632/oncotarget.6114. PMID: 26473288. PMCID: PMC4741974. PMID: https://www.scopus.com/inward/record.uri?partnerID=HzOxMe3b&scp=84947786217&origin=inward.


27. Song J, Mu Y, Li C, Bergh A, Miaczynska M, Heldin CH, Landström M. 2016; APPL proteins promote TGFβ-induced nuclear transport of the TGFβ type I receptor intracellular domain. Oncotarget. 7:279–292. DOI: 10.18632/oncotarget.6346. PMID: 26583432. PMCID: PMC4807998. PMID: https://www.scopus.com/inward/record.uri?partnerID=HzOxMe3b&scp=85020883234&origin=inward.


28. Ding Y, Cao Y, Wang B, Wang L, Zhang Y, Zhang D, Chen X, Li M, Wang C. 2016; APPL1-mediating leptin signaling contributes to proliferation and migration of cancer cells. PLoS One. 11:e0166172. DOI: 10.1371/journal.pone.0166172. PMID: 27820851. PMCID: PMC5098739. PMID: https://www.scopus.com/inward/record.uri?partnerID=HzOxMe3b&scp=84994589804&origin=inward.


29. Hennig J, McShane MP, Cordes N, Eke I. 2014; APPL proteins modulate DNA repair and radiation survival of pancreatic carcinoma cells by regulating ATM. Cell Death Dis. 5:e1199. DOI: 10.1038/cddis.2014.167. PMID: 24763056. PMCID: PMC4001316. PMID: https://www.scopus.com/inward/record.uri?partnerID=HzOxMe3b&scp=84901045038&origin=inward.


30. Kunkel TA, Roberts JD, Zakour RA. 1987; Rapid and efficient site-specific mutagenesis without phenotypic selection. Methods Enzymol. 154:367–382. DOI: 10.1016/0076-6879(87)54085-X. PMID: 3323813. PMID: https://www.scopus.com/inward/record.uri?partnerID=HzOxMe3b&scp=0023613953&origin=inward.


31. Cheng KK, Lam KS, Wang Y, Wu D, Zhang M, Wang B, Li X, Hoo RL, Huang Z, Sweeney G, Xu A. 2013; TRAF6-mediated ubiquitination of APPL1 enhances hepatic actions of insulin by promoting the membrane translocation of Akt. Biochem J. 455:207–216. DOI: 10.1042/BJ20130760. PMID: 23909487. PMID: https://www.scopus.com/inward/record.uri?partnerID=HzOxMe3b&scp=84884787995&origin=inward.


32. Wang C, Xin X, Xiang R, Ramos FJ, Liu M, Lee HJ, Chen H, Mao X, Kikani CK, Liu F, Dong LQ. 2009; Yin-Yang regulation of adiponectin signaling by APPL isoforms in muscle cells. J Biol Chem. 284:31608–31615. DOI: 10.1074/jbc.M109.010355. PMID: 19661063. PMCID: PMC2797231. PMID: https://www.scopus.com/inward/record.uri?partnerID=HzOxMe3b&scp=70450265225&origin=inward.


33. Ding Y, Zhang D, Wang B, Zhang Y, Wang L, Chen X, Li M, Tang Z, Wang C. 2016; APPL1-mediated activation of STAT3 contributes to inhibitory effect of adiponectin on hepatic gluconeogenesis. Mol Cell Endocrinol. 433:12–19. DOI: 10.1016/j.mce.2016.05.021. PMID: 27246173. PMID: https://www.scopus.com/inward/record.uri?partnerID=HzOxMe3b&scp=84973326543&origin=inward.


34. Guan F, Ding Y, Zhang Y, Zhou Y, Li M, Wang C. 2016; Curcumin suppresses proliferation and migration of MDA-MB-231 breast cancer cells through autophagy-dependent Akt degradation. PLoS One. 11:e0146553. DOI: 10.1371/journal.pone.0146553. PMID: 26752181. PMCID: PMC4708990. PMID: https://www.scopus.com/inward/record.uri?partnerID=HzOxMe3b&scp=84954567599&origin=inward.


35. Ding Y, Wang B, Chen X, Zhou Y, Ge J. 2017; Staurosporine suppresses survival of HepG2 cancer cells through Omi/HtrA2-mediated inhibition of PI3K/Akt signaling pathway. Tumour Biol. 39:1010428317694317. DOI: 10.1177/1010428317694317. PMID: 28349827. PMID: 26a7cdaef35d4ddb81d28370ec20d244. PMID: https://www.scopus.com/inward/record.uri?partnerID=HzOxMe3b&scp=85016925370&origin=inward.


36. Bai J, Cederbaum AI. 2006; Cycloheximide protects HepG2 cells from serum withdrawal-induced apoptosis by decreasing p53 and phosphorylated p53 levels. J Pharmacol Exp Ther. 319:1435–1443. DOI: 10.1124/jpet.106.110007. PMID: 16971506. PMID: https://www.scopus.com/inward/record.uri?partnerID=HzOxMe3b&scp=33751188946&origin=inward.
37. Pilecka I, Sadowski L, Kalaidzidis Y, Miaczynska M. 2011; Recruitment of APPL1 to ubiquitin-rich aggresomes in response to proteasomal impairment. Exp Cell Res. 317:1093–1107. DOI: 10.1016/j.yexcr.2011.02.002. PMID: 21320486. PMCID: PMC3072527. PMID: https://www.scopus.com/inward/record.uri?partnerID=HzOxMe3b&scp=79953069853&origin=inward.


38. Xiao D, Singh SV. 2006; Diallyl trisulfide, a constituent of processed garlic, inactivates Akt to trigger mitochondrial translocation of BAD and caspase-mediated apoptosis in human prostate cancer cells. Carcinogenesis. 27:533–540. DOI: 10.1093/carcin/bgi228. PMID: 16169930. PMID: https://www.scopus.com/inward/record.uri?partnerID=HzOxMe3b&scp=33644869135&origin=inward.


39. Shrotriya S, Kundu JK, Na HK, Surh YJ. 2010; Diallyl trisulfide inhibits phorbol ester-induced tumor promotion, activation of AP-1, and expression of COX-2 in mouse skin by blocking JNK and Akt signaling. Cancer Res. 70:1932–1940. Erratum in: Cancer Res. 2010;70:3414. DOI: 10.1158/0008-5472.CAN-09-3501. PMID: 20179211. PMID: https://www.scopus.com/inward/record.uri?partnerID=HzOxMe3b&scp=77950280661&origin=inward.


40. Chandra-Kuntal K, Singh SV. 2010; Diallyl trisulfide inhibits activation of signal transducer and activator of transcription 3 in prostate cancer cells in culture and in vivo. Cancer Prev Res (Phila). 3:1473–1483. DOI: 10.1158/1940-6207.CAPR-10-0123. PMID: 20959517. PMCID: PMC2988081. PMID: https://www.scopus.com/inward/record.uri?partnerID=HzOxMe3b&scp=78649238180&origin=inward.


41. Wang H, Sun N, Li X, Li K, Tian J, Li J. 2016; Diallyl trisulfide induces osteosarcoma cell apoptosis through reactive oxygen species-mediated downregulation of the PI3K/Akt pathway. Oncol Rep. 35:3648–3658. DOI: 10.3892/or.2016.4722. PMID: 27035545. PMID: https://www.scopus.com/inward/record.uri?partnerID=HzOxMe3b&scp=84964910272&origin=inward.


42. Saxena NK, Sharma D, Ding X, Lin S, Marra F, Merlin D, Anania FA. 2007; Concomitant activation of the JAK/STAT, PI3K/AKT, and ERK signaling is involved in leptin-mediated promotion of invasion and migration of hepatocellular carcinoma cells. Cancer Res. 67:2497–2507. DOI: 10.1158/0008-5472.CAN-06-3075. PMID: 17363567. PMCID: PMC2925446. PMID: https://www.scopus.com/inward/record.uri?partnerID=HzOxMe3b&scp=34047250349&origin=inward.


43. Gant-Branum RL, Broussard JA, Mahsut A, Webb DJ, McLean JA. 2010; Identification of phosphorylation sites within the signaling adaptor APPL1 by mass spectrometry. J Proteome Res. 9:1541–1548. DOI: 10.1021/pr901043e. PMID: 20095645. PMCID: PMC2845304. PMID: https://www.scopus.com/inward/record.uri?partnerID=HzOxMe3b&scp=77949780036&origin=inward.


44. Holmes RM, Yi Z, De Filippis E, Berria R, Shahani S, Sathyanarayana P, Sherman V, Fujiwara K, Meyer C, Christ-Roberts C, Hwang H, Finlayson J, Dong LQ, Mandarino LJ, Bajaj M. 2011; Increased abundance of the adaptor protein containing pleckstrin homology domain, phosphotyrosine binding domain and leucine zipper motif (APPL1) in patients with obesity and type 2 diabetes: evidence for altered adiponectin signalling. Diabetologia. 54:2122–2131. DOI: 10.1007/s00125-011-2173-x. PMID: 21562756. PMCID: PMC3131511. PMID: https://www.scopus.com/inward/record.uri?partnerID=HzOxMe3b&scp=79960912411&origin=inward.


45. Liu M, Zhou L, Wei L, Villarreal R, Yang X, Hu D, Riojas RA, Holmes BM, Langlais PR, Lee H, Dong LQ. 2012; Phosphorylation of adaptor protein containing pleckstrin homology domain, phosphotyrosine binding domain, and leucine zipper motif 1 (APPL1) at Ser430 mediates endoplasmic reticulum (ER) stress-induced insulin resistance in hepatocytes. J Biol Chem. 287:26087–26093. DOI: 10.1074/jbc.M112.372292. PMID: 22685300. PMCID: PMC3406692. PMID: https://www.scopus.com/inward/record.uri?partnerID=HzOxMe3b&scp=84864390268&origin=inward.


46. Wang J, Lu W, Chen L, Zhang P, Qian T, Cao W, Luo J. 2016; Serine 707 of APPL1 is critical for the synaptic NMDA receptor-mediated Akt phosphorylation signaling pathway. Neurosci Bull. 32:323–330. DOI: 10.1007/s12264-016-0042-9. PMID: 27300007. PMCID: PMC5563782. PMID: https://www.scopus.com/inward/record.uri?partnerID=HzOxMe3b&scp=84974851680&origin=inward.


47. Komander D. 2009; The emerging complexity of protein ubiquitination. Biochem Soc Trans. 37(Pt 5):937–953. DOI: 10.1042/BST0370937. PMID: 19754430. PMID: https://www.scopus.com/inward/record.uri?partnerID=HzOxMe3b&scp=70350150000&origin=inward.


48. Xu P, Duong DM, Seyfried NT, Cheng D, Xie Y, Robert J, Rush J, Hochstrasser M, Finley D, Peng J. 2009; Quantitative proteomics reveals the function of unconventional ubiquitin chains in proteasomal degradation. Cell. 137:133–145. DOI: 10.1016/j.cell.2009.01.041. PMID: 19345192. PMCID: PMC2668214. PMID: https://www.scopus.com/inward/record.uri?partnerID=HzOxMe3b&scp=63049125531&origin=inward.


49. Deng L, Wang C, Spencer E, Yang L, Braun A, You J, Slaughter C, Pickart C, Chen ZJ. 2000; Activation of the IkappaB kinase complex by TRAF6 requires a dimeric ubiquitin-conjugating enzyme complex and a unique polyubiquitin chain. Cell. 103:351–361. DOI: 10.1016/S0092-8674(00)00126-4. PMID: 11057907. PMID: https://www.scopus.com/inward/record.uri?partnerID=HzOxMe3b&scp=0034644474&origin=inward.


50. Hoege C, Pfander B, Moldovan GL, Pyrowolakis G, Jentsch S. 2002; RAD6-dependent DNA repair is linked to modification of PCNA by ubiquitin and SUMO. Nature. 419:135–141. DOI: 10.1038/nature00991. PMID: 12226657. PMID: https://www.scopus.com/inward/record.uri?partnerID=HzOxMe3b&scp=0037068455&origin=inward.


51. Lauwers E, Jacob C, André B. 2009; K63-linked ubiquitin chains as a specific signal for protein sorting into the multivesicular body pathway. J Cell Biol. 185:493–502. DOI: 10.1083/jcb.200810114. PMID: 19398763. PMCID: PMC2700384. PMID: https://www.scopus.com/inward/record.uri?partnerID=HzOxMe3b&scp=65649128660&origin=inward.


52. Silva GM, Finley D, Vogel C. 2015; K63 polyubiquitination is a new modulator of the oxidative stress response. Nat Struct Mol Biol. 22:116–123. DOI: 10.1038/nsmb.2955. PMID: 25622294. PMCID: PMC4318705. PMID: https://www.scopus.com/inward/record.uri?partnerID=HzOxMe3b&scp=84926417515&origin=inward.


53. Zu Y, Yang Y, Zhu J, Bo X, Hou S, Zhang B, Qiu J, Zheng J. 2016; MiR-146a suppresses hepatocellular carcinoma by downregulating TRAF6. Am J Cancer Res. 6:2502–2513. PMID: 27904767. PMCID: PMC5126269.
54. Li JJ, Luo J, Lu JN, Liang XN, Luo YH, Liu YR, Yang J, Ding H, Qin GH, Yang LH, Dang YW, Yang H, Chen G. 2016; Relationship between TRAF6 and deterioration of HCC: an immunohistochemical and in vitro study. Cancer Cell Int. 16:76. Erratum in: Cancer Cell Int. 2020;20:60. DOI: 10.1186/s12935-016-0352-z. PMID: 27708550. PMCID: PMC5041287. PMID: https://www.scopus.com/inward/record.uri?partnerID=HzOxMe3b&scp=84988930452&origin=inward.


55. Shigemi Z, Furukawa Y, Hosokawa K, Minami S, Matsuhiro J, Nakata S, Watanabe T, Kagawa H, Nakagawa K, Takeda H, Fujimuro M. 2016; Diallyl trisulfide induces apoptosis by suppressing NF-κB signaling through destabilization of TRAF6 in primary effusion lymphoma. Int J Oncol. 48:293–304. DOI: 10.3892/ijo.2015.3247. PMID: 26647777. PMID: https://www.scopus.com/inward/record.uri?partnerID=HzOxMe3b&scp=84953329084&origin=inward.


56. Schmitz KJ, Wohlschlaeger J, Lang H, Sotiropoulos GC, Malago M, Steveling K, Reis H, Cicinnati VR, Schmid KW, Baba HA. 2008; Activation of the ERK and AKT signalling pathway predicts poor prognosis in hepatocellular carcinoma and ERK activation in cancer tissue is associated with hepatitis C virus infection. J Hepatol. 48:83–90. DOI: 10.1016/j.jhep.2007.08.018. PMID: 17998146. PMID: https://www.scopus.com/inward/record.uri?partnerID=HzOxMe3b&scp=36549013178&origin=inward.


57. Yang J, Cai X, Lu W, Hu C, Xu X, Yu Q, Cao P. 2013; Evodiamine inhibits STAT3 signaling by inducing phosphatase shatterproof 1 in hepatocellular carcinoma cells. Cancer Lett. 328:243–251. DOI: 10.1016/j.canlet.2012.09.019. PMID: 23032719. PMID: https://www.scopus.com/inward/record.uri?partnerID=HzOxMe3b&scp=84870364791&origin=inward.


Fig. 1
Effect of DAT on cell survival and proliferation of HepG2 cells.
(A) Dose-response of DAT on cell viability. (B) Time-response of DAT on cell viability. (C) Effect of DAT on cell proliferation. (D) Representative images of cell apoptosis by DAT (×400). (E) Quantitative analysis of cell apoptosis in (D). (F) Impact of DAT on cleaved caspase-3 levels. DAT, diallyl trisulfide. *p < 0.05, **p < 0.01, ***p < 0.001 vs. control group.
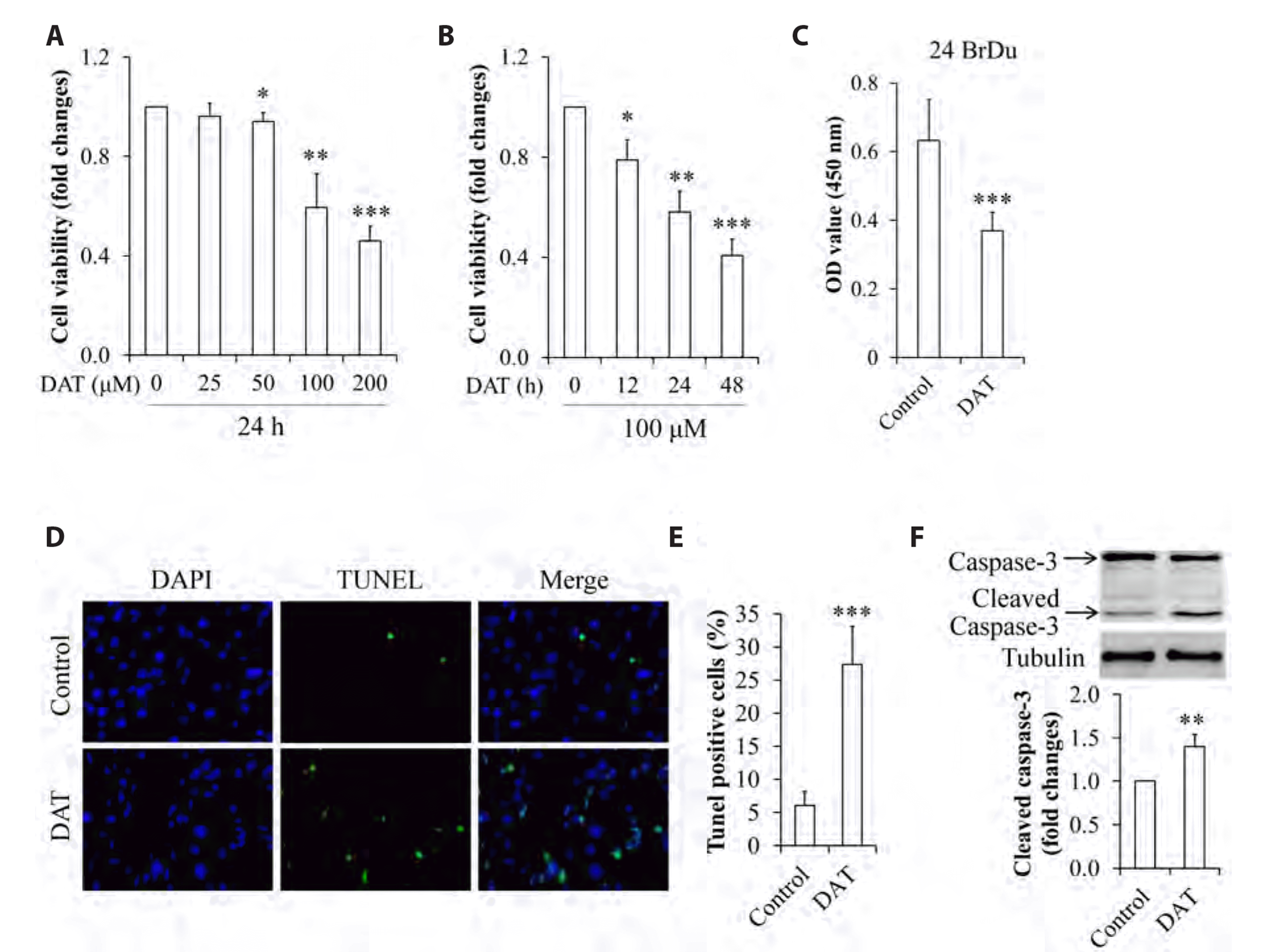
Fig. 2
Effect of DAT on phosphorylation of STAT3, Akt, and Erk1/2 in HepG2 cells.
(A) Impact of DAT on STAT3 phosphorylation. (B) Impact of DAT on Akt phosphorylation. (C) Impact of DAT on Erk1/2 phosphorylation. Upper panels are representative Western blot images and bottom panels are quantitative analysis of the ratio of phosphorylated proteins to the related-proteins. DAT, diallyl trisulfide. *p < 0.05, **p < 0.01, ***p < 0.01 vs. control group.
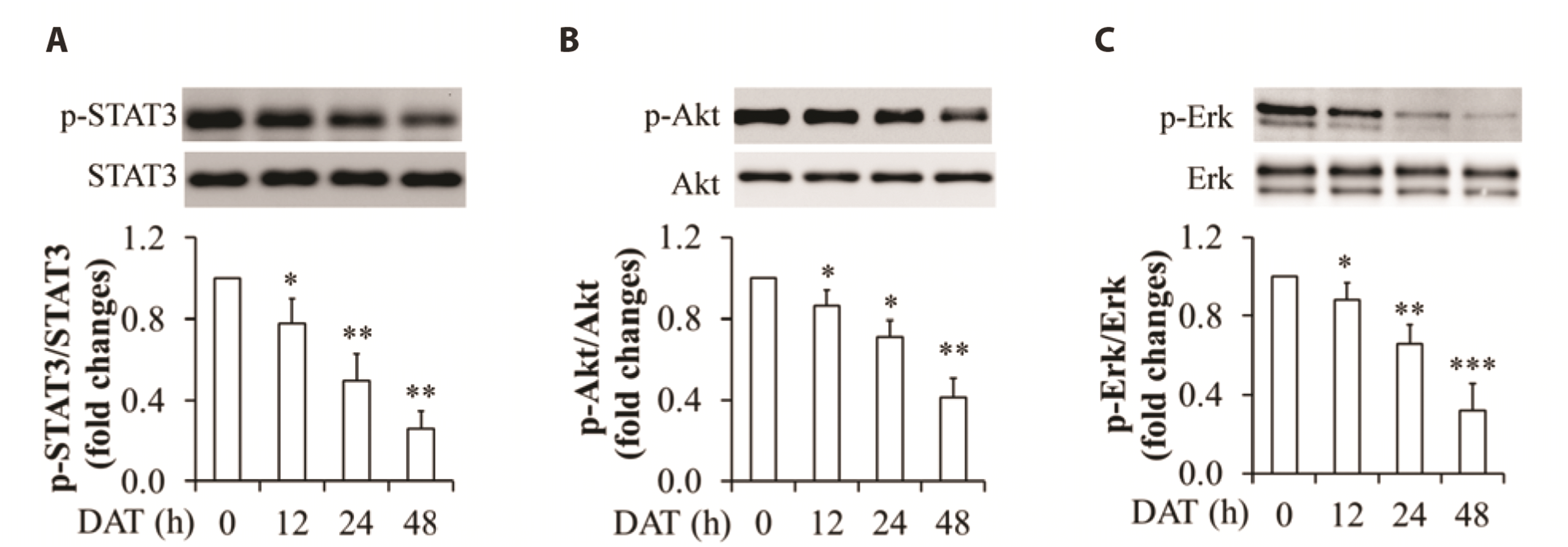
Fig. 3
Effect of DAT on phosphorylation and polyubiquitination of APPL1 in HepG2 cells.
(A) Impact of DAT on the levels of intracellular APPL1 protein. (B) Impact of DAT on serine phosphorylation of APPL1. APPL1 was immunoprecipitated by specific antibody and serine phosphorylation was then detected by western blot using a specific anti-serine antibody. (C) Impact of DAT on K63-linked polyubiquitination of APPL1. APPL1 was immunoprecipitated by specific antibody and polyubiquitination was detected by Western blot using a specific antibody against K63-linked polyubiquitination. (D) Quantitative analysis of K63-linked polyubiquitination of APPL1 in (C). (E) Impact of DAT on the levels of intracellular Nedd4 and TRAF6 proteins. (F) Quantitative analysis of TRAF6 levels in (E). (G) Impact of TRAF6 knockdown on K63-linked polyubiquitination of APPL1. DAT, diallyl trisulfide; APPL1, adaptor protein, phosphotyrosine interacting with PH domain and leucine zipper 1; K63, Lys 63; Nedd4, neural precursor cell expressed, developmentally down-regulated protein 4; TRAF6, TNF receptor-associated factor 6; IP, immunoprecipitation; Ub, ubiquitin; polyub, APPL1 polyubiquitination. *p < 0.05, **p < 0.01 vs. control group.

Fig. 4
Effect of APPL1 K63R mutant on phosphorylation of STAT3, Akt, and Erk1/2 in HepG2 cells.
(A) Representative Western blot images. (B). Quantitative analysis of the ratio of phosphorylated proteins to the related-proteins. APPL1, adaptor protein, phosphotyrosine interacting with PH domain and leucine zipper 1; K63, Lys 63; OE, overexpression; Endo, endogenous; WT, wild type. *p < 0.05, **p < 0.01 vs. control group; ##p < 0.01 vs. APPL1 WT group.
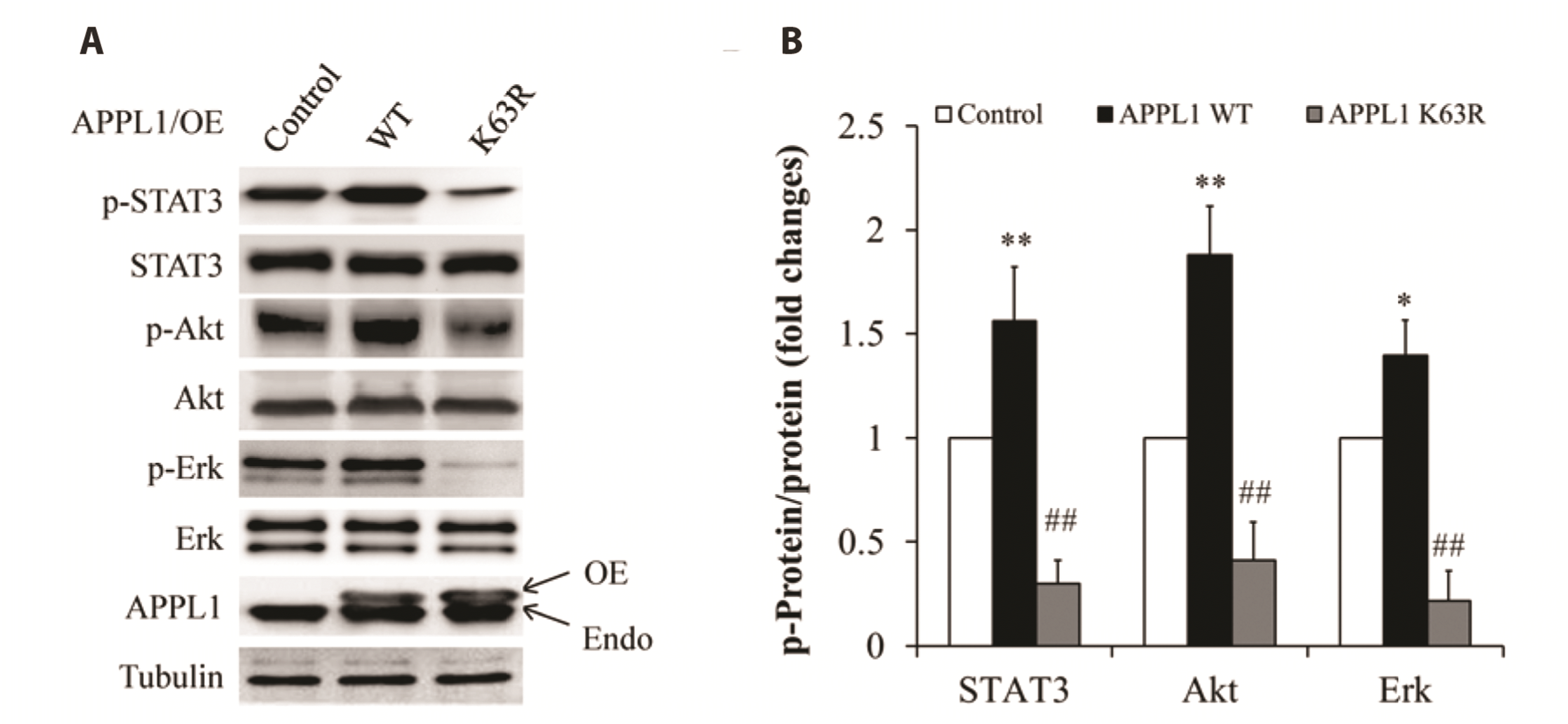
Fig. 5
Effect of APPL1 K63R mutant on survival and proliferation of HepG2 cells.
(A) Impact of K63R mutation of APPL1 on cell viability. (B) Impact of K63 mutation of APPL1 on cell proliferation. (C) Representative images of cell apoptosis by APPL1 K63R mutation (×400). (D) Quantitative analysis of cell apoptosis in (C). (E) Impact of APPL1 K63R mutation on cleaved caspase-3 levels. APPL1, adaptor protein, phosphotyrosine interacting with PH domain and leucine zipper 1; K63, Lys 63; WT, wild type. *p < 0.05, **p < 0.01 vs. control group.
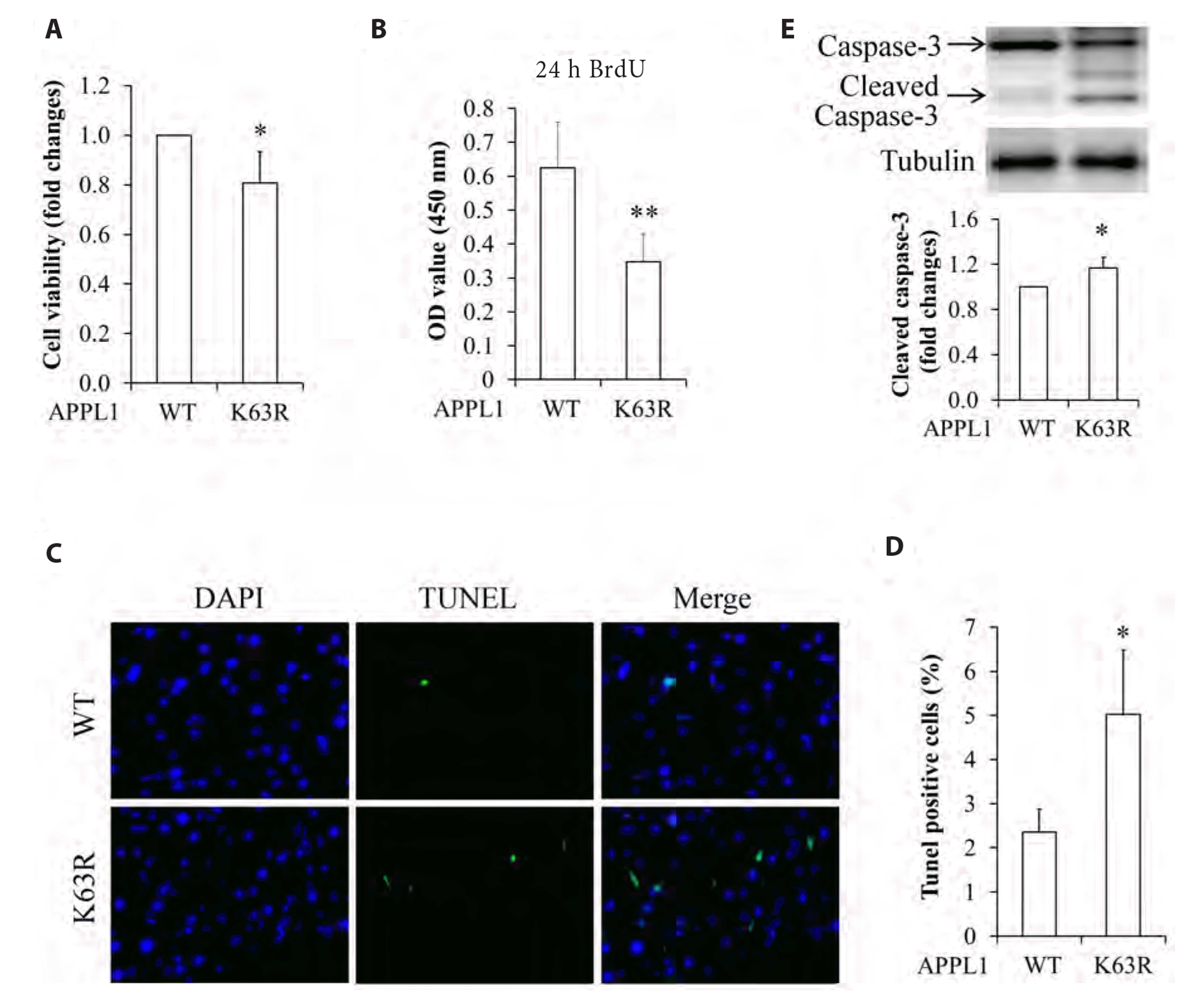
Fig. 6
Effect of overexpression of wild-type APPL1 on phosphorylation of STAT3, Akt, and Erk1/2 in DAT-treated HepG2 cells.
(A) Representative Western blot images. (B) Quantitative analysis of the ratio of phosphorylated proteins to the related-proteins. APPL1, adaptor protein, phosphotyrosine interacting with PH domain and leucine zipper 1; DAT, diallyl trisulfide; OE, overexpression; Endo, endogenous; WT, wild type. **p < 0.01 vs. control group; #p < 0.05, ##p < 0.01 vs. DAT-treated control group.
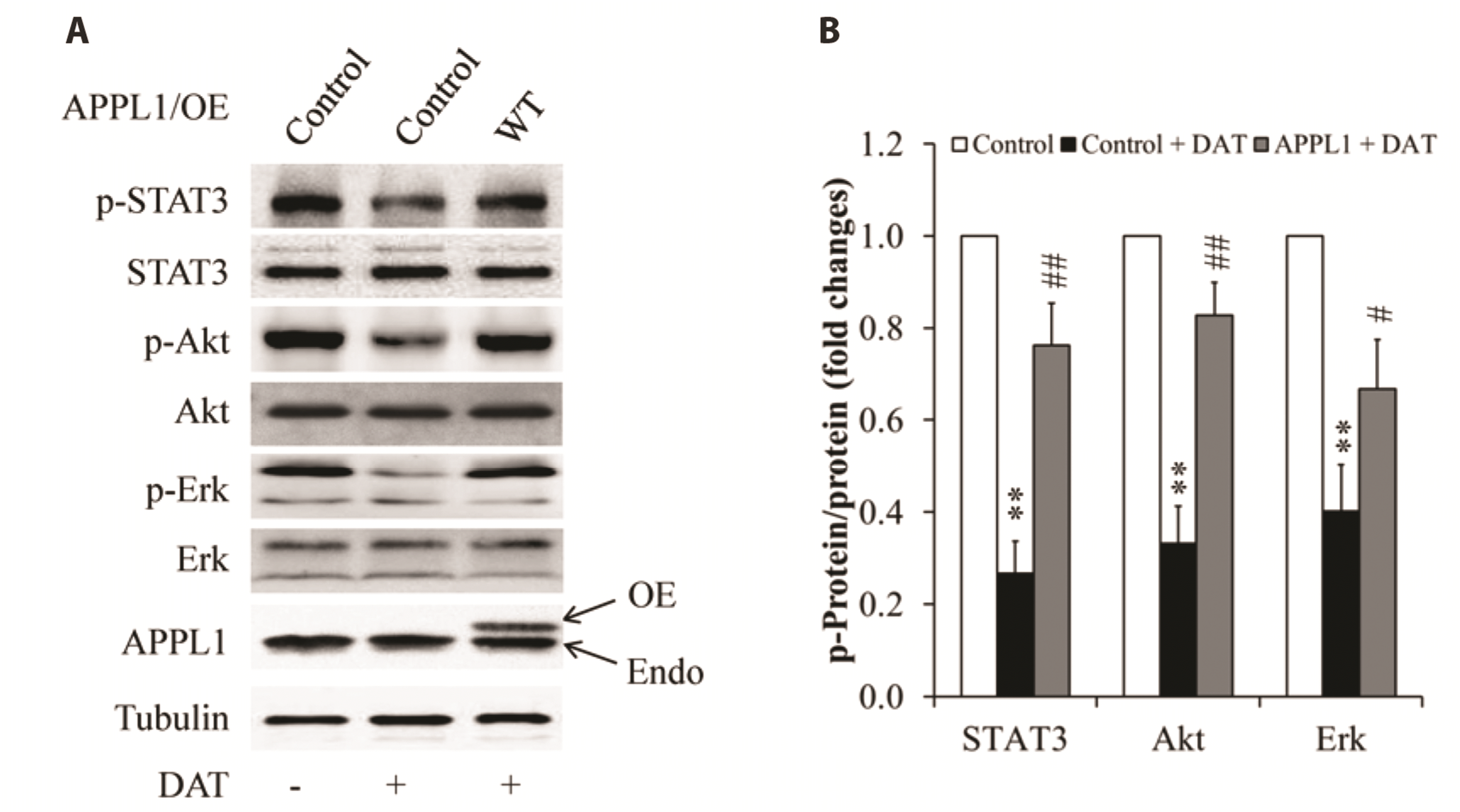
Fig. 7
Effect of overexpression of wild-type APPL1 on cell survival and proliferation in DAT-treated HepG2 cells.
(A) Overexpression of wild-type APPL1 on cell viability. (B) Overexpression of wild-type APPL1 on cell proliferation. (C) Representative images of cell apoptosis by overexpression of wild-type APPL1 (×400). (D) Quantitative analysis of cell apoptosis in (C). (E) Impact of overexpression of wild-type APPL1 on cleaved caspase-3 levels. APPL1, adaptor protein, phosphotyrosine interacting with PH domain and leucine zipper 1; DAT, diallyl trisulfide. **p < 0.01 vs. control group; #p < 0.05, ##p < 0.01 vs. DAT-treated control group.
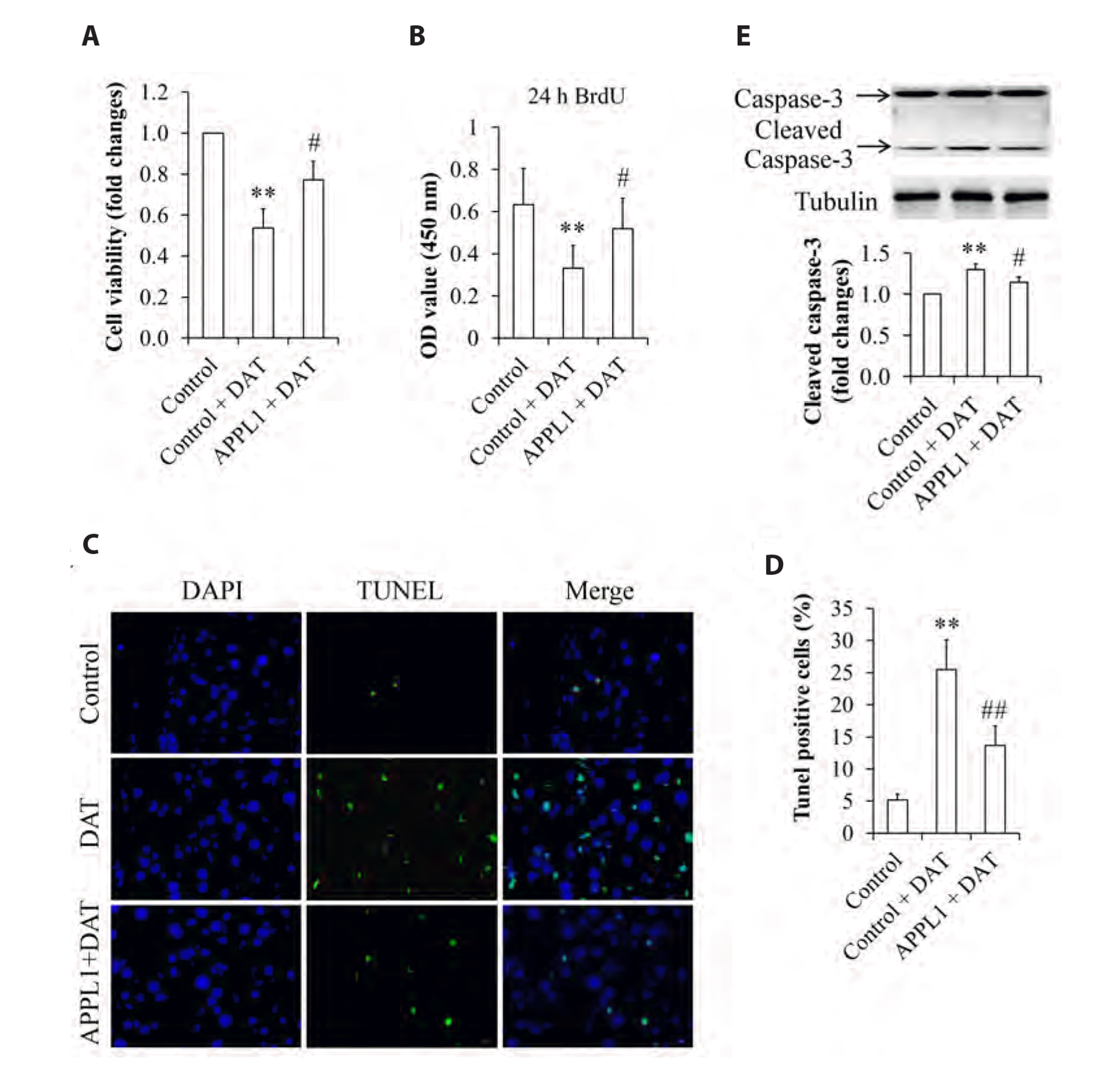
Fig. 8
A schematic diagram of mechanism underlying DAT action on HepG2 cell survival.
TRAF6 functions as an E3 ligase. In the present study, TRAF6-modulated K63-linked polyubiquitination of APPL1 mediates the inhibitory effects of DAT on the survival of HepG2 cells by downregulating pro-survival signaling pathways such as STAT3, Akt, and Erk1/2 pathways. DAT, diallyl trisulfide; TRAF6, TNF receptor-associated factor 6; K63, Lys 63; APPL1, adaptor protein, phosphotyrosine interacting with PH domain and leucine zipper 1; Ub, ubiquitin.
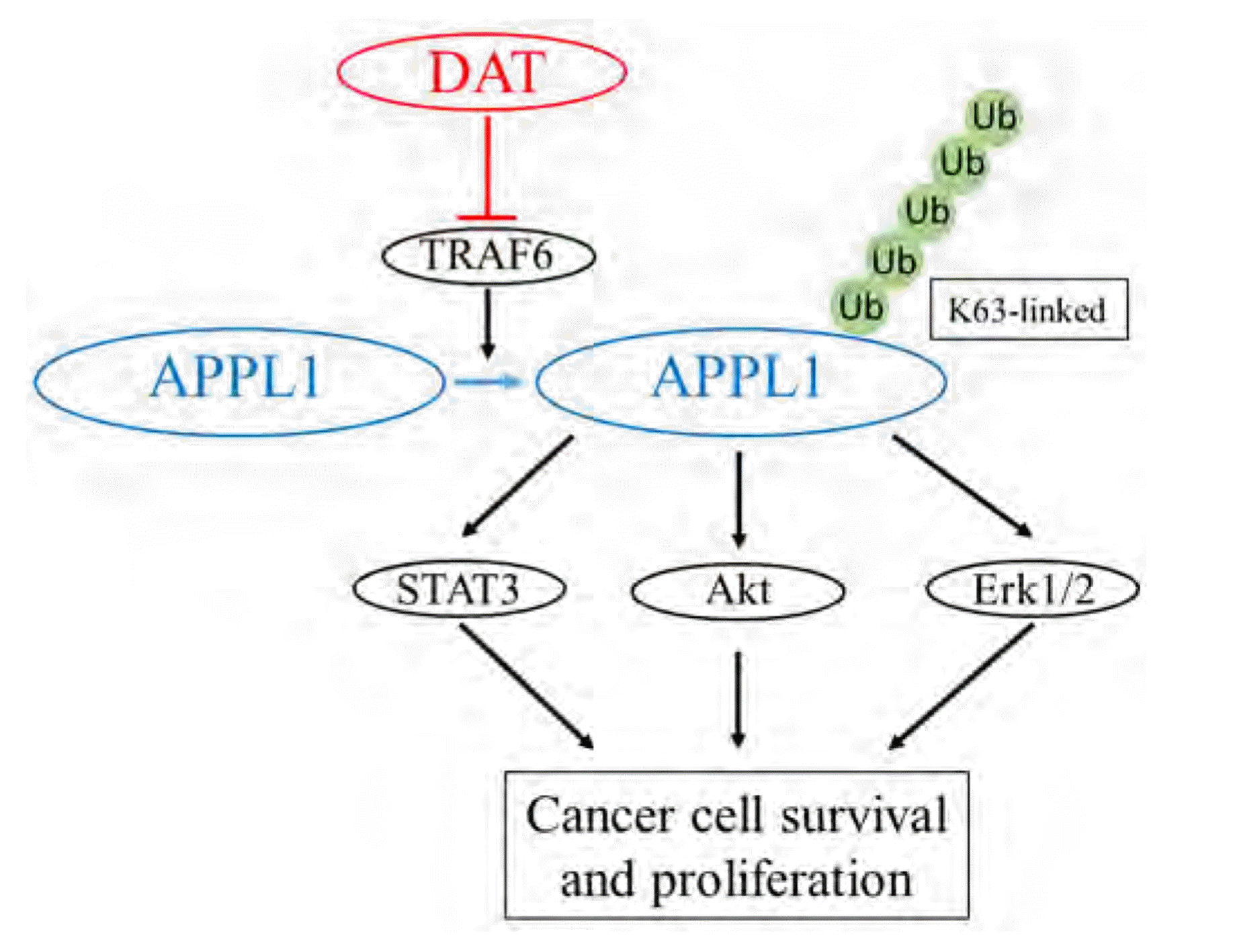