Abstract
The present study was carried out to investigate the effect of Arctigenin on cell growth and the mechanism of cell death elicited by Arctigenin were examined in FaDu human pharyngeal carcinoma cells. To determine the apoptotic activity of Arctigenin in FaDu human pharyngeal carcinoma cells, cell viability assay, DAPI staining, caspase activation analysis, and immunoblotting were performed. Arctigenin inhibited the growth of cells in a dose-dependent manner and induced nuclear condensation and fragmentation. Arctigenin-treated cells showed caspase-3/7 activation and increased apoptosis versus control cells. FasL, a death ligand associated with extrinsic apoptotic signaling pathways, was up-regulated by Arctigenin treatment. Moreover, caspase-8, a part of the extrinsic apoptotic pathway, was activated by Arctigenin treatments. Expressions of anti-apoptotic factors such as Bcl-2 and Bcl-xL, components of the mitochondria-dependent intrinsic apoptosis pathway, significantly decreased following Arctigenin treatment. The expressions of pro-apoptotic factors such as BAX, BAD and caspase-9, and tumor suppressor -53 increased by Arctigenin treatments. In addition, Arctigenin activated caspase-3 and poly (ADP-ribose) polymerase (PARP) induced cell death. Arctigenin also inhibited the proliferation of FaDu cells by the suppression of p38, NF-κB, and Akt signaling pathways. These results suggest that Arctigenin may inhibit cell proliferation and induce apoptotic cell death in FaDu human pharyngeal carcinoma cells through both the mitochondria-mediated intrinsic pathway and the death receptor-mediated extrinsic pathway.
Head and neck cancers such as oral cancer, pharyngeal cancer, and laryngeal cancer are the most common cancers worldwide, especially in South America, Asia, and Europe [1-3]. Also, most of them are squamous cell carcinoma [4]. In the last 30 years, the survival rate of head and neck cancer has not improved significantly despite modern medical techniques and treatments such as the use of anticancer drugs [3,5]. Clinical treatment for head and neck cancer may induce adverse effects related to functional changes in the ability to speak, swallow, or chew [4,6]. In addition, anticancer drug treatment may cause side effects such as gastrointestinal disorders, immune dysfunction, and bone marrow loss [7,8]. Therefore, there is a lot of interest in developing natural anticancer drugs that can minimize side effects while maintaining the effects of anticancer drugs [9].
Apoptosis is a regulatory strategy for the removal of unnecessary and potentially harmful cells from an organism. This process interacts with the development of many diseases and immune system abnormalities [10]. This process is very important for homeostasis preservation and physical development, as well as for preserving homeostasis, as well as for regulating cell growth and proliferation [11]. Most anticancer drugs act as chemotherapeutic agents for cancer by inhibiting the proliferation of cancer cells by inducing apoptosis [12,13]. Therefore, apoptosis of cancer cells due to the use of these anticancer drugs has become an important indicator of cancer treatment outcomes [14,15]. Analysis of apoptosis mechanisms is very valuable and promotes understanding of the pathogenesis of diseases caused by non-functional apoptosis [10]. In cancer, apoptosis can occur either through a death receptor-dependent extrinsic pathway or a mitochondrial-dependent intrinsic pathway induced by chemotherapy [16,17].
Over the past few decades, natural products have been used for many malignant diseases, such as cancer, and more and more studies have demonstrated the importance of phytochemicals in the treatment of these diseases [18].
Arctigenin was first identified in Arctium lappa L. (A. lappa L.), a popular medicinal herb and health supplement frequently used for anti-influenza treatment in Asia, especially China, Korea and Japan [19]. Arctigenin has a variety of therapeutic effects, including antiviral [20], immunomodulatory [21,22] anti-inflammatory [23,24], and anti-tumor activity [25,26].
Arctigenin is known to show anti-proliferative effects on cancer cells including prostate cancer, breast cancer and lung cancer [27,28]. However, Arctigenin effects on Head and neck cancers cells are not clearly established.
In this study, therefore, the effect of Arctigenin on cell growth and the mechanism of cell death elicited by Arctigenin were examined in FaDu human pharyngeal carcinoma cells. Our results showed that Arctigenin can inhibit cell viability and induce apoptosis in a dose‐dependent manner in FaDu human pharyngeal carcinoma cells.
Arctigenin (Fig. 1), 3-[4,5-dimethylthiazol-2-yl]-2,5-diphenyltetrazolium bromide (MTT) and 4´,6-diamidino-2-phenylindole dihydrochloride (DAPI) were purchased from Sigma-Aldrich Corp. (St. Louis, MO, USA). LIVE/DEAD Viability/Cytotoxicity kit was purchased from ThermoFisher Scientific, Inc. (Waltham, MA, USA). PhiPhiLux-G1D2 Caspase-3/7 Assay Kit were purchased from OncoImmunin Inc. (Gaithersburg, MD, USA). Anti-cleaved caspase-3, -8, -9, anti-Fas, anti-PARP, anti-Bcl-2, anti-Bcl-xL, anti-Bax, anti-Bad, anti-Phospho-ERK, anti-total-ERK, anti-phospho-p38, anti-total-p38, anti-phospho-JNK, anti-total-JNK, anti-phospho-NFκB, anti-total-NFκB, anti-phospho-AKT, anti-total-AKT, anti-p53 and anti-β-actin antibodies were supplied by Cell Signaling Technology, Inc. (Danvers, MA, USA).
L-929 murine fibroblast cell and FaDu human pharyngeal carcinoma cells obtained from the American Type Culture Collection (ATCC, Rockville, MD, USA). The FaDu cells were grown in minimum essential medium (MEM; WelGene, Daegu, Korea) containing 10% fetal bovine serum (FBS; WelGene) at 37°C in an atmosphere containing 5% CO2.
The L-929 cells and FaDu cells were seeded at a concentration of 3 × 104 cells/well in 48-well plates. After 24 h growth, the cells were treated with Arctigenin at various concentrations for 24 h. The cell viability test was evaluated using the MTT assay. At least 4 separate experiments were performed on each concentration combination.
The L-929 cells and FaDu cells (2 × 104 cells/well) were cultured in an 8-well chamber slide, and allowed to attach to the bottom of the chamber slide overnight. Thereafter, the cells were treated with 0, 50 or 100 μM Arctigenin for 24 h at 37°C and stained using the Live/Dead cell viability assay kit. The cells were imaged using a fluorescence microscope (Eclipse TE2000; Nikon Instruments, Melville, NY, USA). The Live/Dead cell viability assay kit uses green calcein acetoxymethyl ester (Calcein AM) to stain the live cells (green fluorescence) and ethidium homodimer1 to stain the dead cells (red fluorescence).
FaDu cells (2 × 104 cells/well) were cultured in an 8-well chamber slide, and allowed to attach to the bottom of the chamber slide overnight. After 24 h growth, the cells were treated with 0, 50 or 100 μM Arctigenin for 24 h. DAPI staining was done according to the previously described method [29]. The stained cells examined by fluorescent inverted microscopy (Eclipse TE2000; Nikon Instruments).
FaDu cells (2 × 104 cells/well) were cultured in an 8-well chamber slide, and allowed to attach to the bottom of the chamber slide overnight. After 24 h growth, the cells were treated with 0, 50 or 100 μM Arctigenin for 24 h. TUNEL assay was performed based on Manufacture’s protocol (Promega DeadEnd Colorimetric TUNEL System; Promega, Madison, WI, USA). The images were imaged using a Leica DM750 microscope (Leica Microsystems, Heerbrugg, Switzerland).
The activity of the apoptosis executioner caspase-3/-7 was investigated using the cell-permeable fluorogenic substrate PhiPhiLux-G1D2 according to the manufacturer's instructions and was and cells were imaged using a fluorescence microscope (Eclipse TE2000; Nikon Instruments).
The FaDu cells were treated with 0, 50 and 100 μM Arctigenin or 12.5 μM Z-VAD-fmk, a caspase-3 inhibitor (Sigma-Aldrich Corp.) for 24 h. Immunoblotting was done according to the previously described method with minor modifications [30]. The Anti-cleaved caspase-3, -8, -9, anti-Fas, anti-PARP, anti-Bcl-2, anti-Bcl-xL, anti-Bax, anti-Bad, anti-Phospho-ERK, anti-total-ERK, anti-phospho-p38, anti-total-p38, anti-phospho-JNK, anti-total-JNK, anti-phospho-NFκB, anti-total-NFκB, anti-phospho-AKT, anti-total-AKT, anti-p53 and anti-β-actin were used as the primary antibody. The immunoreactive bands were visualized using the ECL system (Sigma-Aldrich Corp.). And were visualized using a MicroChemi 4.2 imager (DNR Bioimaging Systems, Jerusalem, Israel).
All experiments were performed at least 4 times. The results were presented as mean ± standard deviation (SD). The statistical significance was analyzed by using Student’s t-test for two groups and one way analysis of variance for multi-group comparisons. All statistical analyses were performed using SPSS version 12.0 (SPSS Inc., Chicago, IL, USA). A p-value < 0.05 was considered statistically significant.
To verify the cytotoxic effects of Arctigenin on FaDu cells and L-929, the cells were treated with following various concentration of Arctigenin for 24 and 48 h. After incubation, the cell viability was assed using an MTT assy. As shown in Fig. 2A and B, the viability of L-929 murine fibroblast cells treated with 12.5, 25, 50, 100, and 200 μM Arctigenin were 86 ± 3.51, 82 ± 2.75, 81 ± 1.85, 74 ± 1.95, and 72 ± 1.70, respectively, compared to non-treated control (100 ± 2.99) for 24 h (Fig. 2A upper panel), and were 95 ± 3.12, 94 ± 3.29, 89 ± 3.48, 82 ± 3.48, and 74 ± 3.40, respectively, compared to non-treated control (100 ± 3.48) for 48 h (Fig. 2A lower panel). These data showed that Arctigenin had no significant effect on the cell viability of L-929 except at high concentrations (100 and 200 μM for 24 h, 200 μM for 48 h). In contrast, the viability rate of FaDu cells decreased significantly in response to Arctigenin in a dose-dependent manner for 24 and 48 h (Fig. 2B). Based on these results, the IC50 value for Arctigenin in FaDu cells was estimated to be about 85.76 μM for 24 h, and 81.26 μM for 48 h. To confirm the Arctigenin-induced cell cytotoxicity in FaDu cells, microscopy was used to visualize the live and dead cells stained with calcein-AM (green fluorescence) and ethidium homodimer 1 (red fluorescence), respectively. As shown in Fig. 3, the L-929 cells incubated with following concentration Arctigenin for 24 h were stained green due to the cleavage of the membrane permeable calcein-AM by the cytosolic esterase in living cells. Dead cells were stained red by ethidium bromide homodimer 1 and were observed to be a significant ratio in the FaDu human pharyngeal carcinoma cells that had been treated with Arctigenin (50, 100 μM) for 24 h. These data indicated that Arctigenin had cytotoxic effects specifically in FaDu human pharyngeal carcinoma cells.
To investigate the mechanism underlying Arctigenin-induced FaDu apoptosis, DAPI staining was performed to determine chromatin condensation in FaDu cells following treatment with 50 and 100 μM Arctigenin for 24 h. The number of FaDu carcinoma cells with condensed nuclei was significantly increased following Arctigenin treatment (Fig. 4A, B). To verify whether Arctigenin-induced FaDu cell death is mediated by apoptosis, caspase-3/7 activity was measured using the cell-permeable PhiPhiLux-G1D2. As shown in Fig. 4C, the activities of caspase-3/7 increased in FaDu cells treated with Arctigenin. Taken together, these results suggest that Arctigenin induces cell death through apoptosis signaling pathways in FaDu human pharyngeal cells.
To determine the cellular apoptotic pathways associated with Arctigenin-induced FaDu cell death, immunoblotting was performed. Fas, which is an apoptotic ligand that triggers the death receptor-dependent extrinsic apoptotic pathway in cancer cells, was induced by Arctigenin in FaDu cells (Fig. 5A). As shown in Fig. 5A, the expression level of cleaved caspase-8, the downstream target of pro-apoptotic factor Fas, increased following Arctigenin. These data suggest that the involvement of the extrinsic death receptor-mediated apoptosis pathway in Arctigenin-induced FaDu cell apoptosis.
The expression levels of Bcl-2 and Bcl-xL, anti-apoptotic factors associated with the intrinsic mitochondria-dependent apoptosis pathway, were downregulated by Arctigenin in FaDu cells, while those of mitochondria-dependent pro-apoptotic factors such as Bax and Bad were upregulated by Arctigenin in FaDu cells (Fig. 5B). And, Arctigenin treatment increased the expression level of cleaved caspase-9 in FaDu cells (Fig. 5B). These data show that Arctigenin-induced FaDu cell death involves the intrinsic mitochondria-dependent apoptosis pathway.
Both cleaved caspase-8 and caspase-9, acted in the extrinsic death receptor-mediated and intrinsic mitochondria-dependent apoptosis pathways in FaDu cells after Arctigenin treatment, following to expression of cleaved caspase-3 (Fig. 5C). These results suggest that Arctigenin induces FaDu cell apoptosis, which is mediated by death receptor-mediated extrinsic and mitochondria-dependent intrinsic apoptosis through activation of the caspase cascade in FaDu pharyngeal carcinoma cells.
Apoptotic signaling pathways are mediated by the activation of the caspase cascade, a hallmark of apoptosis. FaDu cells were pretreated for 60 min with 12.5 μM Z-VAD-fmk, a pan-caspase inhibitor, prior to treatment with 100 μM Arctigenin. MTT assays were then performed to measure cell viability, and western blotting was used to observe changes in the expression of caspase-3 and PARP. As shown in Fig. 6A, 100 μM Arctigenin decreased the viability of FaDu cells by ~51.6% compared with that in untreated control cells. However, the viability of FaDu cells was partially recovered (by ~78.4%) in the presence of Z-VAD-fmk and Arctigenin. Furthermore, the Arctigenin-induced upregulation of cleaved caspase-3 was decreased by Z-VAD-fmk in FaDu cells. Subsequently, Z-VAD-fmk significantly suppressed Arctigenininduced cleavage of the pro-form of PARP in FaDu cells (Fig. 6B). Therefore, these data indicated that Arctigenin-induced apoptosis in FaDu cells was regulated by the activation of caspases.
To verify the signaling pathways involved in the Arctigenin-induced apoptosis of FaDu carcinoma cells, we investigated the activation of mitogen activated protein kinase (MAPK) subgroups such as ERK1/2, p38 and NF-κB protein expression in response to Arctigenin. Arctigenin induce the phosphorylation of ERK1/2, p38 and NF-κB decreased in the FaDu cells treated with Arctigenin in a dose-dependent manner compared with non-treated control (Fig. 7). Furthermore, the phosphorylation of Akt, which is closely associated with cellular signaling of cell proliferation, significantly decreased in FaDu cells treated with Artigenin, in a dose-dependent manner compared with non-treated control. Therefore, these data indicate that Arctigenin induced suppression of proliferation is associated with the alteration of ERK1/2, p38 MAPK, NF-κB and PI3K/Akt cellular signaling pathways in FaDu pharyngeal carcinoma cells.
In the present study, the results suggested that Arctigenin-induced FaDu pharyngeal carcinoma cell death is mediated by caspases cascade via death receptor-mediated extrinsic and mitochondria-dependent intrinsic apoptosis pathways. Furthermore, Arctigenin-induced FaDu pharyngeal carcinoma cell death is involved in the alteration of ERK1/2, p38 MAPK, NF-κB, and PI3K/Akt cellular signaling pathways.
Over the past few decades, natural products have been used for many malignant diseases, such as cancer, and more and more studies have demonstrated the importance of phytochemicals in the treatment of these diseases. Although combination chemotherapy is currently being considered as the primary clinical treatment for various cancer cases, this approach is limited by clinical side effects such as high toxicity and drug resistance [31]. Therefore, in an attempt to develop chemotherapeutic agents with fewer side effects, the anticancer activity and cellular mechanisms of natural compounds isolated from medicinal plants used in orthodox traditional medicine are being investigated for various cancer types [32]. So, we investigated whether Arctigenin induces apoptosis in FaDu pharyngeal carcinoma cells and elucidated the cellular mechanism underlying its activity in the present study.
Candidate chemotherapeutic compounds should induce highly specific cancer cell death as well as have low cytotoxicity in normal cells. L-929 is an adherent type of mouse fibroblast cell line was known as an alternate test system for toxicity assessment [33]. In our cell viability (Fig. 2) and Live/Dead cell assay (Fig. 3), Arctigenin did not show significant toxicity in L-929 an adherent type of mouse fibroblast cell line. However, the cell viability of FaDu pharyngeal carcinoma cells gradually decreased by Arctigenin in a dose-dependent manner. Treatment with 50 and 100 μM Arctigenin decreased FaDu cell viability by 60% and 40%, respectively, compared to the non-treated control. The IC50 value of Arctigenin in FaDu cells was estimated at approximately 85 μM, a lower IC50 value than the 200 μM IC50 reported for the glioma U87MG cell lines [34]. However, IC50 values of Arctigenin was estimated at various dose ranges in other cancer cells. For example, Yang et al. [35], reported that IC50 values of Arctigenin were estimated approximately 67.8 μM bladder cancer cell T24. Therefore, these show that IC50 value of Arctigenin can be depend on the cell specificity.
To confirm that Arctigenin had pharyngeal cancer cell-specific cytotoxic effects, we performed a cell survival assay (Fig. 3). In the L-929 cells stimulated with 100 μM Arctigenin for 24 h, almost all of cells were stained green by the membrane-permeable calcein green AM, which is cleaved by esterase in living cells to produce cytoplasmic green fluorescence. In contrast, 50 and 100 μM Arctigenin decreased the total number of FaDu pharyngeal carcinoma cells and increased the number of cells stained red by ethidium homodimer-1, a marker of membrane-impermeable cell death. Taken together, these dates indicate that Arctigenin inhibits cancer cell-specific cytotoxicity and has fewer side effects as a potential chemotherapeutic agent.
Subsequently, to elucidate the mechanism by which Arctigenin induces FaDu pharyngeal carcinoma cell death, DAPI staining was performed to investigate alterations in chromatin condensation (Fig. 4A). The number of FaDu pharyngeal carcinoma cells with condensed chromatin gradually increased in response to Arctigenin in a dose-dependent manner. Chromatin condensation is a hallmark of apoptosis [36]. Therefore, these results suggested that Arctigenin induced the apoptosis of FaDu pharyngeal carcinoma cells. Activation of caspases is a key function of apoptotic signaling pathways [37]. Therefore, to confirm that Arctigenin-induced apoptosis is mediated by caspase activation, we examined a caspase-3/-7 activation assay in FaDu cells treated with Arctigenin by using PhiPhiLux, a fluorogenic caspase substrate (Fig. 4B). As shown in Fig. 4B, the expression of caspase-3/-7 activation was higher in FaDu cells treated with 50 μM and 100 μM Arctigenin than in non-treated control cells.
Apoptosis is mainly regulated by two general pathways: intrinsic (mitochondrial) pathway and/or extrinsic (death receptor) pathway [26]. Fas, an important regulator of apoptosis, binds to the receptor FasR across the surface of the target cell, and then initiates the death receptor-mediated extrinsic apoptotic pathway through activation of caspase-8, -3 and PARP [38]. In the intrinsic pathway, mitochondrial outer membrane permeabilization is initiated by signals originating internally [39]. Anti-apoptotic mitochondrial proteins such as Bcl-2 and Bcl-xL, pro-apoptotic proteins such as Bax and Bad are important regulators of cytochrome c release in mitochondria [40,41]. Han et al. [42], reported that Arctigenin activates apoptosis though the mitochondrial pathway, which participates in the initiation of caspase-3 and caspase-9 followed by the cleavage of poly-ADP-ribose polymerase (PARP). In addition, Bcl-2 and Bcl-xl levels were decreased and Bax increased in Arctigenin-treated (5, 50 μM) colorectal cancer CT26 cells. Similarly, in our study, Arctigenin treatment increased the levels of Bax and Bad protein expressions, but decreased the levels of Bcl-2 and Bcl-xl protein expressions in FaDu pharyngeal carcinoma cells (Fig. 5B). Changes in the levels of these anti- and pro-apoptotic factors associated with the mitochondria-dependent intrinsic pathway subsequently induced the activation cascade of caspase-9, caspase-3 and PARP in FaDu cells treated with Arctigenin (Figs. 5, 6). These results indicate that Arctigenin induces apoptosis in FaDu pharyngeal carcinoma cells involving the death receptor- and mitochondrial-signal transduction pathways.
The p38-MAPK signaling pathway is involved in a variety of cellular responses, and the outcomes of cellular responses are diverse and complex. The involvement of p38-MAPK in apoptosis is also variable [43]. It is known that p38-MAPK signaling promotes apoptosis [44]. To verify the signaling pathways involved in the Arctigenin-induced apoptosis of FaDu carcinoma cells, we investigated the activation of mitogen activated protein kinase (MAPK) subgroups such as ERK1/2, p38 and NF-κB protein expression in response to Arctigenin. Arctigenin induce the phosphorylation of ERK1/2, p38 and NF-κB decreased in the FaDu cells treated with Arctigenin in a dose-dependent manner compared with non-treated control (Fig. 7). Therefore, these data indicate that Arctigenin induced suppression of proliferation is associated with the alteration of ERK1/2, p38 MAPK, NF-κB and PI3K/Akt cellular signaling pathways in FaDu pharyngeal carcinoma cells. In the present study, we demonstrated that Arctigenin-induced cell death in FaDu pharyngeal carcinoma cells depended on the activation of caspases involved in both intrinsic and extrinsic apoptotic pathways. Furthermore, Arctigenin induce apoptosis via the p38 MAPK pathway in FaDu pharyngeal carcinoma cell. Taken together, these findings suggest that Arctigenin a potential chemotherapeutic compounds can be used for the management of pharyngeal carcinoma.
REFERENCES
1. Warnakulasuriya S. 2009; Global epidemiology of oral and oropharyngeal cancer. Oral Oncol. 45:309–316. DOI: 10.1016/j.oraloncology.2008.06.002. PMID: 18804401. PMID: https://www.scopus.com/inward/record.uri?partnerID=HzOxMe3b&scp=67349186933&origin=inward.


2. Kalavrezos N, Scully C. 2015; Mouth cancer for clinicians. Part 2: epidemiology. Dent Update. 42:354–356. 358–359. DOI: 10.12968/denu.2015.42.4.354. PMID: 26062260.


3. Sun F, Li D, Wang C, Peng C, Zheng H, Wang X. 2019; Acacetin-induced cell apoptosis in head and neck squamous cell carcinoma cells: evidence for the role of muscarinic M3 receptor. Phytother Res. 33:1551–1561. DOI: 10.1002/ptr.6343. PMID: 31066474. PMID: https://www.scopus.com/inward/record.uri?partnerID=HzOxMe3b&scp=85065512110&origin=inward.


4. Rettig EM, D'Souza G. 2015; Epidemiology of head and neck cancer. Surg Oncol Clin N Am. 24:379–396. DOI: 10.1016/j.soc.2015.03.001. PMID: 25979389. PMID: https://www.scopus.com/inward/record.uri?partnerID=HzOxMe3b&scp=84929504129&origin=inward.


5. Pezzuto F, Buonaguro L, Caponigro F, Ionna F, Starita N, Annunziata C, Buonaguro FM, Tornesello ML. 2015; Update on head and neck cancer: current knowledge on epidemiology, risk factors, molecular features and novel therapies. Oncology. 89:125–136. DOI: 10.1159/000381717. PMID: 25967534. PMID: https://www.scopus.com/inward/record.uri?partnerID=HzOxMe3b&scp=84942190711&origin=inward.


6. Kundu SK, Nestor M. 2012; Targeted therapy in head and neck cancer. Tumour Biol. 33:707–721. DOI: 10.1007/s13277-012-0350-2. PMID: 22373581. PMID: https://www.scopus.com/inward/record.uri?partnerID=HzOxMe3b&scp=84863587948&origin=inward.


7. Sacks PG. 1996; Cell, tissue and organ culture as in vitro models to study the biology of squamous cell carcinomas of the head and neck. Cancer Metastasis Rev. 15:27–51. DOI: 10.1007/BF00049486. PMID: 8842478. PMID: https://www.scopus.com/inward/record.uri?partnerID=HzOxMe3b&scp=0029929233&origin=inward.


8. Todd R, Donoff RB, Wong DT. 1997; The molecular biology of oral carcinogenesis: toward a tumor progression model. J Oral Maxillofac Surg. 55:613–623. discussion 623–625. DOI: 10.1016/S0278-2391(97)90495-X. PMID: 9191644. PMID: https://www.scopus.com/inward/record.uri?partnerID=HzOxMe3b&scp=0030926441&origin=inward.


9. Links M, Lewis C. 1999; Chemoprotectants: a review of their clinical pharmacology and therapeutic efficacy. Drugs. 57:293–308. DOI: 10.2165/00003495-199957030-00003. PMID: 10193684. PMID: https://www.scopus.com/inward/record.uri?partnerID=HzOxMe3b&scp=0033014520&origin=inward.
10. Goldar S, Khaniani MS, Derakhshan SM, Baradaran B. 2015; Molecular mechanisms of apoptosis and roles in cancer development and treatment. Asian Pac J Cancer Prev. 16:2129–2144. DOI: 10.7314/APJCP.2015.16.6.2129. PMID: 25824729. PMID: https://www.scopus.com/inward/record.uri?partnerID=HzOxMe3b&scp=84929224850&origin=inward.


11. Rahman MA, Hannan MA, Dash R, Rahman MH, Islam R, Uddin MJ, Sohag AAM, Rahman MH, Rhim H. 2021; Phytochemicals as a complement to cancer chemotherapy: pharmacological modulation of the autophagy-apoptosis pathway. Front Pharmacol. 12:639628. DOI: 10.3389/fphar.2021.639628. PMID: 34025409. PMCID: PMC8138161. PMID: 8e76401c7d1f4cf1b752c609c09dc5db. PMID: https://www.scopus.com/inward/record.uri?partnerID=HzOxMe3b&scp=85106167533&origin=inward.


12. Sak K. 2012; Chemotherapy and dietary phytochemical agents. Chemother Res Pract. 2012:282570. DOI: 10.1155/2012/282570. PMID: 23320169. PMCID: PMC3539428.


13. Alfarouk KO, Stock CM, Taylor S, Walsh M, Muddathir AK, Verduzco D, Bashir AH, Mohammed OY, Elhassan GO, Harguindey S, Reshkin SJ, Ibrahim ME, Rauch C. 2015; Resistance to cancer chemotherapy: failure in drug response from ADME to P-gp. Cancer Cell Int. 15:71. DOI: 10.1186/s12935-015-0221-1. PMID: 26180516. PMCID: PMC4502609. PMID: https://www.scopus.com/inward/record.uri?partnerID=HzOxMe3b&scp=84937028711&origin=inward.


14. Tompkins KD, Thorburn A. 2019; Regulation of apoptosis by autophagy to enhance cancer therapy. Yale J Biol Med. 92:707–718. PMID: 31866785. PMCID: PMC6913805. PMID: https://www.scopus.com/inward/record.uri?partnerID=HzOxMe3b&scp=85077163064&origin=inward.
15. Wang X, Zhang H, Chen X. 2019; Drug resistance and combating drug resistance in cancer. Cancer Drug Resist. 2:141–160. DOI: 10.20517/cdr.2019.10. PMID: 34322663. PMCID: PMC8315569. PMID: https://www.scopus.com/inward/record.uri?partnerID=HzOxMe3b&scp=85103071967&origin=inward.


16. Kaufmann SH, Earnshaw WC. 2000; Induction of apoptosis by cancer chemotherapy. Exp Cell Res. 256:42–49. DOI: 10.1006/excr.2000.4838. PMID: 10739650. PMID: https://www.scopus.com/inward/record.uri?partnerID=HzOxMe3b&scp=0034630167&origin=inward.


17. Reed JC. 2001; Apoptosis-regulating proteins as targets for drug discovery. Trends Mol Med. 7:314–319. DOI: 10.1016/S1471-4914(01)02026-3. PMID: 11425640. PMID: https://www.scopus.com/inward/record.uri?partnerID=HzOxMe3b&scp=0034953632&origin=inward.


18. Punia R, Raina K, Agarwal R, Singh RP. 2017; Acacetin enhances the therapeutic efficacy of doxorubicin in non-small-cell lung carcinoma cells. PLoS One. 12:e0182870. DOI: 10.1371/journal.pone.0182870. PMID: 28859099. PMCID: PMC5578506. PMID: https://www.scopus.com/inward/record.uri?partnerID=HzOxMe3b&scp=85029188611&origin=inward.


19. Gao Q, Yang M, Zuo Z. 2018; Overview of the anti-inflammatory effects, pharmacokinetic properties and clinical efficacies of arctigenin and arctiin from Arctium lappa L. Acta Pharmacol Sin. 39:787–801. DOI: 10.1038/aps.2018.32. PMID: 29698388. PMCID: PMC5943914. PMID: https://www.scopus.com/inward/record.uri?partnerID=HzOxMe3b&scp=85046671767&origin=inward.


20. Yang Z, Liu N, Huang B, Wang Y, Hu Y, Zhu Y. 2005; Effect of anti-influenza virus of Arctigenin in vivo. Zhong Yao Cai. 28:1012–1014. Chinese. PMID: 16514891. PMID: https://www.scopus.com/inward/record.uri?partnerID=HzOxMe3b&scp=33748874508&origin=inward.
21. Tsai WJ, Chang CT, Wang GJ, Lee TH, Chang SF, Lu SC, Kuo YC. 2011; Arctigenin from Arctium lappa inhibits interleukin-2 and interferon gene expression in primary human T lymphocytes. Chin Med. 6:12. DOI: 10.1186/1749-8546-6-12. PMID: 21435270. PMCID: PMC3076299. PMID: https://www.scopus.com/inward/record.uri?partnerID=HzOxMe3b&scp=79953092808&origin=inward.


22. Yao X, Li G, Lü C, Xu H, Yin Z. 2012; Arctigenin promotes degradation of inducible nitric oxide synthase through CHIP-associated proteasome pathway and suppresses its enzyme activity. Int Immunopharmacol. 14:138–144. DOI: 10.1016/j.intimp.2012.06.017. PMID: 22770942. PMID: https://www.scopus.com/inward/record.uri?partnerID=HzOxMe3b&scp=84864075049&origin=inward.


23. Lee JY, Kim CJ. 2010; Arctigenin, a phenylpropanoid dibenzylbutyrolactone lignan, inhibits type I-IV allergic inflammation and pro-inflammatory enzymes. Arch Pharm Res. 33:947–957. DOI: 10.1007/s12272-010-0619-1. PMID: 20607501. PMID: https://www.scopus.com/inward/record.uri?partnerID=HzOxMe3b&scp=77954485451&origin=inward.


24. Kang HS, Lee JY, Kim CJ. 2008; Anti-inflammatory activity of arctigenin from Forsythiae Fructus. J Ethnopharmacol. 116:305–312. DOI: 10.1016/j.jep.2007.11.030. PMID: 18180122. PMID: https://www.scopus.com/inward/record.uri?partnerID=HzOxMe3b&scp=39149122828&origin=inward.


25. Lu Z, Cao S, Zhou H, Hua L, Zhang S, Cao J. 2015; Mechanism of arctigenin-induced specific cytotoxicity against human hepatocellular carcinoma cell lines: Hep G2 and SMMC7721. PLoS One. 10:e0125727. DOI: 10.1371/journal.pone.0125727. PMID: 25933104. PMCID: PMC4416797. PMID: https://www.scopus.com/inward/record.uri?partnerID=HzOxMe3b&scp=84928776796&origin=inward.


26. He Y, Fan Q, Cai T, Huang W, Xie X, Wen Y, Shi Z. 2018; Molecular mechanisms of the action of arctigenin in cancer. Biomed Pharmacother. 108:403–407. DOI: 10.1016/j.biopha.2018.08.158. PMID: 30236849. PMID: https://www.scopus.com/inward/record.uri?partnerID=HzOxMe3b&scp=85053399921&origin=inward.


27. Wang HQ, Jin JJ, Wang J. 2014; Arctigenin enhances chemosensitivity to cisplatin in human nonsmall lung cancer H460 cells through downregulation of survivin expression. J Biochem Mol Toxicol. 28:39–45. DOI: 10.1002/jbt.21533. PMID: 24395429. PMID: https://www.scopus.com/inward/record.uri?partnerID=HzOxMe3b&scp=84891632136&origin=inward.


28. Maxwell T, Chun SY, Lee KS, Kim S, Nam KS. 2017; The anti-metastatic effects of the phytoestrogen arctigenin on human breast cancer cell lines regardless of the status of ER expression. Int J Oncol. 50:727–735. DOI: 10.3892/ijo.2016.3825. PMID: 28035371. PMID: https://www.scopus.com/inward/record.uri?partnerID=HzOxMe3b&scp=85013040741&origin=inward.


29. Kim JS, Cho IA, Kang KR, Lim H, Kim TH, Yu SK, Kim HJ, Lee SA, Moon SM, Chun HS, Kim CS, Kim DK. 2019; Reversine induces caspase-dependent apoptosis of human osteosarcoma cells through extrinsic and intrinsic apoptotic signaling pathways. Genes Genomics. 41:657–665. DOI: 10.1007/s13258-019-00790-1. PMID: 30953339. PMID: https://www.scopus.com/inward/record.uri?partnerID=HzOxMe3b&scp=85064266609&origin=inward.


30. Park MG, Kim JS, Park SY, Lee SA, Kim HJ, Kim CS, Kim JS, Chun HS, Park JC, Kim DK. 2014; MicroRNA-27 promotes the differentiation of odontoblastic cell by targeting APC and activating Wnt/β-catenin signaling. Gene. 538:266–272. DOI: 10.1016/j.gene.2014.01.045. PMID: 24487055. PMID: https://www.scopus.com/inward/record.uri?partnerID=HzOxMe3b&scp=84893935584&origin=inward.


31. Hadden JW. 1997; The immunopharmacology of head and neck cancer: an update. Int J Immunopharmacol. 19:629–644. DOI: 10.1016/S0192-0561(97)00063-5. PMID: 9669203. PMID: https://www.scopus.com/inward/record.uri?partnerID=HzOxMe3b&scp=0344447055&origin=inward.


32. Hensley P, Mishra M, Kyprianou N. 2013; Targeting caspases in cancer therapeutics. Biol Chem. 394:831–843. DOI: 10.1515/hsz-2013-0128. PMID: 23509217. PMCID: PMC3721733. PMID: https://www.scopus.com/inward/record.uri?partnerID=HzOxMe3b&scp=84881177777&origin=inward.


33. Ray RS, Agrawal N, Sharma A, Hans RK. 2008; Use of L-929 cell line for phototoxicity assessment. Toxicol In Vitro. 22:1775–1781. DOI: 10.1016/j.tiv.2008.06.015. PMID: 18657604. PMID: https://www.scopus.com/inward/record.uri?partnerID=HzOxMe3b&scp=51749101270&origin=inward.


34. Jiang Y, Liu J, Hong W, Fei X, Liu R. 2020; Arctigenin inhibits glioblastoma proliferation through the AKT/mTOR pathway and induces autophagy. Biomed Res Int. 2020:3542613. DOI: 10.1155/2020/3542613. PMID: 33015162. PMCID: PMC7512051. PMID: https://www.scopus.com/inward/record.uri?partnerID=HzOxMe3b&scp=85092488454&origin=inward.


35. Yang S, Ma J, Xiao J, Lv X, Li X, Yang H, Liu Y, Feng S, Zhang Y. 2012; Arctigenin anti-tumor activity in bladder cancer T24 cell line through induction of cell-cycle arrest and apoptosis. Anat Rec (Hoboken). 295:1260–1266. DOI: 10.1002/ar.22497. PMID: 22619087. PMID: https://www.scopus.com/inward/record.uri?partnerID=HzOxMe3b&scp=84863549883&origin=inward.
36. Toné S, Sugimoto K, Tanda K, Suda T, Uehira K, Kanouchi H, Samejima K, Minatogawa Y, Earnshaw WC. 2007; Three distinct stages of apoptotic nuclear condensation revealed by time-lapse imaging, biochemical and electron microscopy analysis of cell-free apoptosis. Exp Cell Res. 313:3635–3644. DOI: 10.1016/j.yexcr.2007.06.018. PMID: 17643424. PMCID: PMC2705844. PMID: https://www.scopus.com/inward/record.uri?partnerID=HzOxMe3b&scp=34548696434&origin=inward.


37. Creagh EM, Conroy H, Martin SJ. 2003; Caspase-activation pathways in apoptosis and immunity. Immunol Rev. 193:10–21. DOI: 10.1034/j.1600-065X.2003.00048.x. PMID: 12752666. PMID: https://www.scopus.com/inward/record.uri?partnerID=HzOxMe3b&scp=0037833742&origin=inward.


38. Herrnring C, Reimer T, Jeschke U, Makovitzky J, Krüger K, Gerber B, Kabelitz D, Friese K. 2000; Expression of the apoptosis-inducing ligands FasL and TRAIL in malignant and benign human breast tumors. Histochem Cell Biol. 113:189–194. DOI: 10.1007/s004180050438. PMID: 10817673. PMID: https://www.scopus.com/inward/record.uri?partnerID=HzOxMe3b&scp=0034036052&origin=inward.


39. Kluck RM, Martin SJ, Hoffman BM, Zhou JS, Green DR, Newmeyer DD. 1997; Cytochrome c activation of CPP32-like proteolysis plays a critical role in a Xenopus cell-free apoptosis system. EMBO J. 16:4639–4649. DOI: 10.1093/emboj/16.15.4639. PMID: 9303308. PMCID: PMC1170090. PMID: https://www.scopus.com/inward/record.uri?partnerID=HzOxMe3b&scp=0030741528&origin=inward.


40. Kroemer G, Reed JC. 2000; Mitochondrial control of cell death. Nat Med. 6:513–519. DOI: 10.1038/74994. PMID: 10802706. PMID: https://www.scopus.com/inward/record.uri?partnerID=HzOxMe3b&scp=0034068601&origin=inward.


41. Wei MC, Zong WX, Cheng EH, Lindsten T, Panoutsakopoulou V, Ross AJ, Roth KA, MacGregor GR, Thompson CB, Korsmeyer SJ. 2001; Proapoptotic BAX and BAK: a requisite gateway to mitochondrial dysfunction and death. Science. 292:727–730. DOI: 10.1126/science.1059108. PMID: 11326099. PMCID: PMC3049805. PMID: https://www.scopus.com/inward/record.uri?partnerID=HzOxMe3b&scp=0035957653&origin=inward.


42. Han YH, Kee JY, Kim DS, Mun JG, Jeong MY, Park SH, Choi BM, Park SJ, Kim HJ, Um JY, Hong SH. 2016; Arctigenin inhibits lung metastasis of colorectal cancer by regulating cell viability and metastatic phenotypes. Molecules. 21:1135. DOI: 10.3390/molecules21091135. PMID: 27618887. PMCID: PMC6272973. PMID: https://www.scopus.com/inward/record.uri?partnerID=HzOxMe3b&scp=84987858588&origin=inward.


43. Yue J, López JM. 2020; Understanding MAPK signaling pathways in apoptosis. Int J Mol Sci. 21:2346. DOI: 10.3390/ijms21072346. PMID: 32231094. PMCID: PMC7177758. PMID: ea6f3fdf31194ddd9c6b405921186f76. PMID: https://www.scopus.com/inward/record.uri?partnerID=HzOxMe3b&scp=85082791079&origin=inward.


44. Royuela M, Arenas MI, Bethencourt FR, Sánchez-Chapado M, Fraile B, Paniagua R. 2002; Regulation of proliferation/apoptosis equilibrium by mitogen-activated protein kinases in normal, hyperplastic, and carcinomatous human prostate. Hum Pathol. 33:299–306. DOI: 10.1053/hupa.2002.32227. PMID: 11979370. PMID: https://www.scopus.com/inward/record.uri?partnerID=HzOxMe3b&scp=0036235788&origin=inward.


Fig. 2
Effect of Arctigenin on cell viability in FaDu pharyngeal carcinoma cells.
The effect of Arctigenin on the viability of L-929 cells (A) and FaDu cells (B) was accessed by the MTT assay. The percentage of cell viability was calculated as a ratio of A570nms of Arctigenin treated with cells and untreated control cells. Each data point represents the mean ± SEM of four experiments. MTT, 3-[4,5-dimethylthiazol-2-yl]-2,5-diphenyltetrazolium bromide. **p < 0.01 vs. control and ***p < 0.001 vs. control (the control cells measured in the absence of Arctigenin).
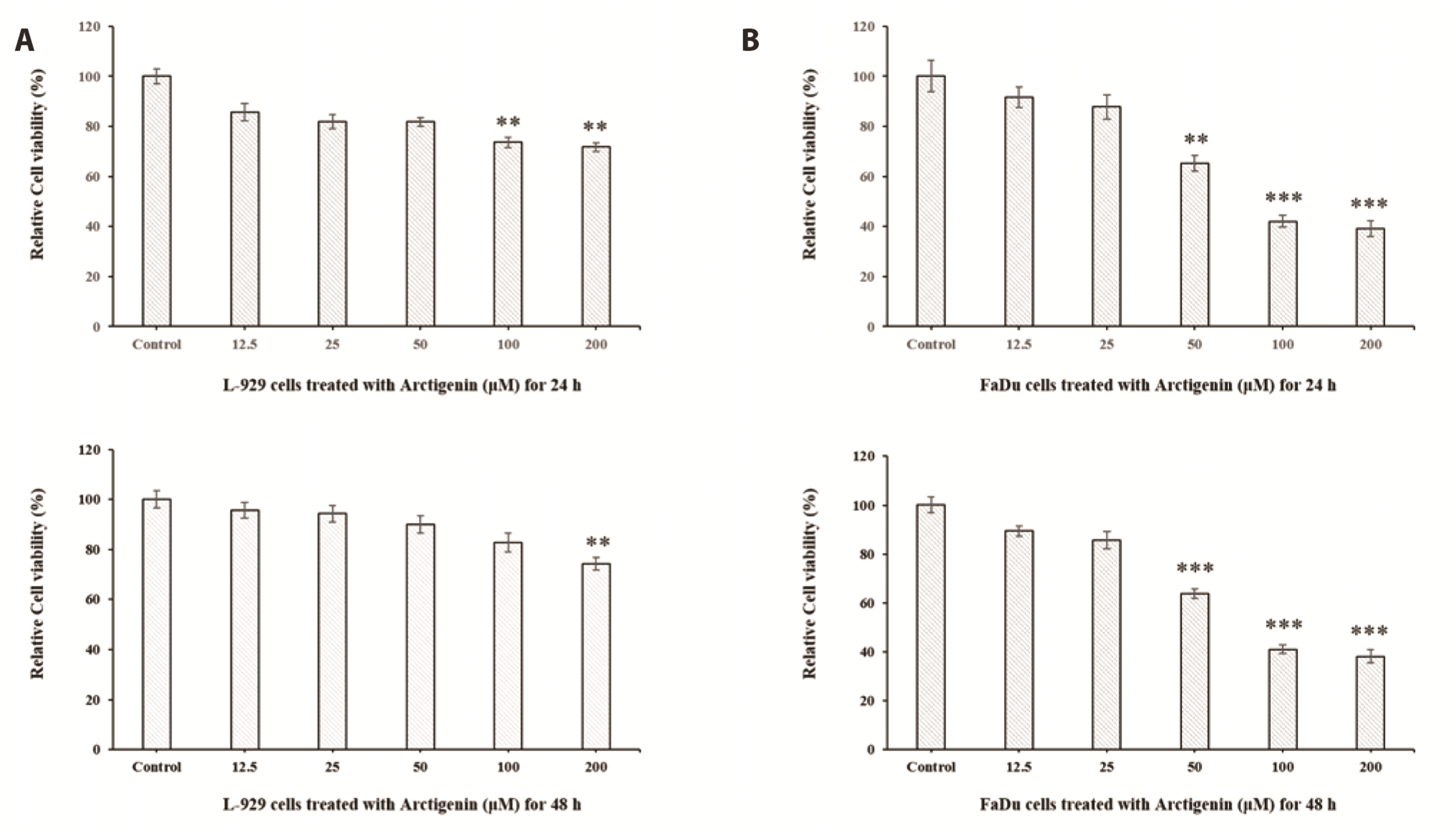
Fig. 3
Induction of cell death by Arctigenin.
(A) L-929 cells and FaDu cell death by Arctigenin. The cells were treated with 0, 50, or 100 μM Arctigenin for 24 h. Arctigenin induced the death of FaDu cells in the dose-dependent manner. FaDu cells emitting green fluorescence are live cells stained by green calcein AM, whereas cells emitting red fluorescence are dead cells stained by ethidium homodimer-1. The arrows indicate dead cells stained red. Scale bar represents 100 µm. (B) Quantitative data of (A) were counted, and calculated as 100%. Each data point represents the mean ± SEM of four experiments. **p < 0.01 vs. control and ***p < 0.001 vs. control (the control cells measured in the absence of Arctigenin).

Fig. 4
Apoptotic phenomenon in FaDu pharyngeal carcinoma cells stimulated with Arctigenin.
(A) Changes in nuclear morphology by Arctigenin. The cells were treated with 0, 50, 100 μM Arctigenin for 24 h. DAPI staining revealed that the number of FaDu cells with nuclear condensation was increased by Arctigenin. The arrows indicated the apoptotic cells with chromatic condensation characteristics. Scale bar represents 100 µm. (B) TUNEL assay revealed that the number of FaDu cells with apoptotic nuclei are stained dark brown was increased by Arctigenin. The arrows indicate apoptotic nuclei stained dark brown. Scale bar represents 100 µm. (C) Arctigenin induces the caspase-3/-7 activation in FaDu cells. The caspase-3/-7 intracellular activity assay was performed using PhiPhiLux-caspase-3/-7 substrate. Images were observed by fluorescence microscopy (Eclipse TE 2000; Nikon Instruments, Melville, NY, USA). Scale bar represents 100 µm.
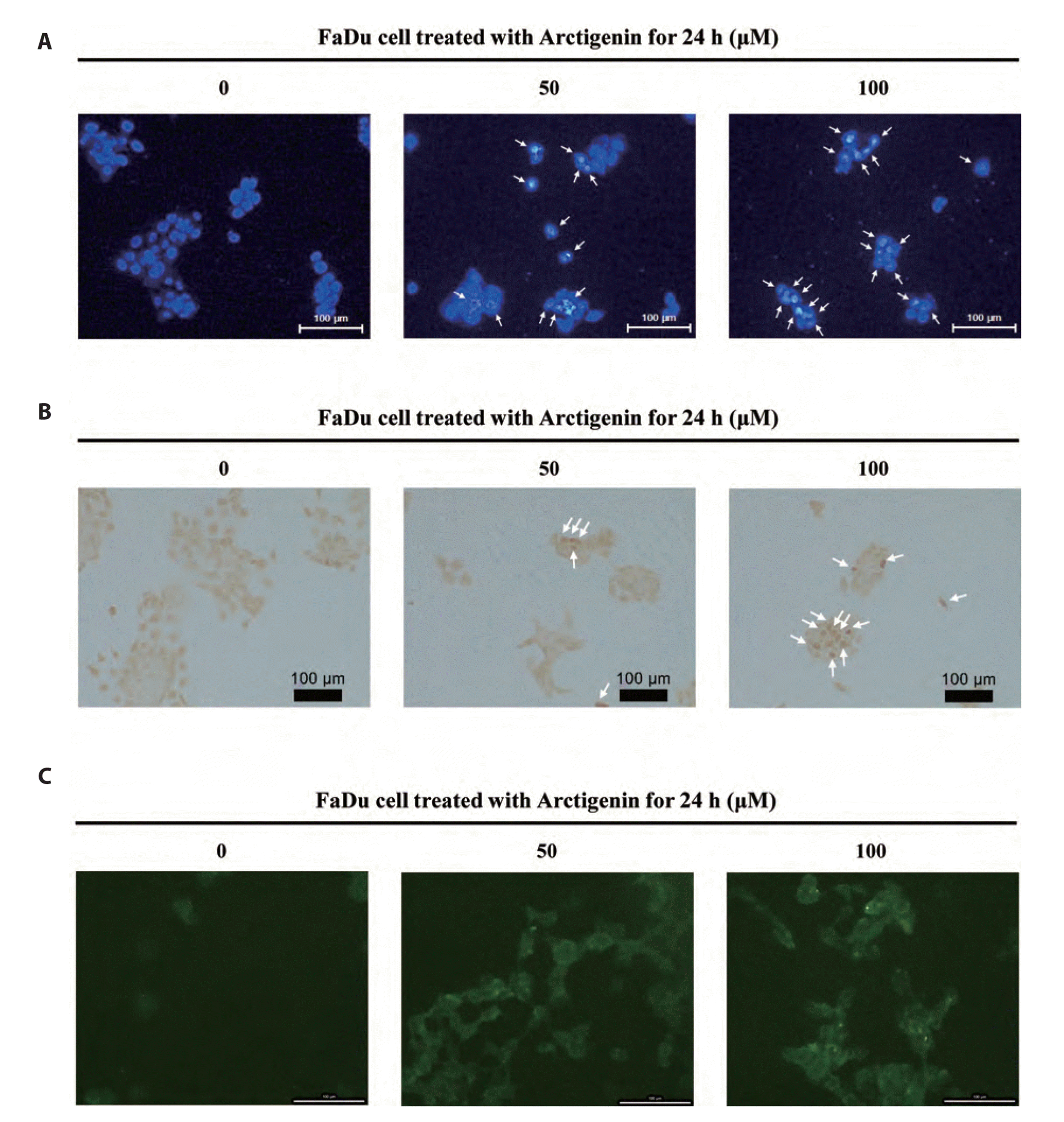
Fig. 5
Arctigenin-induced FaDu pharyngeal carcinoma cell death is meditated by both intrinsic and extrinsic pathways.
(A) Extrinsic death receptor-mediated apoptotic signaling pathway induced by Arctigenin. Arctigenin upregulated the expression level of the death receptor ligand Fas and subsequently activated the extrinsic death receptor-mediated apoptotic signaling pathway through the cleavage of caspase-8 in FaDu cells. (B) Intrinsic mitochondria-dependent apoptotic signaling pathway induced by Arctigenin. Arctigenin downregulated anti-apoptotic factors Bcl-2 and Bcl-xL associated with the intrinsic mitochondria-dependent apoptotic pathway and upregulated the mitochondria-dependent pro-apoptotic factors Bax and Bad in FaDu cells. (C) Extrinsic death receptor-mediated and intrinsic mitochondria-dependent apoptosis signaling pathways via the activation of caspase-3 and PARP induced by Arctigenin. Cleaved caspase-8 and cleaved caspase-9 induced the activation of caspase-3 and PARP in FaDu cells treated with Arctigenin.

Fig. 6
Arctigenin-induced apoptosis in FaDu cells was mediated by caspase.
(A) Z-VAD-fmk, a pan-caspase inhibitor, reversed Arctigenin induced cell death in FaDu cells. (B) Activation of caspase-3 and PARP in FaDu cells treated with Arctigenin was inhibited in the presence of Z-VAD-fmk. Each data point represents the mean ± SEM of four experiments (**p < 0.01).
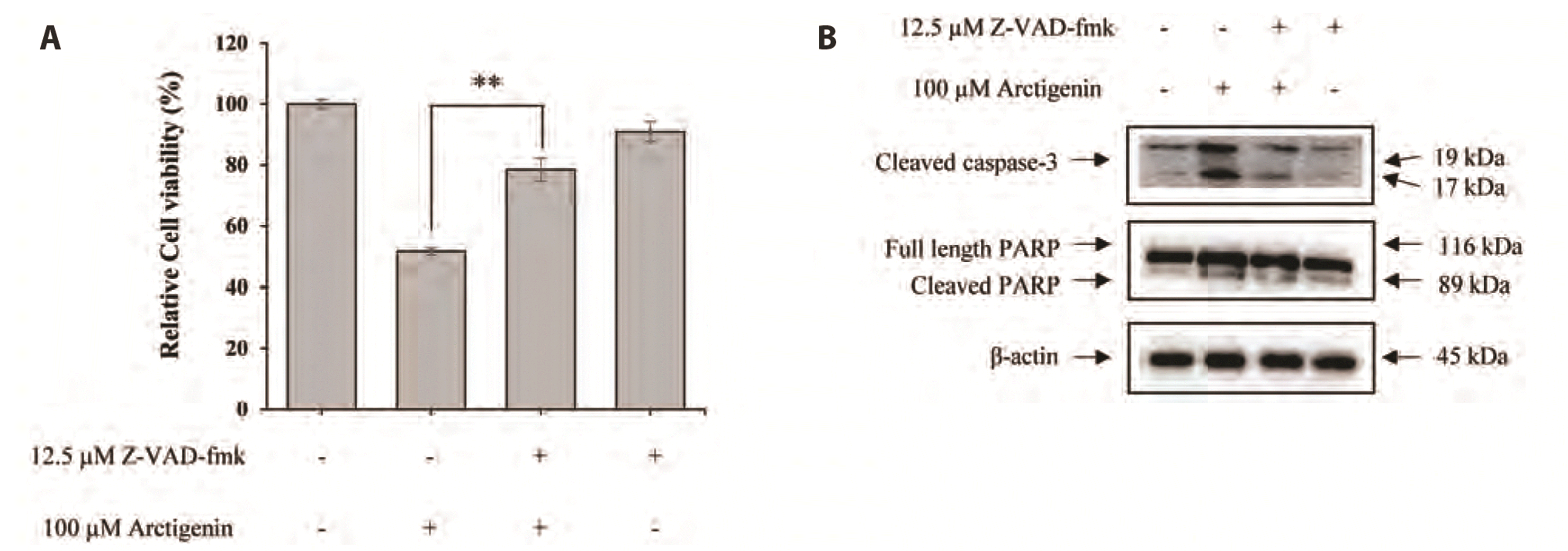
Fig. 7
Arctigenin reduced the phosphorylation of proteins in the MAPKs, NF-κB, and AKT pathways in FaDu pharyngeal cells.
FaDu cells were treated with 0, 50, and 100 μM Arctigenin for 24 h. Thereafter, immunoblotting using specific antibodies against extracellular signal-regulated kinase 1/2 (ERK1/2), p38, NFκB, and AKT was performed to verify potential cellular signaling pathways associated with Arctigenin-induced apoptosis.
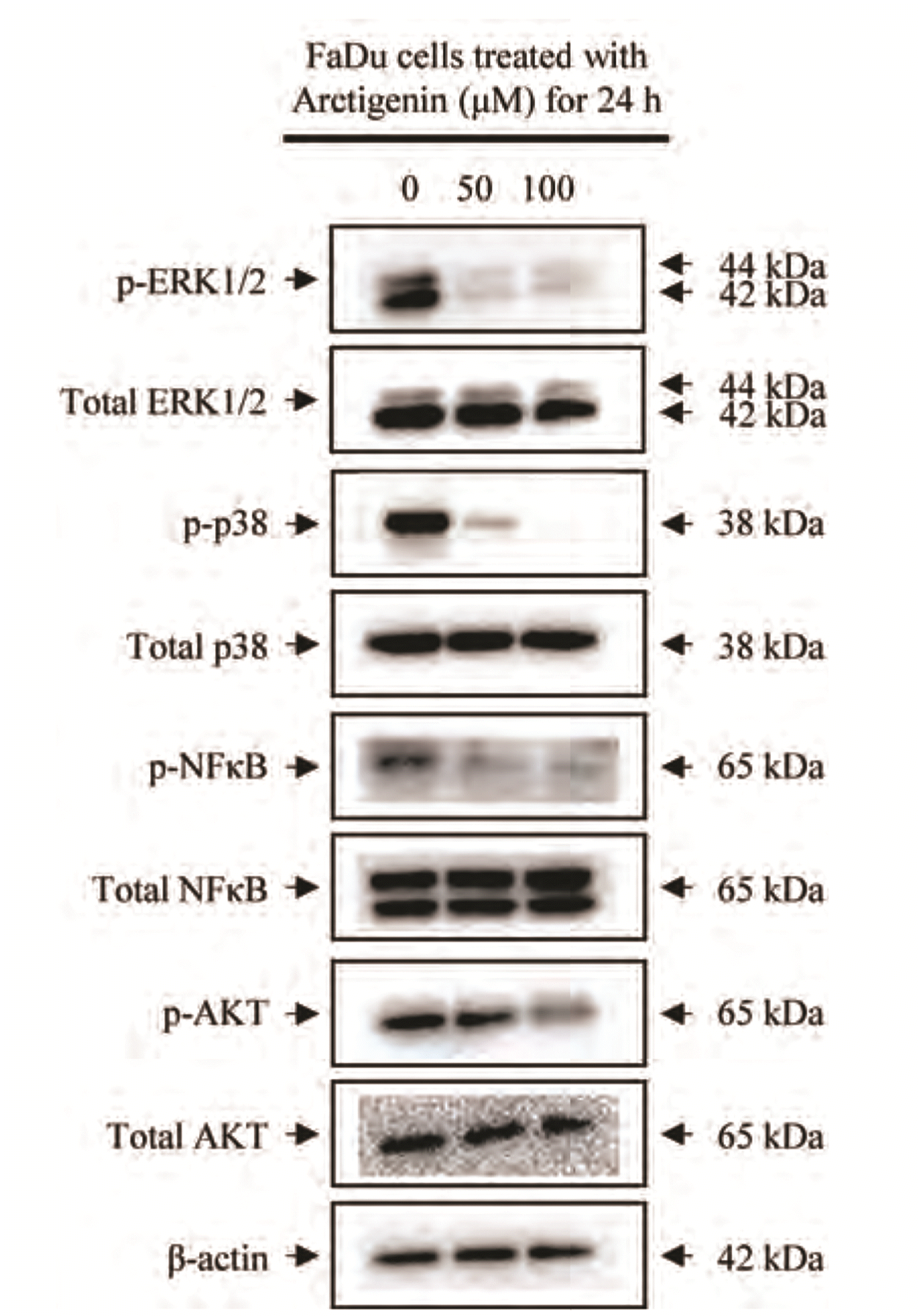