Abstract
Hair loss is a common status found among people of all ages. Since the role of hair is much more related to culture and individual identity, hair loss can have a great influence on well-being and quality of life. It is a disorder that is observed in only scalp patients with androgenetic alopecia (AGA) or alopecia areata caused by stress or immune response abnormalities. Food and Drug Administration (FDA)-approved therapeutic medicines such as finasteride, and minoxidil improve hair loss temporarily, but when they stop, they have a limitation in that hair loss occurs again. As an alternative strategy for improving hair growth, many studies reported that there is a relationship between the expression levels of prostaglandins (PGs) and hair growth. Four major PGs such as prostaglandin D2 (PGD2), prostaglandin I2 (PGI2), prostaglandin E2 (PGE2), and prostaglandin F2 alpha (PGF2α) are spatiotemporally expressed in hair follicles and are implicated in hair loss. This review investigated the physiological roles and pharmacological interventions of the PGs in the pathogenesis of hair loss and provided these novel insights for clinical therapeutics for patients suffering from alopecia.
The formation of hair follicles and hair growth is tightly regulated by epithelial-mesenchymal interactions. The epithelium is comprised of the inner root sheath (IRS), the outer root sheath (ORS), the matrix, and the hair shaft, whereas mesenchyme contains dermal papilla and dermal sheath cells [1,2]. The hair follicle undergoes three kinds of cycles including the growth phase (called anagen), the regression phase (called catagen), and the resting phase (called telogen).
Androgenetic alopecia (AGA) is one of the hair cycle disorders affecting both males and females and causes inconvenience in modern social life. Hair loss is generally accompanied by thinned skin areas in the bald region [3,4]. This alopecia is generally caused by various internal factors such as a genetic problem or hormonal balance [3-5]. The major pathologic alteration of hair follicles in AGA exhibits a gradual shortening of the anagen phase in hair cycle dynamics. 5α-reductase plays an important role in the conversion of testosterone to dihydrotestosterone (DHT) in dermal papilla cells. After DHT binds to androgen receptors, the following signaling pathways disturb hair growth and eventually miniaturize hair follicles [3,4,6]. However, the precise molecular mechanism underlying AGA has not yet been fully elucidated. There have been many therapeutic trials for hair loss such as medicine and hair transplantation [7,8]. There are two Food and Drug Administration (FDA)-approved therapeutic medicines Finasteride and minoxidil [7,9]. Finasteride is well known for suppressing the 5-alpha-reductase, thus preventing the conversion of testosterone to DHT. Minoxidil is a 2,4-di-amino-6-piperidinopyrimidine-3-oxide and is effective in AGA by prolonging anagen, shortening telogen, and leading to enlarging miniaturized hair follicles. These two medicines have the following limitation. They provide a temporary improvement, and if one stops taking medication, it causes immediate hair loss.
As another strategy for treating hair loss, it has been proposed that prostaglandins (PGs) may play a key role in the etiology of hair loss [10,11]. PGs are unsaturated carboxylic acids, which are 20 carbo skeletons and are synthesized from arachidonic acid. They are signaling molecules observed in most tissues, and have various functions including the activation of the inflammatory response, pain production, and fever [12]. The cyclooxygenase (COX) enzyme metabolizes the arachidonic acid into prostaglandin H2 (PGH2), which is a kind of origin prostaglandin [13,14]. The molecular structure of PGH2 can be modified by specific synthase to produce several isoforms of prostaglandin such as prostaglandin D2 (PGD2), prostaglandin E2 (PGE2), and prostaglandin F2 alpha (PGF2α). Colombe et al. [13] proposed that the hair cell types had prostaglandin metabolism machinery and could produce PGE2 and PGF2α. The receptors of all four major PGs (PGE2, PGD2, PGF2α, and prostaglandin I2 [PGI2]) were also observed in hair follicles. Interestingly, PGD2 and its PGD2 enzyme synthase were significantly up-regulated in skin biopsies derived from male bald scalps [15,16]. PGE2 and PGF2α could contribute to accelerating hair growth in mice and protect them from alopecia [15-17].
This review investigated the physiological roles and pharmacological interventions of the PGs in the pathogenesis of hair loss and provided these novel insights into hair loss for meaningful interventions for patients.
Hair follicles are composed of the epidermal compartment and the dermal compartments, especially showing a bulb-shaped structure [1,2,15,17]. These dermal papillae consist of dermal papillar cells (DPCs), which play a key role in hair follicle morphogenesis, hair cycle, and hair growth. These dermal papillae are surrounded by keratinocytes in the hair matrix region. These keratinocytes proliferate and form hair fiber during hair growth. Hair development undergoes three stages including induction, organogenesis, and cytodifferentiation [1,2,15]. The induction stage forms a placode by the thickening of epithelial cells. During the organogenesis stage, the epithelial cells transmit signals to dermal cells, and then, contribute to forming a dermal condensate, which induces the migration of epithelial cells toward the dermis. During the cytodifferentiation stage, the dermal condensate finally forms the dermal papillae [1,2,15].
Hair growth undergoes a hair cycle, which is composed of the anagen phase for growth, the catagen phase for cessation, and the telogen phase for rest. The anagen phase lasts from two to eight years, while the catagen phase takes from two to three weeks. The telogen phase ends with a fall from the original hair and is followed by the generation of new hair. If the onset of anagen is significantly delayed, this leads to harmful effects on hair regeneration [15-17].
Minoxidil, a known FDA-approved medicine for hair growth, stimulated the activity of COX-1, implying that PGs have a positive role in hair growth. Several transgenic mouse models overexpressing COX-2 have been developed, and these transgenic mice have shown characteristic phenotypes of hair dysregulation [18-20]. Neufang et al. [18] demonstrated that the COX-2 transgenic mice model under the control of a keratin 5 promoter led to retarded hair follicle morphogenesis, diminished density of hair follicles, and the hyperplasia of a sebaceous gland [18]. Bol et al. [19] also reported that COX-2 overexpressing transgenic mice using a human keratin 14 promoter exhibited distinct alopecia. These K14-COX-2 transgenic mice showed atrophy of the skin and enlargement of sebaceous glands. Furthermore, treatment of celecoxib, a selective COX-2 inhibitor, recovered hair growth, implying that the alopecia was due to increased COX-2 activity [19]. Similarly, another study observed that homozygous COX-2 overexpressing transgenic mice accelerated the catagen stage and abnormal status in the hair follicle cycle. As hair follicles at early-stage gradually turned into fully differentiated follicles, COX-2 expression exclusively occurred in ORS and sebaceous gland cells. When follicles entered the catagen phase, the expression level of COX-2 was decreased and was barely detectable in telogen follicles. The expression levels of both COX-1 and COX-2 are spatiotemporally changed during the morphogenesis and cycling of hair follicles, which affects the production of PGs. Interestingly, these mice also exhibited alopecia and sebaceous gland hyperplasia and were recovered by the specific COX-2 inhibitor [20]. COX-1 was also present in the dermal papilla cells, whereas COX-2 was exclusively expressed in the anagen status of the dermal papilla. Although COX-2 activity was necessary for the early growth of hair follicles, treatment of PGD2 turned the anagen phase into the catagen phase and led to inducing alopecia [21].
Prostanoids are one of the representative lipid mediator groups, which contains PGs and thromboxanes (TXA2). Prostanoids are generated by the serial reaction of phospholipase A2 and prostaglandin endoperoxide synthases. And then, the endoperoxide prostaglandin is processed into prostaglandins or thromboxanes by specific isomerases. Prostanoids play a role as autocrine/paracrine hormones by using specific G-protein coupled receptors (GPCRs) as downstream signaling molecules [21]. They have various biological effects on the regulation of cell growth, apoptosis, and inflammatory responses. Prostanoids are classified into five types, namely PGD2, PGE2, PGF2α, PGI2, and TXA2. PGD2 binds to prostaglandin D2 receptors (DP1, DP2), whereas PGE2 binds to prostaglandin E2 receptors (EP1, EP2, EP3, EP4) (Fig. 1). PGF2α binds to the prostaglandin F2α receptor (FP). PGI2 binds to the prostaglandin I2 receptor (IP). TXA2 binds to the prostanoid thromboxane A2 receptor (TP) (Fig. 1) [22]. Colombe et al. [13] examined the expression profile of the main enzymes involved with PGs metabolism in human hair follicles. They demonstrated that human hair follicles remarkably expressed microsomal PGES (mPGES)-1, mPGES-2, and cytosolic PGES (cPGES), which converts PGH2 to PGE2 (Fig. 1). These human hair follicles also significantly expressed AKR1C3/PGFS, which catalyzes PGH2 to PGF2α (Fig. 1) [13,15]. PGE2 and PGF2α metabolism exist in human hair follicles. PGD2, PGI2, and TXA2 synthases were also expressed in the human hair follicle. These results suggested that PGs may be associated with hair growth and differentiation.
Some studies reported the distribution of prostanoid receptors in human hair follicles. All prostanoid receptors are one of the rhodopsin family, which has seven transmembrane domains, and are present in hair follicles [15]. The EP2, EP3, EP4, DP2, TP, and EP1 are associated with the hair follicle compartments, whereas DP1 and IP are expressed in ORS basal layer and the hair cuticle layer, respectively (Fig. 1). FP was expressed in the dermal papilla and the ORS companion layer [15]. In addition, Nesher et al. [23] found that FP was also present in the IRS region of the bulb and stem region of eyelashes and exclusively expressed in eyelashes at the anagen phase, implying that the expression level of FP in dermal papilla is involved with the effect of PGF2α on hair growth. These prostanoid receptors are classified into three types. TP, EP1, and FP initiate Gq protein-coupled Ca2+ signaling, the generation of inositol 1,4,5-triphosphate, and the activation of protein kinase C. IP, EP2, EP4, and DP1 induce the activation of Gαs protein-coupled adenylate cyclase and lead to increasing cAMP synthesis and the activation of protein kinase A. EP3 and DP2 initiate the inhibition of Gi protein-coupled adenylate cyclase and resulting in the down-regulation of intracellular cAMP [24]. Various cAMP or Ca2+ amplitude induces specific stimulation of cell regulation mechanisms.
Prostaglandin D2 synthase (PTGDS), which synthesizes prostaglandin D2 (PGD2), exhibits hormone response in various organs. PGD2 binds to two known G protein-coupled receptors, prostaglandin D2 receptor type I (DP1 or PTGDR) and prostaglandin D2 receptor type II (DP2 or GPR44) (Fig. 1) [25,26]. Since androgens affect stimulating PTGDS, the stimulation of PGD2 could come from enhanced androgen levels [27].
Garza et al. [16] first demonstrated that the expression levels of PTGDS and its product, PGD2, were significantly increased in the region of the balding scalp compared to that in the region of the non-balding scalp in the patient group with AGA. Interestingly, they revealed that expression levels of PGE2 and PGF2α were remarkably reduced in bald areas compared to the haired areas. In addition, PG levels in healthy samples were similar to the haired areas. They also revealed that PGD2 and its metabolite, 15-deoxy-Δ12,14-prostaglandin J2 (15-dPGJ2), suppressed the growth of explanted human hair follicles and suppress hair growth via DP2, but not the PTGDR in mice [16]. They further demonstrated that K14-Ptgs2, a transgenic mouse that targets PGES-2, enhanced expression levels of PGD2 in the skin and developed alopecia by showing several symptoms such as sebaceous gland hyperplasia and hair follicle miniaturization. These results implied that the precise balance among these four components in scalp hair follicles, and their maintenance may be important for hair growth. Thus, the PGD2-DP2 pathway can be a potential target for treating androgenetic alopecia [11,16].
Prostaglandins are one of the key inflammatory factors related to wound closure and the modulation of hair growth [28,29]. In a subsequent study, Nelson et al. [29] reported that PGE2 and PGD2 were enhanced at the early and late stages during wound healing in wound-induced hair follicle neogenesis (WIHN). They further demonstrated that the highest expression levels of PGD2 were involved with the lowest amount of hair regeneration. Interestingly, exogenous application of PGD2 remarkably down-regulated WIHN in wild-type mice, and DP2-knockout mice exhibited the improvement of WIHN compared to control mice. In addition, DP2-knockout mice were resistant to PGD2-induced suppression of hair follicle neogenesis. These results suggested that PGD2 affects hair follicle regeneration via DP2 [29].
Another study demonstrated that DP2 is remarkably expressed in the ORS cells and slightly expressed in the dermal papilla cells [30]. Interestingly, they found that a higher concentration of 15-dPGJ2 treatment (5 μM) significantly suppressed the cell viability and caused apoptosis of ORS, dermal papilla cells, and follicular keratinocytes of cultured human hair follicles, implying that 15-dPGJ2 provides a theoretical basis for the development of therapeutic agents for improving hair loss, which is based on DP2 antagonism [16,30].
Mantel et al. [31] group examined the relationship between PGD2 and testosterone in the skin. They demonstrated that the treatment of PGD2 on human keratinocytes increased the capacity to convert from androstenedione to testosterone, and induced the level of reactive oxygen species (ROS) by the production of the lipid peroxidation product, 4-hydroxynonenal. Furthermore, they demonstrated that N-acetyl-cysteine, one of the ROS scavengers, disturbed the increased production of testosterone from PGD2-treated keratinocytes, implying that the PGD2-ROS axis can be possible crosstalk with testosterone metabolism in keratinocytes [31]. These results suggested that antioxidants can be clinically utilized as a supplement for AGA therapeutics including PGD2 receptor blockers, finasteride, and minoxidil.
AGA exhibited a loss of CD200+/CD34+ cells while retaining a keratin 15+ (K15+) cell population [32]. Human scalp hair follicles (hHFs) contain several epithelial stem cells (eHFSCs) and progenitor cells, which are spatially organized into distinct niches. Purba et al. [33] group observed the relative inactivity of the cell cycle in the CD200+/K15+ bulge region compared to other non-bulge CD34+ and K15+ progenitor regions. Interestingly, they found that PGD2 differentially affected the proliferation of distinct eHFSCs populations. Administration of PGD2 on organ-cultured hHFs for 24 h decreased the expression level of Ki-67 and the incorporation of 5-ethynyl-2'-deoxyuridine (EdU) in bulge resident K15+ cells, but not ORS K15+ progenitors in supra/proximal bulb, implying that there are differences between the cell cycle behavior of distinct stem/progenitor cell niches in the hHFs spatially. These results implied that PGD2 could perturb the dynamics of proliferation in epithelial stem cells [33]. Another previous study revealed that PGD2 stimulated the expression level of androgenic receptors and AKT signaling via DP2. PGD2 also induced androgenic receptors-related proteins including transforming growth factor-beta 1 (TGF-β1), insulin-like growth factor 1 (IGF-1), lymphoid enhancer binding factor 1 (LEF1), GSK3β, and Creb on the expression levels of androgenic receptors in human dermal papilla cells. As expected, DP2 antagonists (TM30089) and AKT inhibitors (LY294002) down-regulated these factors, reduced the expression levels of androgenic receptors, and suppressed AKT signaling in human dermal papilla cells [34]. These data implied that PGD2 accelerated androgenic receptor, and AKT signaling through DP2 in human dermal papilla cells and provide insights into the development of therapeutics for treating AGA by inhibiting PGD2-DP2. These results provide novel strategies which suppress the activity of PTGDS, PGD2, or DP2 for treating androgenetic alopecia. Interestingly, a recent study demonstrated that the expression levels of PGD2 and PGI2 are relatively low in the haired scalp but are remarkably enhanced in the bald scalp [35].
Cetirizine is a well-known antihistamine for its anti-inflammatory properties and its ability to decrease PGD2 production. A previous study demonstrated that cetirizine suppressed the release of PGD2 [36]. A recent study demonstrated that a 1% cetirizine-treated group for 6 months exhibited remarkably higher hair regrowth compared to the control group (Table 1) [37]. Another recent study observed that a 1% cetirizine solution for 16 weeks was effective in hair growth and hair density by increasing the percentage of hair in the anagen phase for the treatment of male AGA, but its effects were less than the minoxidil group (Table 1) [38]. These results imply that cetirizine can be used as a potential therapeutic in treating AGA.
Setipiprant is a selective oral inhibitor against DP2 [39]. A phase 2A clinical trial of a setipiprant for treating AGA was completed (NCT02781311). The oral treatment of 500 mg setipiprant twice daily exhibited safety and tolerability but did not show statistically significant efficacy compared to the placebo (Table 1) [39].
Levocetirizine hydrochloride down-regulated the expressions levels of PGD2, PTGDS, and DP2 compared to the control group, whereas up-regulated the expressions levels of PGF2α, pAKT, and pGSK3β. These results suggested that levocetirizine hydrochloride accelerated the growth and proliferation of hDPCs by suppressing the PGD2-DP2 pathway and stimulating the AKT signaling pathway (Table 1) [40].
Until now, two candidate drugs have been studied to improve hair growth by increasing PGF2α. Latanoprost is a PGF2α analog, which is clinically used to cure glaucoma. Treatment of unilateral topical latanoprost induced hypertrichosis including eyelashes, regional intermediate hairs of the eyelid, and ipsilateral terminal eyelashes by increasing the number, length, and thickness of hair in the treated region [41]. The administration of 0.1% latanoprost on patients with AGA exhibited an increase in hair density compared to the control. 45% of patients with AGA were satisfied with a combinatory administration of both 0.005% latanoprost ophthalmic solution and triamcinolone acetonide (Table 1) [42]. On the contrary, two other reports demonstrated that the administration of topical latanoprost failed to improve eyelashes and eyebrows [43,44]. The discrepancy regarding the effects of the latanoprost probably was due to different experimental methods such as the experimental period, gender, and age. Another study demonstrated that the treatment of 0.1% latanoprost in males with mild AGA for 24 weeks remarkably increased hair density, hair growth, hair diameter, and pigmentation (Table 1) [45]. These results suggest that latanoprost may be a potential drug for stimulating the activity of hair follicles and treating AGA.
Recently, latanoprost was also found to be effective in treating alopecia areata, which is a recurrent chronic disease affecting hair follicles and causing hair loss in the specific site. Administration of topical 0.005% latanoprost for 12 weeks significantly enhanced hair density and regrowth compared to the control group. The results also exhibited the acceptable safety and efficacy of 0.005% latanoprost solution, implying that latanoprost may be safely used for treating alopecia areta (Table 1) [46].
Bimatoprost is a PGF2α analog approved by FDA for treating eyelash hypotrichosis [47-50]. The safety and efficacy of bimatoprost for the treatment of eyelash hypotrichosis have been well elucidated [50,51]. Yoelin et al. [50] demonstrated that the application of 0.03% ophthalmic bimatoprost solution induced a higher growth of eyelashes compared with latanoprost (Table 1). Furthermore, 0.03% bimatoprost treatment for eyelash hypotrichosis in 585 subjects revealed a 92.5% satisfaction level, and the safe of long-term use for at least 12 months. Suwanchatchai et al. [52] reported that bimatoprost was similarly effective to minoxidil in improving eyebrow thickness, and had relatively fewer side effects (Table 1). Another study demonstrated that daily treatment of 0.03% bimatoprost ophthalmic solution for 1 year was significantly effective and well tolerated in patients suffering from eyelash hypotrichosis (Table 1) [53]. On the other hand, the efficacy of bimatoprost for the treatment of alopecia areata in patients has been debatable [54-58]. A previous study reported that 0.03% bimatoprost exhibited significant efficacy for treating eyelash universalis alopecia areata [54]. Roseborough et al. [57] demonstrated that bimatoprost showed no significant changes in growing eyelashes in patients suffering from alopecia areata. Borchert et al. [58] found that 0.03% bimatoprost exhibited significant efficiency of eyelash hypotrichosis in healthy adolescents, but not in alopecia areata groups (Table 1). Thus, it is necessary to study larger sample size, longer study period, and higher drug concentrations to confirm whether bimatoprost is effective in curing alopecia areata.
Recently, Subedi et al. [59] prepared a topical formulation of bimatoprost having high skin permeability (Table 1). They designed a solvent mixture system, which contained ethanol, cyclomethicone, diethylene glycol monoethyl ether, and butylated hydroxyanisole. This formulation of 5 μM bimatoprost stimulated the proliferation of human dermal papilla cells, equivalent to 10 μM minoxidil. Furthermore, 0.3% bimatoprost remarkably accelerated hair regrowth in the AGA mouse model and exhibited a 585% increase within 10 days compared to the control group, implying that most of the telogen was transitioned into the anagen phase. This 5% bimatoprost formulation significantly increased the hair weight by 8.45- and 1.30-fold compared to the 5% bimatoprost in ethanol and 5% minoxidil-treated groups, respectively. This 5% bimatoprost formulation also remarkably enhanced the number and diameter of hair follicles compared to the vehicle-treated mice or 5% (w/w) bimatoprost in the ethanol-treated group [59]. The clinical application of bimatoprost to the treatment of AGA is also needed. Currently, several clinical trials with bimatoprost for improving AGA are processed in the US National Institutes of Health Clinical Trials database (https://clinicaltrials.gov).
Interestingly, after tissue injury, unexpected hair growth can occur, which will be likely related to inflammatory mediators. Recently, high-mobility group box 1 (HMGB1), one of the nuclear proteins that are released from nuclei after tissue injury, was identified as one of these inflammatory mediators [60]. Hwang et al. [60] demonstrated that HMGB1 increased hair shaft elongation in a hair organ culture. Interestingly, HMGB1 remarkably enhanced expression levels of PGES and led to the increased secretion of PGE2 from human dermal papilla cells, implying that HGMB1 can be a potential therapeutic target for curing alopecia.
Based on many previous studies, this review provides that there is a highly remarkable correlation between the expression levels of PGs and hair growth. Four major PGs including PGD2, PGI2, PGE2, and PGF2α are implicated in the pathogenesis of the hair loss pattern of both males and females [10,11,16,35,61]. That is, PGD2 and PGI2 contribute to inhibiting hair growth, On the other hand, PGE2 and PGF2α contribute to promoting hair growth. Thus, the lack of PGE2 and PGF2α, while the increase of PGD2 and PGI2, can lead to hair follicle miniaturization.
These results imply that there is a precise balance between these four PGs in hair follicles, and their maintenance can be important for hair growth. Although both PGE2 and PGD2 are present in the hair follicle, they differently affect the hair growth [15]. Female scalps seem to have hair follicles that generate more PGE2 and PGF2α than males scalps [13]. This may be another reason why most females did not suffer from massive hair loss during the aging process. PG receptors could be also potential molecular targets for the treatment of AGA. Several studies reported that the expression levels of PGD2 and PGI2 receptors are severely low in the haired scalp, whereas the expression levels of PGE2 and PGF2α receptor levels are significantly high compared to bald areas [15,17]. Interestingly, a recent study also demonstrated that there is no significant difference between the expression levels of PGs in comparing either occipital hairy areas or frontal bald in males and females [35]. This result implied that PG may represent a final common pathway, even if AGA in females may not be androgen-mediated. Thus, targeting PGs must help improve both males and females suffering from hair loss. When reaching specific circumstances, the arachidonic acid cascade can induce a high level of PGD2 whereas lowering the amount of PGE2 in the alopecia area. Thus, future research is needed to understand gender differences in the relationship between PGs and PG receptors in hair follicles.
A previous study found that the expression levels of the D2, PTGDS, and PTGES exhibited no differences in balding scalps between Hispanic male and female patients with AGA. Both PTGDS and PTGES were significantly increased in males with AGA compared to controls, whereas these genes are not different in females. There were no statistical differences in the expression level of D2 in males or females comparing balding and non-balding areas. Interestingly, Hispanic males with early male pattern hair loss strongly overexpressed the PTGES, which synthesizes PGE2 in suffering from AGA, implying that PGs may play a different role, depending on the progress stage of AGA [61]. Thus, future studies are required to elucidate the precise functional roles and their underlying signaling mechanism of PGs in hair follicles in detail. It can be also considered that a proper combinatory treatment of PGE2 agonist and PGD2 antagonist can be another solution for treating hair loss. Thus, much research is also necessary for clarifying the efficacy, safety, and underlying mechanism of these candidate therapeutics for improving hair growth.
Notes
REFERENCES
1. Botchkarev VA, Kishimoto J. 2003; Molecular control of epithelial-mesenchymal interactions during hair follicle cycling. J Investig Dermatol Symp Proc. 8:46–55. DOI: 10.1046/j.1523-1747.2003.12171.x. PMID: 12894994. PMID: https://www.scopus.com/inward/record.uri?partnerID=HzOxMe3b&scp=0041868117&origin=inward.


2. Stenn KS, Paus R. 2001; Controls of hair follicle cycling. Physiol Rev. 81:449–494. DOI: 10.1152/physrev.2001.81.1.449. PMID: 11152763. PMID: https://www.scopus.com/inward/record.uri?partnerID=HzOxMe3b&scp=0035134022&origin=inward.


3. Kaufman KD. 2002; Androgens and alopecia. Mol Cell Endocrinol. 198:89–95. DOI: 10.1016/S0303-7207(02)00372-6. PMID: 12573818. PMID: https://www.scopus.com/inward/record.uri?partnerID=HzOxMe3b&scp=0037203503&origin=inward.


4. Sawaya ME, Keane RW, Blume-Peytavi U, Mullins DL, Nusbaum BP, Whiting D, Nicholson DW. 2001; Androgen responsive genes as they affect hair growth. Eur J Dermatol. 11:304–308. PMID: 11399535. PMID: https://www.scopus.com/inward/record.uri?partnerID=HzOxMe3b&scp=0034936539&origin=inward.
5. Bak DH, Choi MJ, Kim SR, Lee BC, Kim JM, Jeon ES, Oh W, Lim ES, Park BC, Kim MJ, Na J, Kim BJ. 2018; Human umbilical cord blood mesenchymal stem cells engineered to overexpress growth factors accelerate outcomes in hair growth. Korean J Physiol Pharmacol. 22:555–566. DOI: 10.4196/kjpp.2018.22.5.555. PMID: 30181702. PMCID: PMC6115345. PMID: https://www.scopus.com/inward/record.uri?partnerID=HzOxMe3b&scp=85052861159&origin=inward.


6. Inui S, Itami S. 2013; Androgen actions on the human hair follicle: perspectives. Exp Dermatol. 22:168–171. DOI: 10.1111/exd.12024. PMID: 23016593. PMID: https://www.scopus.com/inward/record.uri?partnerID=HzOxMe3b&scp=84875050350&origin=inward.


7. Lee SW, Juhasz M, Mobasher P, Ekelem C, Mesinkovska NA. 2018; A systematic review of topical finasteride in the treatment of androgenetic alopecia in men and women. J Drugs Dermatol. 17:457–463. PMID: 29601622. PMCID: PMC6609098. PMID: https://www.scopus.com/inward/record.uri?partnerID=HzOxMe3b&scp=85053729398&origin=inward.
8. Jimenez F, Alam M, Vogel JE, Avram M. 2021; Hair transplantation: basic overview. J Am Acad Dermatol. 85:803–814. DOI: 10.1016/j.jaad.2021.03.124. PMID: 33905785. PMID: https://www.scopus.com/inward/record.uri?partnerID=HzOxMe3b&scp=85114433698&origin=inward.


9. Adil A, Godwin M. 2017; The effectiveness of treatments for androgenetic alopecia: a systematic review and meta-analysis. J Am Acad Dermatol. 77:136–141.e5. DOI: 10.1016/j.jaad.2017.02.054. PMID: 28396101. PMID: https://www.scopus.com/inward/record.uri?partnerID=HzOxMe3b&scp=85017108673&origin=inward.


10. Sasaki S, Hozumi Y, Kondo S. 2005; Influence of prostaglandin F2α and its analogues on hair regrowth and follicular melanogenesis in a murine model. Exp Dermatol. 14:323–328. DOI: 10.1111/j.0906-6705.2005.00270.x. PMID: 15854125. PMID: https://www.scopus.com/inward/record.uri?partnerID=HzOxMe3b&scp=18844450968&origin=inward.


11. Nieves A, Garza LA. 2014; Does prostaglandin D2 hold the cure to male pattern baldness? Exp Dermatol. 23:224–227. DOI: 10.1111/exd.12348. PMID: 24521203. PMCID: PMC3982925. PMID: https://www.scopus.com/inward/record.uri?partnerID=HzOxMe3b&scp=84896995060&origin=inward.


12. Smyth EM, Grosser T, Wang M, Yu Y, FitzGerald GA. 2009; Prostanoids in health and disease. J Lipid Res. 50(Suppl):S423–S428. DOI: 10.1194/jlr.R800094-JLR200. PMID: 19095631. PMCID: PMC2674745. PMID: https://www.scopus.com/inward/record.uri?partnerID=HzOxMe3b&scp=66349102248&origin=inward.


13. Colombe L, Vindrios A, Michelet JF, Bernard BA. 2007; Prostaglandin metabolism in human hair follicle. Exp Dermatol. 16:762–769. DOI: 10.1111/j.1600-0625.2007.00586.x. PMID: 17697149. PMID: https://www.scopus.com/inward/record.uri?partnerID=HzOxMe3b&scp=34547912906&origin=inward.


14. Guevara-Balcazar G, Ramirez-Sanchez I, Mera-Jimenez E, Rubio-Gayosso I, Aguilar-Najera ME, Castillo-Hernandez MC. 2017; Participation of COX-1 and COX-2 in the contractile effect of phenylephrine in prepubescent and old rats. Korean J Physiol Pharmacol. 21:407–413. DOI: 10.4196/kjpp.2017.21.4.407. PMID: 28706454. PMCID: PMC5507779. PMID: https://www.scopus.com/inward/record.uri?partnerID=HzOxMe3b&scp=85023747329&origin=inward.


15. Colombe L, Michelet JF, Bernard BA. 2008; Prostanoid receptors in anagen human hair follicles. Exp Dermatol. 17:63–72. DOI: 10.1111/j.1600-0625.2007.00639.x. PMID: 18005048. PMID: https://www.scopus.com/inward/record.uri?partnerID=HzOxMe3b&scp=37249028593&origin=inward.


16. Garza LA, Liu Y, Yang Z, Alagesan B, Lawson JA, Norberg SM, Loy DE, Zhao T, Blatt HB, Stanton DC, Carrasco L, Ahluwalia G, Fischer SM, FitzGerald GA, Cotsarelis G. 2012; Prostaglandin D2 inhibits hair growth and is elevated in bald scalp of men with androgenetic alopecia. Sci Transl Med. 4:126ra34. DOI: 10.1126/scitranslmed.3003122. PMID: 22440736. PMCID: PMC3319975. PMID: https://www.scopus.com/inward/record.uri?partnerID=HzOxMe3b&scp=84863344412&origin=inward.


17. Xu XG, Chen HD. 2018; Prostanoids and hair follicles: implications for therapy of hair disorders. Acta Derm Venereol. 98:318–323. DOI: 10.2340/00015555-2843. PMID: 29136266. PMID: https://www.scopus.com/inward/record.uri?partnerID=HzOxMe3b&scp=85043334752&origin=inward.


18. Neufang G, Furstenberger G, Heidt M, Marks F, Müller-Decker K. 2001; Abnormal differentiation of epidermis in transgenic mice constitutively expressing cyclooxygenase-2 in skin. Proc Natl Acad Sci U S A. 98:7629–7634. DOI: 10.1073/pnas.121574098. PMID: 11381142. PMCID: PMC34719. PMID: https://www.scopus.com/inward/record.uri?partnerID=HzOxMe3b&scp=0035912711&origin=inward.


19. Bol DK, Rowley RB, Ho CP, Pilz B, Dell J, Swerdel M, Kiguchi K, Muga S, Klein R, Fischer SM. 2002; Cyclooxygenase-2 overexpression in the skin of transgenic mice results in suppression of tumor development. Cancer Res. 62:2516–2521. PMID: 11980643. PMID: https://www.scopus.com/inward/record.uri?partnerID=HzOxMe3b&scp=0036569925&origin=inward.
20. Müller-Decker K, Leder C, Neumann M, Neufang G, Bayerl C, Schweizer J, Marks F, Fürstenberger G. 2003; Expression of cyclooxygenase isozymes during morphogenesis and cycling of pelage hair follicles in mouse skin: precocious onset of the first catagen phase and alopecia upon cyclooxygenase-2 overexpression. J Invest Dermatol. 121:661–668. DOI: 10.1046/j.1523-1747.2003.12473.x. PMID: 14632179. PMID: https://www.scopus.com/inward/record.uri?partnerID=HzOxMe3b&scp=0141887557&origin=inward.


21. Kobayashi T, Narumiya S. 2002; Function of prostanoid receptors: studies on knockout mice. Prostaglandins Other Lipid Mediat. 68-69:557–573. DOI: 10.1016/S0090-6980(02)00055-2. PMID: 12432943. PMID: https://www.scopus.com/inward/record.uri?partnerID=HzOxMe3b&scp=0036669383&origin=inward.


22. Kabashima K, Miyachi Y. 2004; Prostanoids in the cutaneous immune response. J Dermatol Sci. 34:177–184. DOI: 10.1016/j.jdermsci.2003.11.008. PMID: 15113587. PMID: https://www.scopus.com/inward/record.uri?partnerID=HzOxMe3b&scp=2142810073&origin=inward.


23. Nesher R, Mimouni M, Elnaddaf H, Nemet A, Kidron D. 2015; Characterization of prostaglandin F2α receptors in human eyelids. Eur J Ophthalmol. 25:81–84. DOI: 10.5301/ejo.5000519. PMID: 25363851. PMID: https://www.scopus.com/inward/record.uri?partnerID=HzOxMe3b&scp=84922694988&origin=inward.


24. Woodward DF, Jones RL, Narumiya S. 2011; International Union of Basic and Clinical Pharmacology. LXXXIII: classification of prostanoid receptors, updating 15 years of progress. Pharmacol Rev. 63:471–538. DOI: 10.1124/pr.110.003517. PMID: 21752876. PMID: https://www.scopus.com/inward/record.uri?partnerID=HzOxMe3b&scp=79955575162&origin=inward.


25. Gallant MA, Samadfam R, Hackett JA, Antoniou J, Parent JL, de Brum-Fernandes AJ. 2005; Production of prostaglandin D2 by human osteoblasts and modulation of osteoprotegerin, RANKL, and cellular migration by DP and CRTH2 receptors. J Bone Miner Res. 20:672–681. DOI: 10.1359/JBMR.041211. PMID: 15765187. PMID: https://www.scopus.com/inward/record.uri?partnerID=HzOxMe3b&scp=15344348453&origin=inward.


26. Hirai H, Tanaka K, Yoshie O, Ogawa K, Kenmotsu K, Takamori Y, Ichimasa M, Sugamura K, Nakamura M, Takano S, Nagata K. 2001; Prostaglandin D2 selectively induces chemotaxis in T helper type 2 cells, eosinophils, and basophils via seven-transmembrane receptor CRTH2. J Exp Med. 193:255–261. DOI: 10.1084/jem.193.2.255. PMID: 11208866. PMCID: PMC2193345. PMID: https://www.scopus.com/inward/record.uri?partnerID=HzOxMe3b&scp=0035862329&origin=inward.
27. Bhargava S. 2014; Increased DHT levels in androgenic alopecia have been selected for to protect men from prostate cancer. Med Hypotheses. 82:428–432. DOI: 10.1016/j.mehy.2014.01.016. PMID: 24548754. PMID: https://www.scopus.com/inward/record.uri?partnerID=HzOxMe3b&scp=84896733327&origin=inward.


28. Lee JL, Mukhtar H, Bickers DR, Kopelovich L, Athar M. 2003; Cyclooxygenases in the skin: pharmacological and toxicological implications. Toxicol Appl Pharmacol. 192:294–306. DOI: 10.1016/S0041-008X(03)00301-6. PMID: 14575647. PMID: https://www.scopus.com/inward/record.uri?partnerID=HzOxMe3b&scp=0142219780&origin=inward.


29. Nelson AM, Loy DE, Lawson JA, Katseff AS, Fitzgerald GA, Garza LA. 2013; Prostaglandin D2 inhibits wound-induced hair follicle neogenesis through the receptor, Gpr44. J Invest Dermatol. 133:881–889. DOI: 10.1038/jid.2012.398. PMID: 23190891. PMCID: PMC3593761. PMID: https://www.scopus.com/inward/record.uri?partnerID=HzOxMe3b&scp=84875211689&origin=inward.


30. Joo HW, Kang YR, Kwack MH, Sung YK. 2016; 15-deoxy prostaglandin J2, the nonenzymatic metabolite of prostaglandin D2, induces apoptosis in keratinocytes of human hair follicles: a possible explanation for prostaglandin D2-mediated inhibition of hair growth. Naunyn Schmiedebergs Arch Pharmacol. 389:809–813. DOI: 10.1007/s00210-016-1257-z. PMID: 27185495. PMID: https://www.scopus.com/inward/record.uri?partnerID=HzOxMe3b&scp=84968583555&origin=inward.


31. Mantel A, McDonald JT, Goldsborough K, Harvey VM, Chan J. 2017; Prostaglandin D2 uses components of ROS signaling to enhance testosterone production in keratinocytes. J Investig Dermatol Symp Proc. 18:S81–S84. DOI: 10.1016/j.jisp.2017.01.003. PMID: 28941500. PMID: https://www.scopus.com/inward/record.uri?partnerID=HzOxMe3b&scp=85031732444&origin=inward.
32. Garza LA, Yang CC, Zhao T, Blatt HB, Lee M, He H, Stanton DC, Carrasco L, Spiegel JH, Tobias JW, Cotsarelis G. 2011; Bald scalp in men with androgenetic alopecia retains hair follicle stem cells but lacks CD200-rich and CD34-positive hair follicle progenitor cells. J Clin Invest. 121:613–622. DOI: 10.1172/JCI44478. PMID: 21206086. PMCID: PMC3026732. PMID: https://www.scopus.com/inward/record.uri?partnerID=HzOxMe3b&scp=79551525437&origin=inward.


33. Purba TS, Peake M, Farjo B, Farjo N, Bhogal RK, Jenkins G, Paus R. 2017; Divergent proliferation patterns of distinct human hair follicle epithelial progenitor niches in situ and their differential responsiveness to prostaglandin D2. Sci Rep. 7:15197. DOI: 10.1038/s41598-017-15038-9. PMID: 29123134. PMCID: PMC5680340. PMID: https://www.scopus.com/inward/record.uri?partnerID=HzOxMe3b&scp=85033705034&origin=inward.


34. Jeong KH, Jung JH, Kim JE, Kang H. 2018; Prostaglandin D2-mediated DP2 and AKT signal regulate the activation of androgen receptors in human dermal papilla cells. Int J Mol Sci. 19:556. DOI: 10.3390/ijms19020556. PMID: 29439547. PMCID: PMC5855778. PMID: https://www.scopus.com/inward/record.uri?partnerID=HzOxMe3b&scp=85042232574&origin=inward.


35. Chovarda E, Sotiriou E, Lazaridou E, Vakirlis E, Ioannides D. 2021; The role of prostaglandins in androgenetic alopecia. Int J Dermatol. 60:730–735. DOI: 10.1111/ijd.15378. PMID: 33528035. PMID: https://www.scopus.com/inward/record.uri?partnerID=HzOxMe3b&scp=85100287770&origin=inward.


36. Rossi A, Campo D, Fortuna MC, Garelli V, Pranteda G, De Vita G, Sorriso-Valvo L, Di Nunno D, Carlesimo M. 2018; A preliminary study on topical cetirizine in the therapeutic management of androgenetic alopecia. J Dermatolog Treat. 29:149–151. DOI: 10.1080/09546634.2017.1341610. PMID: 28604133. PMID: https://www.scopus.com/inward/record.uri?partnerID=HzOxMe3b&scp=85021690991&origin=inward.


37. Zaky MS, Abo Khodeir H, Ahmed HA, Elsaie ML. 2021; Therapeutic implications of topical cetirizine 1% in treatment of male androgenetic alopecia: a case-controlled study. J Cosmet Dermatol. 20:1154–1159. DOI: 10.1111/jocd.13940. PMID: 33417284. PMID: https://www.scopus.com/inward/record.uri?partnerID=HzOxMe3b&scp=85099361938&origin=inward.


38. Hossein Mostafa D, Samadi A, Niknam S, Nasrollahi SA, Guishard A, Firooz A. 2021; Efficacy of cetirizine 1% versus minoxidil 5% topical solution in the treatment of male alopecia: a randomized, single-blind controlled study. J Pharm Pharm Sci. 24:191–199. DOI: 10.18433/jpps31456. PMID: 33909554. PMID: 17c0c79757a443aabf18ae1209269e9c. PMID: https://www.scopus.com/inward/record.uri?partnerID=HzOxMe3b&scp=85105066571&origin=inward.
39. DuBois J, Bruce S, Stewart D, Kempers S, Harutunian C, Boodhoo T, Weitzenfeld A, Chang-Lin JE. 2021; Setipiprant for androgenetic alopecia in males: results from a randomized, double-blind, placebo-controlled phase 2a trial. Clin Cosmet Investig Dermatol. 14:1507–1517. DOI: 10.2147/CCID.S319676. PMID: 34703265. PMCID: PMC8526366. PMID: https://www.scopus.com/inward/record.uri?partnerID=HzOxMe3b&scp=85120577218&origin=inward.


40. Wen S, Wei J, Bao J, Guo T, Zheng W, Zhuang X, Lin Y. 2020; Effect of levocetirizine hydrochloride on the growth of human dermal papilla cells: a preliminary study. Ann Palliat Med. 9:308–317. DOI: 10.21037/apm.2020.01.15. PMID: 32156129. PMID: https://www.scopus.com/inward/record.uri?partnerID=HzOxMe3b&scp=85082959422&origin=inward.


41. Johnstone MA. 1997; Hypertrichosis and increased pigmentation of eyelashes and adjacent hair in the region of the ipsilateral eyelids of patients treated with unilateral topical latanoprost. Am J Ophthalmol. 124:544–547. DOI: 10.1016/S0002-9394(14)70870-0. PMID: 9323945. PMID: https://www.scopus.com/inward/record.uri?partnerID=HzOxMe3b&scp=0030867340&origin=inward.


42. Coronel-Pérez IM, Rodríguez-Rey EM, Camacho-Martínez FM. 2010; Latanoprost in the treatment of eyelash alopecia in alopecia areata universalis. J Eur Acad Dermatol Venereol. 24:481–485. DOI: 10.1111/j.1468-3083.2009.03543.x. PMID: 20028444. PMID: https://www.scopus.com/inward/record.uri?partnerID=HzOxMe3b&scp=77749302366&origin=inward.


43. Faghihi G, Andalib F, Asilian A. 2009; The efficacy of latanoprost in the treatment of alopecia areata of eyelashes and eyebrows. Eur J Dermatol. 19:586–587. DOI: 10.1684/ejd.2009.0766. PMID: 19620039. PMID: https://www.scopus.com/inward/record.uri?partnerID=HzOxMe3b&scp=73249131977&origin=inward.


44. Ross EK, Bolduc C, Lui H, Shapiro J. 2005; Lack of efficacy of topical latanoprost in the treatment of eyebrow alopecia areata. J Am Acad Dermatol. 53:1095–1096. DOI: 10.1016/j.jaad.2005.06.031. PMID: 16310083. PMID: https://www.scopus.com/inward/record.uri?partnerID=HzOxMe3b&scp=27944434900&origin=inward.


45. Blume-Peytavi U, Lönnfors S, Hillmann K, Garcia Bartels N. 2012; A randomized double-blind placebo-controlled pilot study to assess the efficacy of a 24-week topical treatment by latanoprost 0.1% on hair growth and pigmentation in healthy volunteers with androgenetic alopecia. J Am Acad Dermatol. 66:794–800. DOI: 10.1016/j.jaad.2011.05.026. PMID: 21875758. PMID: https://www.scopus.com/inward/record.uri?partnerID=HzOxMe3b&scp=84859849330&origin=inward.


46. Rafati M, Mahmoudian R, Golpour M, Kazeminejad A, Saeedi M, Nekoukar Z. 2022; The effect of latanoprost 0.005% solution in the management of scalp alopecia areata, a randomized double-blind placebo-controlled trial. Dermatol Ther. 35:e15450. DOI: 10.1111/dth.15450. PMID: 35289043. PMID: https://www.scopus.com/inward/record.uri?partnerID=HzOxMe3b&scp=85126863575&origin=inward.


47. Yoelin S, Walt JG, Earl M. 2010; Safety, effectiveness, and subjective experience with topical bimatoprost 0.03% for eyelash growth. Dermatol Surg. 36:638–649. DOI: 10.1111/j.1524-4725.2010.01519.x. PMID: 20384751. PMID: https://www.scopus.com/inward/record.uri?partnerID=HzOxMe3b&scp=77951274423&origin=inward.


48. Wester ST, Lee WW, Shi W. 2010; Eyelash growth from application of bimatoprost in gel suspension to the base of the eyelashes. Ophthalmology. 117:1024–1031. DOI: 10.1016/j.ophtha.2009.10.017. PMID: 20163864. PMCID: PMC2864326. PMID: https://www.scopus.com/inward/record.uri?partnerID=HzOxMe3b&scp=77951623319&origin=inward.


49. Woodward JA, Haggerty CJ, Stinnett SS, Williams ZY. 2010; Bimatoprost 0.03% gel for cosmetic eyelash growth and enhancement. J Cosmet Dermatol. 9:96–102. DOI: 10.1111/j.1473-2165.2010.00487.x. PMID: 20618554. PMID: https://www.scopus.com/inward/record.uri?partnerID=HzOxMe3b&scp=77954445858&origin=inward.


50. Yoelin SG, Fagien S, Cox SE, Davis PG, Campo A, Caulkins CA, Gallagher CJ. 2014; A retrospective review and observational study of outcomes and safety of bimatoprost ophthalmic solution 0.03% for treating eyelash hypotrichosis. Dermatol Surg. 40:1118–1124. DOI: 10.1097/01.DSS.0000452658.83001.d9. PMID: 25229783. PMID: https://www.scopus.com/inward/record.uri?partnerID=HzOxMe3b&scp=84909583005&origin=inward.


51. Kwon O, Kim JY, Paik SH, Jeon HC, Jung YJ, Lee Y, Baek JH, Chun JH, Lee WS, Lee JY, Rogers JD, Halstead M, Eun HC. 2014; Long-term utility and durability of the therapeutic effects of bimatoprost 0.03% for eyelash augmentation in healthy Asian subjects. Dermatology. 229:222–229. DOI: 10.1159/000363379. PMID: 25228046. PMID: https://www.scopus.com/inward/record.uri?partnerID=HzOxMe3b&scp=84913615048&origin=inward.


52. Suwanchatchai W, Tanglertsampan C, Pengsalae N, Makornwattana M. 2012; Efficacy and safety of bimatoprost 0.03% versus minoxidil 3% in enhancement of eyebrows: a randomized, double-blind, split-face comparative study. J Dermatol. 39:865–866. DOI: 10.1111/j.1346-8138.2012.01579.x. PMID: 22594928. PMID: https://www.scopus.com/inward/record.uri?partnerID=HzOxMe3b&scp=84867098787&origin=inward.


53. Glaser DA, Hossain P, Perkins W, Griffiths T, Ahluwalia G, Weng E, Beddingfield FC. 2015; Long-term safety and efficacy of bimatoprost solution 0·03% application to the eyelid margin for the treatment of idiopathic and chemotherapy-induced eyelash hypotrichosis: a randomized controlled trial. Br J Dermatol. 172:1384–1394. DOI: 10.1111/bjd.13443. PMID: 25296533. PMCID: PMC4832276. PMID: https://www.scopus.com/inward/record.uri?partnerID=HzOxMe3b&scp=84929129951&origin=inward.


54. Vila TO, Camacho Martinez FM. 2010; Bimatoprost in the treatment of eyelash universalis alopecia areata. Int J Trichology. 2:86–88. DOI: 10.4103/0974-7753.77511. PMID: 21712909. PMCID: PMC3107964. PMID: https://www.scopus.com/inward/record.uri?partnerID=HzOxMe3b&scp=84861320499&origin=inward.


55. Zaheri S, Hughes B. 2010; Successful use of bimatoprost in the treatment of alopecia of the eyelashes. Clin Exp Dermatol. 35:e161–e162. DOI: 10.1111/j.1365-2230.2009.03755.x. PMID: 19925481. PMID: https://www.scopus.com/inward/record.uri?partnerID=HzOxMe3b&scp=77951247075&origin=inward.


56. Law SK. 2010; Bimatoprost in the treatment of eyelash hypotrichosis. Clin Ophthalmol. 4:349–358. DOI: 10.2147/OPTH.S6480. PMID: 20463804. PMCID: PMC2861943. PMID: https://www.scopus.com/inward/record.uri?partnerID=HzOxMe3b&scp=77953374959&origin=inward.


57. Roseborough I, Lee H, Chwalek J, Stamper RL, Price VH. 2009; Lack of efficacy of topical latanoprost and bimatoprost ophthalmic solutions in promoting eyelash growth in patients with alopecia areata. J Am Acad Dermatol. 60:705–706. DOI: 10.1016/j.jaad.2008.08.029. PMID: 19293023. PMID: https://www.scopus.com/inward/record.uri?partnerID=HzOxMe3b&scp=61949178512&origin=inward.


58. Borchert M, Bruce S, Wirta D, Yoelin SG, Lee S, Mao C, VanDenburgh A. 2016; An evaluation of the safety and efficacy of bimatoprost for eyelash growth in pediatric subjects. Clin Ophthalmol. 10:419–429. DOI: 10.2147/OPTH.S89561. PMID: 27022239. PMCID: PMC4792214. PMID: https://www.scopus.com/inward/record.uri?partnerID=HzOxMe3b&scp=84961148688&origin=inward.
59. Subedi L, Pandey P, Shim JH, Kim KT, Cho SS, Koo KT, Kim BJ, Park JW. 2022; Preparation of topical bimatoprost with enhanced skin infiltration and in vivo hair regrowth efficacy in androgenic alopecia. Drug Deliv. 29:328–341. DOI: 10.1080/10717544.2022.2027046. PMID: 35040730. PMCID: PMC8774136. PMID: 4ee435de62364ca4a794eb4b7e77d295. PMID: https://www.scopus.com/inward/record.uri?partnerID=HzOxMe3b&scp=85123354630&origin=inward.


60. Hwang JH, Chu H, Ahn Y, Kim J, Kim DY. 2019; HMGB1 promotes hair growth via the modulation of prostaglandin metabolism. Sci Rep. 9:6660. DOI: 10.1038/s41598-019-43242-2. PMID: 31040377. PMCID: PMC6491442. PMID: https://www.scopus.com/inward/record.uri?partnerID=HzOxMe3b&scp=85064925362&origin=inward.
61. Villarreal-Villarreal CD, Sinclair RD, Martínez-Jacobo L, Garza-Rodríguez V, Rodríguez-León SA, Lamadrid-Zertuche AC, Rodríguez-Gutierrez R, Ortiz-Lopez R, Rojas-Martinez A, Ocampo-Candiani J. 2019; Prostaglandins in androgenetic alopecia in 12 men and four female. J Eur Acad Dermatol Venereol. 33:e214–e215. DOI: 10.1111/jdv.15479. PMID: 30730082. PMID: https://www.scopus.com/inward/record.uri?partnerID=HzOxMe3b&scp=85062335498&origin=inward.


Fig. 1
Diagram of prostanoids and their biological effects on hair follicles through stimulating different type of G protein-coupled receptors.
Prostanoids are classified into five types, namely thromboxane A2 (TXA2), prostaglandin D2 (PGD2), prostaglandin E2 (PGE2), prostaglandin F2α (PGF2α), and prostaglandin I2 (PGI2). PGD2 binds to prostaglandin D2 receptors (DP1, DP2), whereas PGE2 binds to prostaglandin E2 receptors (EP1, EP2, EP3, EP4). PGF2α binds to the prostaglandin F2α receptor (FP). PGI2 binds to the prostaglandin I2 receptor (IP). TXA2 binds to the thromboxane A2 receptor (TP). Human hair follicles remarkably expressed microsomal PGES (mPGES)-1, mPGES-2, and cytosolic PGES (cPGES), which converts PGH2 to PGE2. These human hair follicles also significantly expressed AKR1C3/PGFS, which catalyzes PGH2 to PGF2α. In addition, cyclooxygenase-1 (COX-1), cyclooxygenase-2 (COX-2), prostaglandin G2 (PGG2), prostaglandin H2 (PGH2), PGD2 synthase (PGDS), PGE2 synthase (PGES), PGF2α synthase (PGFS), PGI2 synthase (PGIS) are marked.
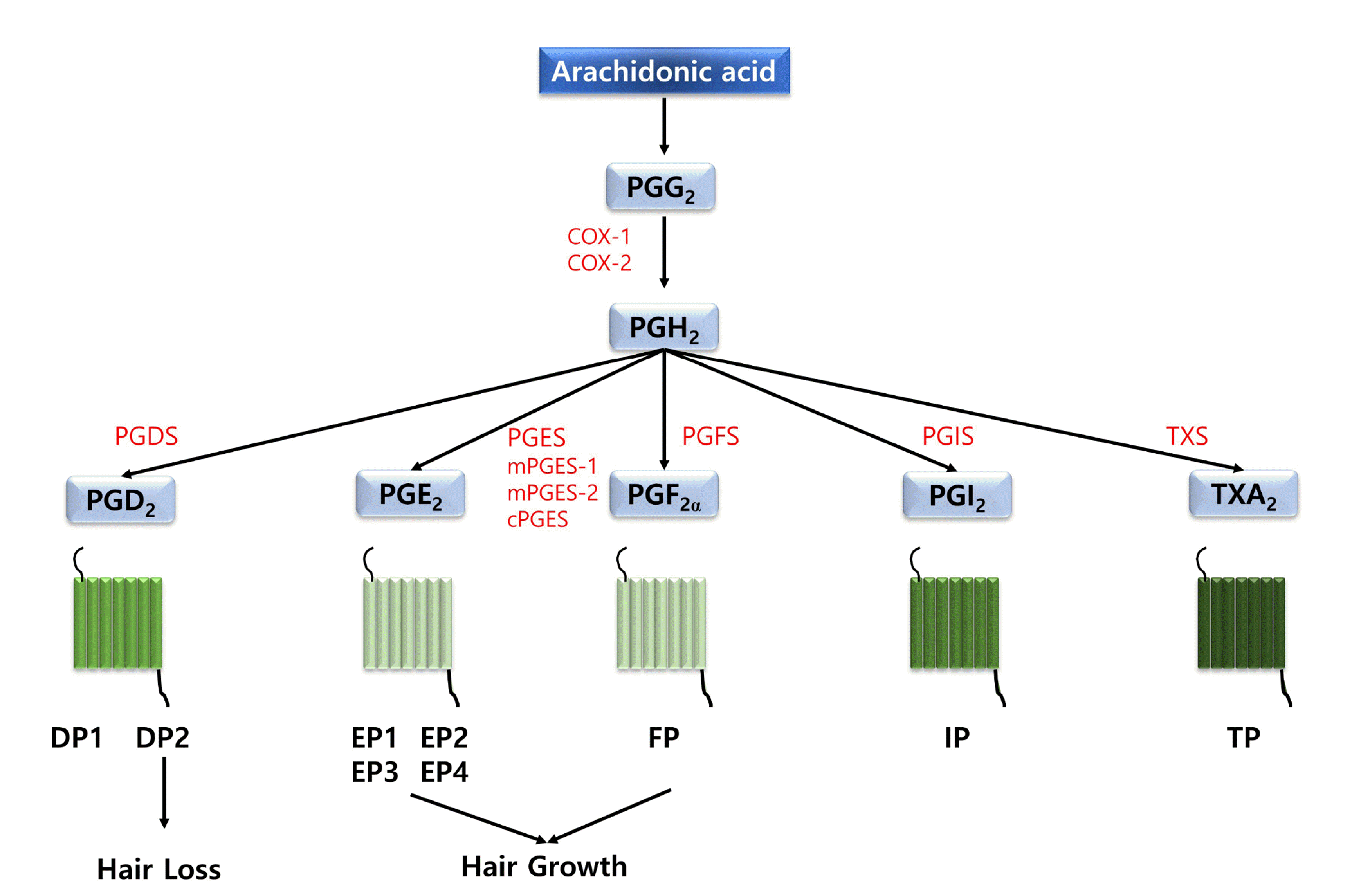
Table 1
Chemical compounds or medicines for improving hair loss
Compound name (type) |
Subjects | Working concentration & condition | Positive effects or molecular targets | References |
---|---|---|---|---|
Cetirizine(Anti-histamine, PGD2 inhibitor) | 60 patients with AGA | 1% cetirizine-treated group for 6 months | ↑ Hair growth | [37] |
40 males with AGA | 1% cetirizine solution for 16 weeks | ↑ Total & vellus hair density | [38] | |
↑ Anagen phase ratio | ||||
67 males with AGA | Topical 1% cetirizine per daily | ↑ Total hair density | [61] | |
↑ Terminal hair density | ||||
Setipiprant(DP2 antagonist) | 83 males with AGA | Phase 2a clinical trial 500 mg twice a day for 24 weeks | Safety and tolerability | [39] |
Not significant efficacy | ||||
Levocetirizinehydrochloride (Anti-histamine, PGD2 inhibitor) | Human dermal papilla cells | 1, 10, 100, 1,000, and 10,000 ng/ml for 24 h | ↓ PDG2, PTGDS, DP2 | [40] |
↑ PGF2α, | ||||
↑ pAKT, pGSK3β | ||||
Latanoprost(PGF2α analog) | 43 patients with glaucoma | Unilateral topical 0.1% latanoprost administration for 10 weeks | ↑ Hypertrichosis, | [41] |
↑ Pirgmentation | ||||
54 males with alopecia areta | Latanoprost 0.005% (50 μg/ml) ophthalmic solution for 3 months to 2 year | ↑ Hair regrowth | [42] | |
16 males with AGA | 0.1% latanoprost in males with mild AGA for 24 weeks | ↑ Hair density, hair growth | [45] | |
↑ Hair diameter | ||||
30 males with alopecia areta | Topical 0.005% latanoprost for 12 weeks | ↑ Hair density | [46] | |
↑ Hair regrowth | ||||
Bimatoprost(PGF2α analog) | 585 subjects | 0.03% ophthalmic bimatoprost solution for at least 12 months | ↑ Eyelashes regrowth | [50] |
30 patients | 0.03% bimatoprost ophthalmic solution for 1 year | ↑ Eyebrow thickness | [52] | |
238 patients with idiopathic hypotrichosis | 0.03% bimatoprost ophthalmic solution for 1 year | ↑ Eyelash regrowth | [53] | |
36 patients with alopecia areata 40 subjects | 0.03% bimatoprost for 6 months | ↑ Eyelash regrowth | [54] | |
Human dermal papilla cells | 0.03% bimatoprost | ↑ Eyelash regrowth in healthy adolescents | [58] | |
AGA mouse model(MaleC57BL/6J mice) | 5 μM bimatoprost in solvent mixture system | ↑ Cell proliferation | ||
0.3%–5% bitmatoprost formulation for 14 days | ↑ Hair regrowth | [59] | ||
↑ Hair weight | ||||
↑ Diameter & number of Hair follicles |