Abstract
Background
The treatment of trigeminal neuralgia remains a challenging issue. Stem cells from human exfoliated deciduous teeth (SHED) provide optimized therapy for chronic pain. This study aimed to investigate the mechanisms underlying the attenuation of trigeminal neuralgia by SHED.
Methods
Trigeminal neuralgia was induced by chronic constriction injury of the infraorbital nerve. The mechanical threshold was assessed after model establishment and local SHED transplantation. Endoplasmic reticulum (ER) morphology and Caspase12 expression in trigeminal ganglion (TG) was evaluated as well. BiP expression was observed in PC12 cells induced by tunicamycin.
Results
The local transplantation of SHED could relieve trigeminal neuralgia in rats. Further, transmission electron microscopy revealed swelling of the ER in rats with trigeminal neuralgia. Moreover, SHED inhibited the tunicamycin-induced up-regulated expression of BiP mRNA and protein in vitro. Additionally, SHED decreased the up-regulated expression of Caspase12 mRNA and protein in the TG of rats caused by trigeminal neuralgia after chronic constriction injury of the infraorbital nerve mode.
Trigeminal neuralgia (TN) refers to neuropathic pain originating from the trigeminal nerve. It is among the most common facial pain syndromes and is characterized by recurrent, sporadic, stabbing, paroxysmal, and burning pain in the orofacial region [1]. Although this neuropathic pain is common, its etiology remains unclear.
Stem cells from human exfoliated deciduous teeth (SHED), which represent a group of immature stem cells, can be easily obtained and isolated using noninvasive procedures [2]. SHED can stay undifferentiated and stable without alterations in their biological and immunological features for a long time, and therefore are important in tissue engineering and stem cell therapy [3]. Under non-neural induction conditions, SHED can express neural markers, including nestin, glutamate decarboxylase, neuron-specific nucleoprotein, glial fibrillary acidic protein, and neurofilament protein, which could be attributed to the dental pulp originating from the neural crest [4]. SHED not only express the early neural marker, nestin, but also later neuron-related enzymes under neural induction culture conditions. SHED have significant nerve regeneration activity and can promote functional recovery after spinal cord injury. Studies have shown that SHED transplantation into a rat model of spinal cord injury significantly improved the recovery of hind limb motor function and inhibited the apoptosis of neurons, astrocytes, and oligodendrocytes; further, it preserved neurofilaments and myelin sheath in the peri-injury site [5,6]. Given the neurogenic differentiation in vivo and neural crest origin of SHED, researchers provide an alternative model for treating chronic pain, including TN. The authors previously showed that SHED are crucially involved in attenuating TN; however, the underlying mechanisms remain unclear [7].
Endoplasmic reticulum (ER) stress refers to a disorder of the protein folding process in the ER lumen, which essentially activates the unfolded protein response (UPR). ER stress and UPR are involved in neurodegenerative diseases and are closely associated with chronic pain [8]. Moreover, continuous activation of ER stress may be involved in neuronal injury in response to neuropathic pain stimuli. ER stress associated with neuropathic pain could induce cell apoptosis resulting from neuronal injury through two pathways: namely, the IRE1/ASK1/JNK pathway and the Caspase12 kinase pathway [9]. Caspase12 is crucially involved in cell death induced by ER stress, where its expression is increased. BiP, which is also known as GRP78, is the most commonly expressed molecular chaperone protein in early-stage ER stress and is considered a marker protein of ER stress [10]. However, the relationship of ER stress with TN and whether SHED can attenuate TN by regulating ER stress remains unclear.
This study aimed to investigate whether SHED could increase the mechanical threshold by inhibiting ER stress via the regulation of BiP and Caspase12. This could provide preclinical evidence supporting the use of dental stem cells in the treatment of TN.
Human exfoliated deciduous incisors obtained from children aged 6–8 years were collected as discarded experimental samples in the Hospital of Stomatology, China Medical University. Before obtaining SHED from the samples, approval was obtained from an ethical committee as per the Institutional Review Board guidelines and informed consent from the children’s parents. After the pulp was removed from the incisors, it was quickly placed in a precooled medium containing 100 U/mL penicillin/streptomycin double-antibody. Subsequently, the dental pulp was repeatedly rinsed using phosphate buffer solution (HyClone, Logan, UT) containing the double-antibody and chopped with a sterile scalpel to form a paste [11]. The minced dental pulp was transferred to a centrifuge tube containing 3 mg/mL type I collagenase (Worthington Biochemical Co, Lakewood, CO) and 4 mg/mL neutral protease (Sigma-Aldrich, St Louis, MO), digested at 37°C for 30 minutes, and vortexed at 10-min intervals until the tissue was no longer visible. Next, an equal volume of medium was added for neutralization, followed by centrifugation at 1,500 rpm for 5 minutes. The supernatant was then discarded, and medium was added for resuspension. A single-cell suspension was obtained using a 70-µm cell sieve (Merck Millipore Ltd, Cork, Ireland) and inoculated in a 10-cm petri dish at a density of 1 × 104 cells/mL. Next, fetal bovine serum (Gibco, Waltham, MA), L-glutamate (Hyclone), L-ascorbic acid 2-phosphate (Sigma-Aldrich), and penicillin/streptomycin in aMEM (Hyclone) were added and placed in a cell culture incubator (37°C and 5% CO2). When the cells grow to 80% for passage, they will be used for subsequent experiments in their third and fifth generations. The cells used in the present study belonged to the same batch used in the authors’ previous flow cytometry study, which demonstrated that SHED have the potential for osteogenic and neurogenic differentiation [7].
In the co-cultivation experiment between SHED and PC12 cells (from rat adrenal pheochromocytoma), a micropore with a diameter of 0.4 μm was used in the Transwell chamber for establishing an indirect cell co-culture system. During the third to fifth passages, SHED were inoculated to the upper chamber of the 6-well plate at a density of 1 × 105 cells/mL. Moreover, PC12 cells were adjusted to 1 × 105 cells/mL before inoculation to the orifice plate under the chamber. When the cells were completely adherent after 24 hours, 1 μg/mL tunicamycin (TM, a maturity ER stress model in vitro) were added to PC12 cells in the lower chamber as the SHED group (SHED co-culture). Further, PC12 cells were cultured alone as the control group. Each group was cultured in three holes and the samples were collected after 12 hours for subsequent experiments.
All the experiments met the basic management regulations and ethics of animal experiments of China Medical University (CMU2020192). Forty healthy 8-week-old male Sprague-Dawley rats, weighing 240–280 g, were provided by Speyford Biotechnology Co. Ltd. (Beijing, China) and housed in the laboratory of the Animal Center of China Medical University. They were put in a standard cycle of alternating 12 hours of illumination and darkness, with adequate food and water. A rat model of TN was established through chronic constriction injury of the infraorbital nerve (CCI-ION). All operations were performed under general anesthesia induced by intraperitoneal injection of 2% sodium pentobarbital (40 mg/kg). The rats were placed in a supine position on the operating table to allow extensive exposure of the oral structure. A surgical incision was made in the mouth to avoid damaging the hair, skin, and whiskers. Nerve ligation was performed using two 4-0 chromic catguts. The surgical incision was closed using 4-0 sutures. In the sham group, the infraorbital nerve was only separated rather than ligated. All operations were aseptically performed without the use of antibiotics [12].
After acclimating to the cages for one week, the rats were randomly divided into a sham group, CCI-ION group, CCI-ION + SHED group, and CCI-ION + Medium group. There were 6 rats in each group. Three days after nerve ligation, the rats in the CCI-ION + SHED group were locally injected once with SHED (5 × 105 cells, 200 μL, n = 6 rats) at the surgical site, while rats in the CCI-ION + Medium group were locally injected with serum-free medium (200 μL, n = 6 rats). It is worth noting that SHED and serum-free medium were injected along the intraoral buccal mucosa suture of the rats and infiltrated to the vicinity of the ligated nerve. Rats in the sham group and CCI-ION group did not receive injections. The injection time point was 3 days after ligation. And 14 days after transplantation, the rats were euthanized through an intraperitoneal injection of 70 mg/kg sodium pentobarbital.
The mechanical threshold was assessed using a calibrated von Frey filament. The results were assessed using the same implement by blinded investigators. A suite of calibrated von Frey filaments (weight: 2–15 g) was used to apply pressure on the skin above the infraorbital nerve by bending the filaments. A positive response was indicated by the active withdrawal of the paw and head from the von Frey filament. The test lasted until the rats showed five positive responses to the same filament. The relevant response frequencies ([quantity of positive responses/quantity of total stimuli] × 100%) were calculated; subsequently, a curve of the stimulus-response frequency was plotted. The EF50 value, which is a measure of the mechanical threshold, represents the filament pressure yielding a 50% response frequency based on the plotted curve. An increase in the EF50 value is indicative of relief from mechanical allodynia.
The rats were euthanized under deep anesthesia, followed by cutting open the thoracic cavity, exposing the heart and aorta, carefully inserting a needle into the left ventricle, fixing the needle tip at the beginning of the aorta, and perfusing the right atrium with 0.9% saline solution until the saline from the right atrium became clear. Overnight perfusion was performed using 4% paraformaldehyde. Subsequently, the intact trigeminal ganglion (TG) was placed in 4% paraformaldehyde and fixed overnight. Next, a long tissue with a 1-mm³ block was obtained from the end of the TG and soaked in 1% OSO4 and uranyl acetate for 1 hour and 2 hours, respectively. Subsequently, gradient dehydration was performed using ethanol and propylene oxide, followed by tissue embedding with epoxy resin. Ultrathin tissue sections were stained using uranyl acetate. Tissues were observed and photographed using a Hitachi H-7650 transmission electron microscope (Hitachi, Tokyo, Japan).
After the rats were euthanized under deep anesthesia, 1 cm of the trigeminal nerve was obtained, placed in a refrigerator at –80°C, and the total RNA extracted using the Trizol method. Next, the cells were collected and the culture medium aspirated, followed by washing the cells twice with phosphate-buffered saline. Subsequently, 1 mL Trizol was added to each sample and the cells were thoroughly mixed by pipetting. A fresh 1.5-mL sterile enzyme-free Ep tube was prepared for transferring each sample for RNA reverse transcription. The primer designs were as follows: Caspase12: ATTCCTGGTCTTTATGTCCC, TCATCTGTATCAGCAGTGGC; BiP: CGGAGGAGGAGGACAAGAAGGAG, ATACGACGGTGTGATGCGGTTG; ACTB: CGTTGACATCCGTAAAGAC, CTGGAAGGTGGACAGTGAG. The conditions for the real-time polymerase chain reaction were as follows: pre-denaturation at 95°C for 30 seconds; 40 cycles of 95°C for 5 seconds and 60°C for 30 seconds; and finally, 95°C for 15 seconds, 60°C for 30 seconds, and 95°C for 15 seconds. There were three duplicating holes for each sample and the tests were performed in triplicate.
Initially, denatured total protein was extracted. For protein quantification, based on the number of samples, 50 volumes of A solution plus 1 volume of B solution (50:1) were used to make a BCA working solution (Beyotime, Shanghai, China). After the absorbance value was determined, the protein concentration and absorbance value curves were plotted to determine the optimal protein concentration. For sodium dodecyl sulfate-polyacrylamide gel electrophoresis, after polymerization of the concentrated gel, pre-stained Marker (Thermo Fisher Scientific, Waltham, MA) was added to the sample well, mixed, and transferred to the membrane. The polyvinylidene fluoride membrane was placed into a dish with 5% blocking solution using skimmed milk. After blocking, the membrane was incubated in the dish with the primary antibody Caspase12/BiP (Abcam, Cambridge, UK) overnight at 4°C. On the next day, it was removed, washed with tris-buffered saline Tween 20, and incubated with the secondary antibody (Abcam). The reaction occurred on a shaker at room temperature for 1–2 hours. Finally, luminescence was achieved using the liquid in the ECL kit.
Data are expressed as the mean ± standard error of the mean. Data regarding the mechanical threshold were analyzed using a two-way repeated-measures analysis of variance (ANOVA). Between- and among-group comparisons were calculated using Student’s t-test and one-way ANOVA, respectively. SigmaPlot 14.0 (Systat, Palo Alto, CA) was used to create the artwork. Statistical significance was set at P < 0.05.
The CCI-ION group showed a significantly decreased mechanical threshold at 3, 6, 10, and 17 days after nerve ligation (P < 0.001, for all timepoints). As shown in Fig. 1, the TN model was successfully established; moreover, mechanical allodynia in this model could last for 8 weeks [13]. Also, after local SHED injection on Day 3, there was a significant increase in the mechanical threshold at 6, 10, and 17 days after nerve ligation (P < 0.001, for all time points). Compared with the control group, the SHED group showed a significantly increased mechanical threshold at 6, 10, and 17 days after nerve ligation (P < 0.001, for all timepoints). This indicates that SHED may reverse the mechanical hypersensitivity and allodynia. Medium administration did not significantly alter the mechanical threshold compared with the baseline level.
We examined the TG tissue of the rats in the CCI-ION group and sham group through transmission electron microscopy. This indicated that the sham group showed no obvious abnormal ER morphology as shown in Fig. 2A and trigeminal nerve ligation caused ER swelling and abnormal formation as shown in Fig. 2B. Changes in ER morphology suggest that ER stress response may be involved in the process of TN.
Compared with the control group, the TM group showed significantly up-regulated BiP mRNA (P = 0.033, Fig. 3A) and protein (P = 0.012, Fig. 3B) expression after 24-hr TM stimulation, which suggests that TM could increase BiP expression in PC12 cells and that the degree of ER stress in neuronal cell is greatly increased. Compared with the TM group, SHED administration significantly inhibited upregulated BiP mRNA (P = 0.013, Fig. 3A) and protein (P = 0.006, Fig. 3B) expression, which suggests that SHED may inhibit upregulated BiP expression in PC12 cells and the degree of ER stress in neuronal cell is inhibited as well. These findings suggest that SHED may inhibit TM-induced upregulated BiP expression in PC12 cells.
Compared with the sham group, the CCI-ION group showed significantly upregulated Caspase12 mRNA (P = 0.006, Fig. 4A) and protein (P = 0.005, Fig. 4B) expression in the TG at 14 days after nerve ligation. This suggests that infraorbital nerve ligation could increase Caspase12 expression in the TG, indicating that the violence of ER stress in the TG greatly increased. Compared with the CCI-ION group, the SHED group showed inhibition of the up-regulation of Caspase12 mRNA (P = 0.001, Fig. 4A) and protein (P = 0.009, Fig. 4B) expression at 14 days after SHED transplantation. This suggests that local SHED transplantation may inhibit the up-regulation of Caspase12 expression, and that the violence of ER stress in the TG greatly inhibited. SHED may inhibit the Caspase12 expression and the violence of ER stress caused by infraorbital nerve ligation.
This study’s findings suggest that SHED could alleviate TN by regulating BiP and Caspase12 expression induced by ER stress, which could provide alternative treatment strategies for TN.
Stem cells, which are self-replicating cells with various differentiation potentials, have been used to treat a variety of neurological diseases, including Parkinson’s disease, Alzheimer’s disease, schizophrenia, and pain [14,15]. Direct injection of bone marrow mesenchymal stem cells (BMMSCs) into the lesions of CCI-ION and sciatic nerve CCI rat models was found to increase their mechanical and thermal thresholds [16,17]. Additionally, intrathecal or intraspinal injection of BMMSCs can alleviate pain [18]. Further, dental stem cells have shown reparative effects in spinal cord injury models [19,20]. SHED have better proliferative capabilities than BMMSCs. In a previous study by the authors, local SHED transplantation to the region of the injured nerve increased the mechanical threshold of TN, which indicates that SHED could have good antinociceptive effects. Further, the study’s findings demonstrated that SHED transplantation suppresses TN through c-Jun downregulation [13].
Additionally, intravenous injection of SHED or conditioned medium from SHED via the left external jugular vein was found to revert mechanical allodynia caused by spinal nerve transection [21]. SHED administration can also relieve diabetic neuropathic pain in diabetic Goto-Kakizaki rats and significantly improve limb function [22]. In nude mice, intraperitoneally injected SHED can be distributed to various tissues and organs, including the liver, spleen, and kidney [23]. SHED cannot only differentiate into teeth, bone, cartilage, and fat, among others, they can also become nerve cells under certain induction conditions. SHED originate from the neural crest, which can differentiate into neural tissue and can, therefore, be used to treat neuropathic diseases. Striatal SHED transplantation in a Parkinson’s rat model leads to detectable active cells in the brain, followed by alleviation of Parkinson’s symptoms [24]. The neurological regenerative activity of SHED involves inhibiting neuronal apoptosis and promoting axon regeneration [19]. Further, lost cells are replaced by differentiated gliocytes under extreme conditions. However, this is the first study to investigate whether SHED could alleviate TN. The TN model was successfully established by ligating the infraorbital nerve in rats, which significantly reduced the mechanical threshold. Local SHED transplantation reversed this hypersensitivity, indicating that SHED could relieve TN symptoms.
The ER is a highly dynamic organelle that is crucial in coordinating signal transduction pathways to ensure cell adaptation, resilience, and survival. ER stress is caused by the accumulation of unfolded or misfolded proteins and the resulting changes in the internal environment could cause imbalanced ER function, which induces UPR [25]. UPR requires mediation by three cardinal ER stress receptor proteins, namely inositol requiring enzyme 1, activating transcription factor 6 (ATF-6), and pancreatic ER kinase-like ER kinase. Intrathecal injection of the siRNA of ATF-6 can attenuate neuropathic pain, which demonstrates the crucial role of ER stress in inducing neuropathic pain [26]. ER stress in the dorsal root ganglion contributes to the development of pain hypersensitivity after nerve injury [27]. Moreover, ER stress in the peripheral nervous system is a significant driver of neuropathic pain [28]. ER stress significantly contributes to pain hypersensitivity. The role of ER stress in neuropathic pain has been demonstrated in a rat model of orofacial inflammatory pain. Specifically, injection of complete Freund’s adjuvant was found to increase ER stress in the TG by decreasing heat hyperalgesia, which suggests that induction of ER stress in the primary sensory neurons could be involved in orofacial pain. ER stress and UPR have been examined in a rat model of neuropathic pain induced by L5 spinal nerve ligation; additionally, nerve ligation contributes to activation of BiP, followed by the IRE-XBP1 and ATF6 pathways. In this study, transmission electron microscopy revealed obvious ER swelling in the TG of rats in the CCI-ION group. Moreover, rats in the CCI-ION group showed increased Caspase12 mRNA and protein expression in the TG, which indicates the involvement of ER stress in TN.
Increased BiP expression in the dorsal horn leads to the induction of ER stress in response to neuronal injury. The authors’ in vitro study revealed that TM stimulation significantly upregulated BiP mRNA and protein expression in PC12 cells; moreover, SHED inhibited upregulated BiP mRNA and protein expression. Caspase12 precursor (procaspase12), which is located on the side of the ER close to the cytoplasm, cleaves and specifically activates Caspase12 during ER stress. Caspase12 was the first identified member of the Caspase family and is strongly correlated with the ER [29]. During cell apoptosis induced by ER stress, there is activation of Caspase12 present in the ER. Cytoplasmic Caspase3 is then activated, which finally triggers cell apoptosis [30]. There is increased Caspase12 expression only during the occurrence of ER stress. In the present in vivo study, Caspase12 mRNA and protein expression were significantly upregulated after nerve ligation; additionally, local SHED transplantation reverted the TN hypersensitivity by effectively downregulating Caspase12 mRNA and protein expression. Taken together, this study’s findings demonstrate that SHED could inhibit ER stress by downregulating BiP and Caspase12 expression, providing novel information for the treatment of TN.
In conclusion, these findings demonstrate that SHED could reverse hypersensitivity by inhibition of ER stress via downregulating BiP and Caspase12 expression. These results provide preclinical evidence supporting the use of dental stem cells in TN treatment. However, the detailed mechanisms of how SHED contributes to ER stress for analgesic effect in TN are topics of further investigation.
Notes
REFERENCES
1. Bendtsen L, Zakrzewska JM, Heinskou TB, Hodaie M, Leal PRL, Nurmikko T, et al. 2020; Advances in diagnosis, classification, pathophysiology, and management of trigeminal neuralgia. Lancet Neurol. 19:784–96. DOI: 10.1016/S1474-4422(20)30233-7. PMID: 32822636. PMID: https://www.scopus.com/inward/record.uri?partnerID=HzOxMe3b&scp=85089515336&origin=inward.


2. Anoop M, Datta I. 2021; Stem cells derived from human exfoliated deciduous teeth (SHED) in neuronal disorders: a review. Curr Stem Cell Res Ther. 16:535–50. DOI: 10.2174/1574888X16666201221151512. PMID: 33349220. PMID: https://www.scopus.com/inward/record.uri?partnerID=HzOxMe3b&scp=85113789414&origin=inward.


3. Fuloria S, Jain A, Singh S, Hazarika I, Salile S, Fuloria NK. 2021; Regenerative potential of stem cells derived from human exfoliated deciduous (SHED) teeth during engineering of human body tissues. Curr Stem Cell Res Ther. 16:507–17. DOI: 10.2174/1574888X16999201231213206. PMID: 33390148. PMID: https://www.scopus.com/inward/record.uri?partnerID=HzOxMe3b&scp=85114056149&origin=inward.


4. Fayazi N, Sheykhhasan M, Soleimani Asl S, Najafi R. 2021; Stem cell-derived exosomes: a new strategy of neurodegenerative disease treatment. Mol Neurobiol. 58:3494–514. DOI: 10.1007/s12035-021-02324-x. PMID: 33745116. PMCID: PMC7981389. PMID: https://www.scopus.com/inward/record.uri?partnerID=HzOxMe3b&scp=85103188198&origin=inward.


5. Kunimatsu R, Nakajima K, Awada T, Tsuka Y, Abe T, Ando K, et al. 2018; Comparative characterization of stem cells from human exfoliated deciduous teeth, dental pulp, and bone marrow-derived mesenchymal stem cells. Biochem Biophys Res Commun. 501:193–8. DOI: 10.1016/j.bbrc.2018.04.213. PMID: 29730288. PMID: https://www.scopus.com/inward/record.uri?partnerID=HzOxMe3b&scp=85046699495&origin=inward.


6. Nakamura S, Yamada Y, Katagiri W, Sugito T, Ito K, Ueda M. 2009; Stem cell proliferation pathways comparison between human exfoliated deciduous teeth and dental pulp stem cells by gene expression profile from promising dental pulp. J Endod. 35:1536–42. DOI: 10.1016/j.joen.2009.07.024. PMID: 19840643. PMID: https://www.scopus.com/inward/record.uri?partnerID=HzOxMe3b&scp=70349973455&origin=inward.


7. Bai X, Zhang X, Wang C, Liu Y, Liu X, Fan Y, et al. 2021; Stem cells from human exfoliated deciduous teeth attenuate trigeminal neuralgia in rats. Stem Cells Int. 2021:8819884. DOI: 10.1155/2021/8819884. PMID: 33531911. PMCID: PMC7834821. PMID: https://www.scopus.com/inward/record.uri?partnerID=HzOxMe3b&scp=85100570726&origin=inward.


8. Bettigole SE, Glimcher LH. 2015; Endoplasmic reticulum stress in immunity. Annu Rev Immunol. 33:107–38. DOI: 10.1146/annurev-immunol-032414-112116. PMID: 25493331. PMID: https://www.scopus.com/inward/record.uri?partnerID=HzOxMe3b&scp=84927636122&origin=inward.


9. Zhang D, Zhou Q, Huang D, He L, Zhang H, Hu B, et al. 2019; ROS/JNK/c-Jun axis is involved in oridonin-induced caspase-dependent apoptosis in human colorectal cancer cells. Biochem Biophys Res Commun. 513:594–601. DOI: 10.1016/j.bbrc.2019.04.011. PMID: 30981511. PMID: https://www.scopus.com/inward/record.uri?partnerID=HzOxMe3b&scp=85064059670&origin=inward.


10. Jin H, Komita M, Aoe T. 2017; The role of BiP retrieval by the KDEL receptor in the early secretory pathway and its effect on protein quality control and neurodegeneration. Front Mol Neurosci. 10:222. DOI: 10.3389/fnmol.2017.00222. PMID: 28769758. PMCID: PMC5511815. PMID: https://www.scopus.com/inward/record.uri?partnerID=HzOxMe3b&scp=85026666513&origin=inward.


11. Wu M, Liu X, Li Z, Huang X, Guo H, Guo X, et al. 2021; SHED aggregate exosomes shuttled miR-26a promote angiogenesis in pulp regeneration via TGF-β/SMAD2/3 signalling. Cell Prolif. 54:e13074. DOI: 10.1111/cpr.13074. PMID: 34101281. PMCID: PMC8249784. PMID: https://www.scopus.com/inward/record.uri?partnerID=HzOxMe3b&scp=85107328671&origin=inward.


12. Krzyzanowska A, Pittolo S, Cabrerizo M, Sánchez-López J, Krishnasamy S, Venero C, et al. 2011; Assessing nociceptive sensitivity in mouse models of inflammatory and neuropathic trigeminal pain. J Neurosci Methods. 201:46–54. DOI: 10.1016/j.jneumeth.2011.07.006. PMID: 21782847. PMID: https://www.scopus.com/inward/record.uri?partnerID=HzOxMe3b&scp=80052317834&origin=inward.


13. Bai X, Xiao K, Yang Z, Zhang Z, Li J, Yan Z, et al. 2022; Stem cells from human exfoliated deciduous teeth relieve pain via downregulation of c-Jun in a rat model of trigeminal neuralgia. J Oral Rehabil. 49:219–27. DOI: 10.1111/joor.13243. PMID: 34386989. PMID: https://www.scopus.com/inward/record.uri?partnerID=HzOxMe3b&scp=85112710415&origin=inward.


14. Shetty AK, Bates A. 2016; Potential of GABA-ergic cell therapy for schizophrenia, neuropathic pain, and Alzheimer's and Parkinson's diseases. Brain Res. 1638(Pt A):74–87. DOI: 10.1016/j.brainres.2015.09.019. PMID: 26423935. PMCID: PMC5313260. PMID: https://www.scopus.com/inward/record.uri?partnerID=HzOxMe3b&scp=84951202711&origin=inward.


15. Han YH, Kim KH, Abdi S, Kim TK. 2019; Stem cell therapy in pain medicine. Korean J Pain. 32:245–55. DOI: 10.3344/kjp.2019.32.4.245. PMID: 31569916. PMCID: PMC6813895. PMID: https://www.scopus.com/inward/record.uri?partnerID=HzOxMe3b&scp=85074338727&origin=inward.


16. Guo W, Wang H, Zou S, Gu M, Watanabe M, Wei F, et al. 2011; Bone marrow stromal cells produce long-term pain relief in rat models of persistent pain. Stem Cells. 29:1294–303. DOI: 10.1002/stem.667. PMID: 21630378. PMCID: PMC3277433. PMID: https://www.scopus.com/inward/record.uri?partnerID=HzOxMe3b&scp=79960864273&origin=inward.


17. Abrams MB, Dominguez C, Pernold K, Reger R, Wiesenfeld-Hallin Z, Olson L, et al. 2009; Multipotent mesenchymal stromal cells attenuate chronic inflammation and injury-induced sensitivity to mechanical stimuli in experimental spinal cord injury. Restor Neurol Neurosci. 27:307–21. DOI: 10.3233/RNN-2009-0480. PMID: 19738324. PMID: https://www.scopus.com/inward/record.uri?partnerID=HzOxMe3b&scp=70349159499&origin=inward.


18. Watanabe S, Uchida K, Nakajima H, Matsuo H, Sugita D, Yoshida A, et al. 2015; Early transplantation of mesenchymal stem cells after spinal cord injury relieves pain hypersensitivity through suppression of pain-related signaling cascades and reduced inflammatory cell recruitment. Stem Cells. 33:1902–14. DOI: 10.1002/stem.2006. PMID: 25809552. PMID: https://www.scopus.com/inward/record.uri?partnerID=HzOxMe3b&scp=84929832810&origin=inward.


19. Sakai K, Yamamoto A, Matsubara K, Nakamura S, Naruse M, Yamagata M, et al. 2012; Human dental pulp-derived stem cells promote locomotor recovery after complete transection of the rat spinal cord by multiple neuro-regenerative mechanisms. J Clin Invest. 122:80–90. DOI: 10.1172/JCI59251. PMID: 22133879. PMCID: PMC3248299. PMID: https://www.scopus.com/inward/record.uri?partnerID=HzOxMe3b&scp=84855453812&origin=inward.


20. Nicola FC, Rodrigues LP, Crestani T, Quintiliano K, Sanches EF, Willborn S, et al. 2016; Human dental pulp stem cells transplantation combined with treadmill training in rats after traumatic spinal cord injury. Braz J Med Biol Res. 49:e5319. DOI: 10.1590/1414-431x20165319. PMID: 27509306. PMCID: PMC4988478. PMID: 5b3671fd05174e618020465b674c2eb6. PMID: https://www.scopus.com/inward/record.uri?partnerID=HzOxMe3b&scp=84981351813&origin=inward.


21. Hayashi Y, Kato H, Nonaka K, Nakanishi H. 2021; Stem cells from human exfoliated deciduous teeth attenuate mechanical allodynia in mice through distinct from the siglec-9/MCP-1-mediated tissue-repairing mechanism. Sci Rep. 11:20053. DOI: 10.1038/s41598-021-99585-2. PMID: 34625639. PMCID: PMC8501097. PMID: 81169b5e56444b048baab02f84851c29. PMID: https://www.scopus.com/inward/record.uri?partnerID=HzOxMe3b&scp=85116787082&origin=inward.


22. Xie J, Rao N, Zhai Y, Li J, Zhao Y, Ge L, et al. 2019; Therapeutic effects of stem cells from human exfoliated deciduous teeth on diabetic peripheral neuropathy. Diabetol Metab Syndr. 11:38. DOI: 10.1186/s13098-019-0433-y. PMID: 31131042. PMCID: PMC6525430. PMID: 1eed269eada94797b1d53af62e6d0202. PMID: https://www.scopus.com/inward/record.uri?partnerID=HzOxMe3b&scp=85065879132&origin=inward.


23. Huang GT, Gronthos S, Shi S. 2009; Mesenchymal stem cells derived from dental tissues vs. those from other sources: their biology and role in regenerative medicine. J Dent Res. 88:792–806. DOI: 10.1177/0022034509340867. PMID: 19767575. PMCID: PMC2830488. PMID: https://www.scopus.com/inward/record.uri?partnerID=HzOxMe3b&scp=70349510607&origin=inward.


24. Wang J, Wang X, Sun Z, Wang X, Yang H, Shi S, et al. 2010; Stem cells from human-exfoliated deciduous teeth can differentiate into dopaminergic neuron-like cells. Stem Cells Dev. 19:1375–83. DOI: 10.1089/scd.2009.0258. PMID: 20131979. PMCID: PMC3073455. PMID: https://www.scopus.com/inward/record.uri?partnerID=HzOxMe3b&scp=77956449630&origin=inward.


25. Wu FL, Liu WY, Van Poucke S, Braddock M, Jin WM, Xiao J, et al. 2016; Targeting endoplasmic reticulum stress in liver disease. Expert Rev Gastroenterol Hepatol. 10:1041–52. DOI: 10.1080/17474124.2016.1179575. PMID: 27093595. PMID: https://www.scopus.com/inward/record.uri?partnerID=HzOxMe3b&scp=84965050646&origin=inward.


26. Zhan X, Qian B, Cao F, Wu W, Yang L, Guan Q, et al. 2015; An Arabidopsis PWI and RRM motif-containing protein is critical for pre-mRNA splicing and ABA responses. Nat Commun. 6:8139. DOI: 10.1038/ncomms9139. PMID: 26404089. PMCID: PMC5514415. PMID: https://www.scopus.com/inward/record.uri?partnerID=HzOxMe3b&scp=84942739586&origin=inward.


27. Yamaguchi Y, Oh-Hashi K, Matsuoka Y, Takemura H, Yamakita S, Matsuda M, et al. 2018; Endoplasmic reticulum stress in the dorsal root ganglion contributes to the development of pain hypersensitivity after nerve injury. Neuroscience. 394:288–99. DOI: 10.1016/j.neuroscience.2018.08.005. PMID: 30482273. PMID: https://www.scopus.com/inward/record.uri?partnerID=HzOxMe3b&scp=85054067852&origin=inward.


28. Inceoglu B, Bettaieb A, Trindade da Silva CA, Lee KS, Haj FG, Hammock BD. 2015; Endoplasmic reticulum stress in the peripheral nervous system is a significant driver of neuropathic pain. Proc Natl Acad Sci U S A. 112:9082–7. DOI: 10.1073/pnas.1510137112. PMID: 26150506. PMCID: PMC4517273. PMID: https://www.scopus.com/inward/record.uri?partnerID=HzOxMe3b&scp=84937711018&origin=inward.


29. Van Opdenbosch N, Lamkanfi M. 2019; Caspases in cell death, inflammation, and disease. Immunity. 50:1352–64. DOI: 10.1016/j.immuni.2019.05.020. PMID: 31216460. PMCID: PMC6611727. PMID: https://www.scopus.com/inward/record.uri?partnerID=HzOxMe3b&scp=85066798798&origin=inward.


30. Jia ZL, Cen J, Wang JB, Zhang F, Xia Q, Wang X, et al. 2019; Mechanism of isoniazid-induced hepatotoxicity in zebrafish larvae: activation of ROS-mediated ERS, apoptosis and the Nrf2 pathway. Chemosphere. 227:541–50. DOI: 10.1016/j.chemosphere.2019.04.026. PMID: 31004821. PMID: https://www.scopus.com/inward/record.uri?partnerID=HzOxMe3b&scp=85064457337&origin=inward.


Fig. 1
Changes in the mechanical threshold. Compared with the sham group, the CCI-ION group showed a decreased mechanical threshold at 3, 6, 10, and 17 days after nerve ligation (**P < 0.001). Compared with the baseline level, the CCI-ION group showed a decreased mechanical threshold at 3, 6, 10, and 17 days after nerve ligation (##P < 0.001). Compared with the baseline level, the SHED group showed increased mechanical threshold at 6, 10, and 17 days after nerve ligation (#P < 0.001). Compared with the medium group, the SHED group showed an increased mechanical threshold at 6, 10, and 17 days after nerve ligation (*P < 0.001). Mechanical threshold data were analyzed using two-way repeated measures ANOVA followed by Bonferroni’s post hoc test. The significance level was determined at P < 0.05. The error bars indicate mean ± standard deviation. CCI-ION: chronic constriction injury of the infraorbital nerve, SHED: stem cells from human exfoliated deciduous teeth.
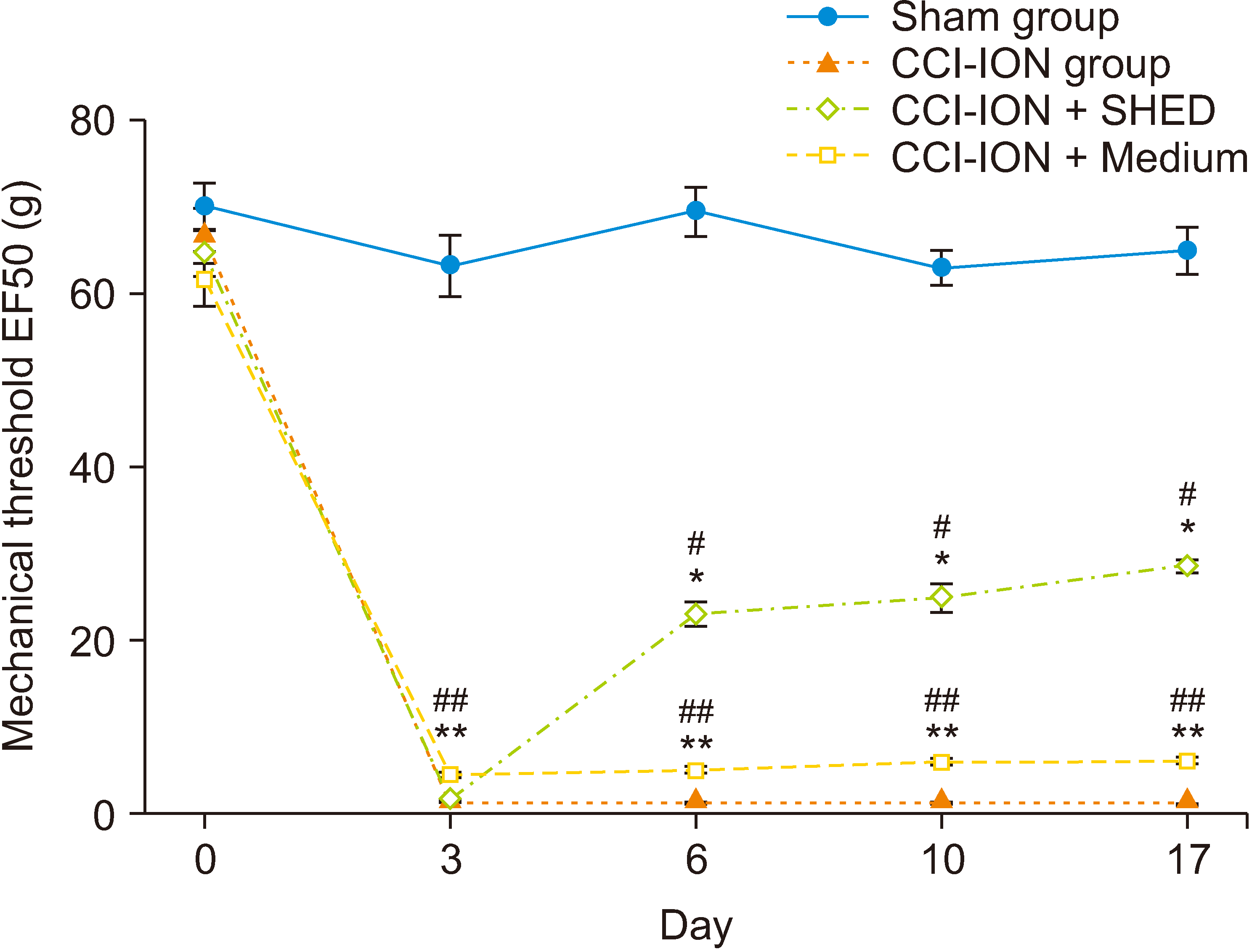
Fig. 2
Change of the shape of the endoplasmic reticulum after nerve ligation. (A) The arrow shows the normal form of the endoplasmic reticulum. (B) The star shows the abnormal form of the endoplasmic reticulum. Scale bar = 1 μm. N: nucleus.
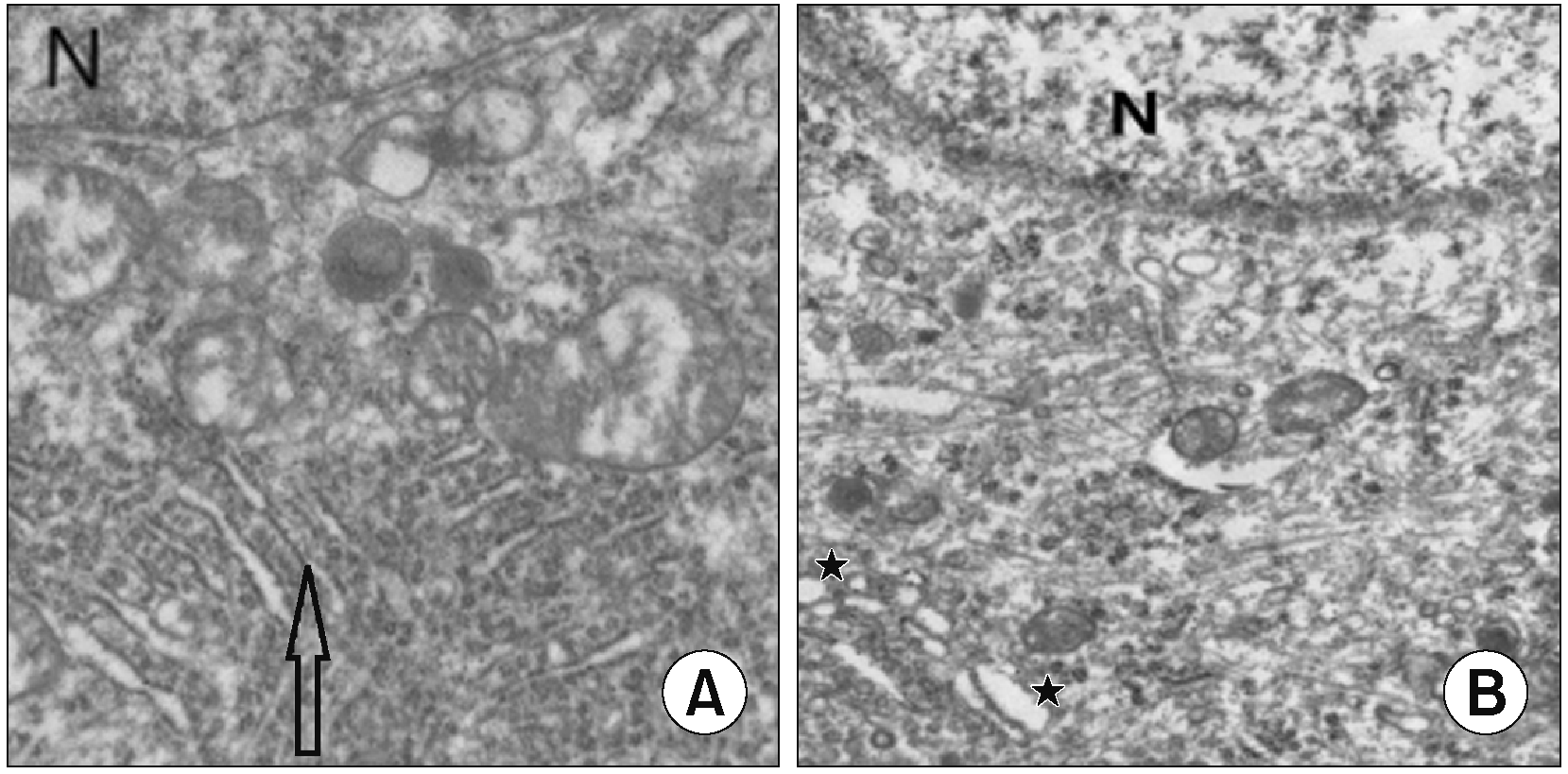
Fig. 3
Relative mRNA and protein levels of BiP induced by TM after SHED treatment in PC12 cells. (A) The TM group compared with the control group (*P = 0.033). The SHED group compared with the TM group (#P = 0.013). (B) The TM group compared with the control group (*P = 0.012).The SHED group compared with the TM group (#P = 0.006). One-way ANOVA was used to compare data in the three groups. The significance level was determined at P < 0.05. The error bars indicate mean ± standard deviation. TM: tunicamycin, SHED: stem cells from human exfoliated deciduous teeth.
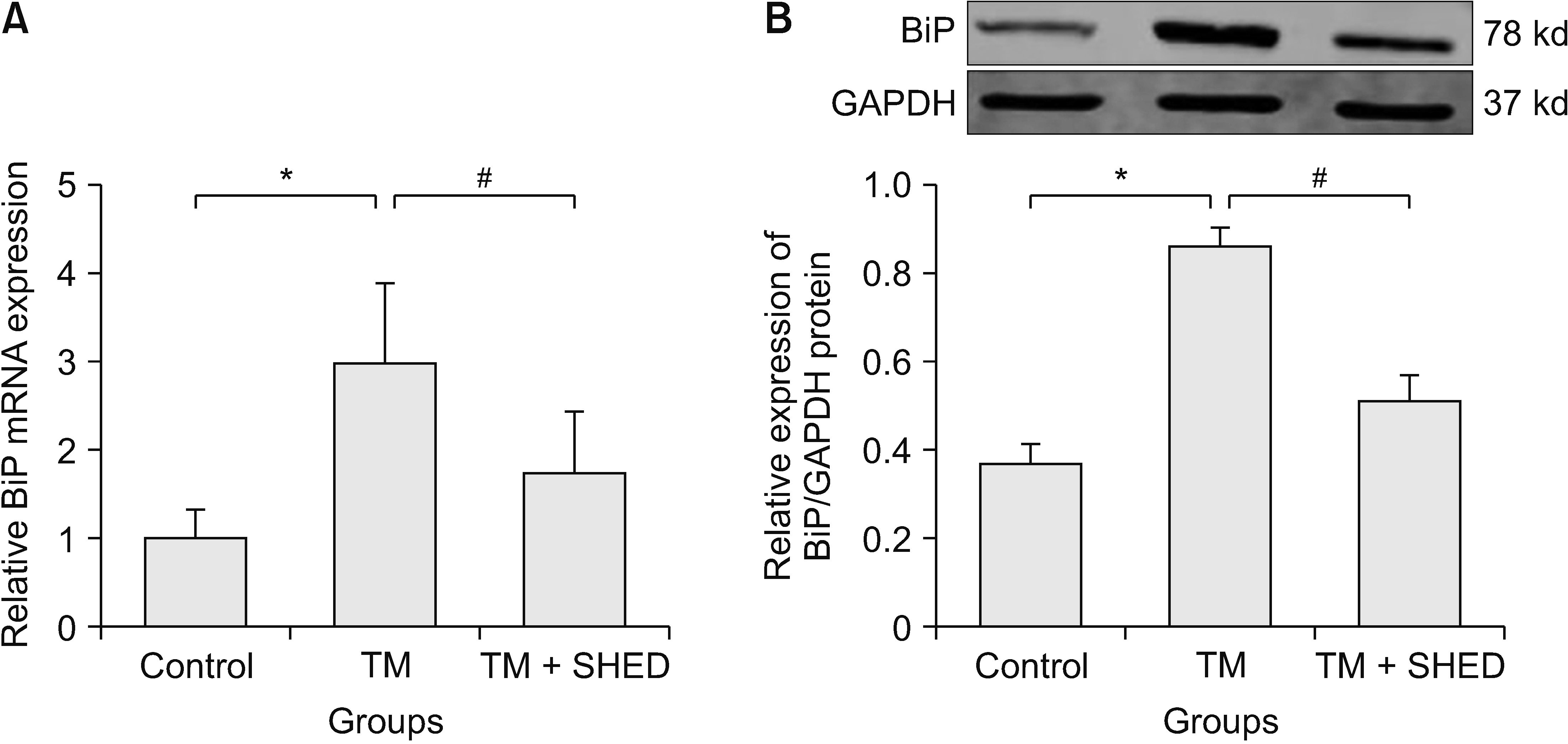
Fig. 4
Relative mRNA and protein levels of Caspase12 from CCI-ION rats after SHED transplantation. (A) The CCI-ION group compared with the sham group (*P = 0.006). The SHED group compared with the CCI-ION group (n = 6 rats in each group) (#P = 0.001). (B) The CCI-ION group compared with the sham group (*P = 0.005). The SHED group compared with the CCI-ION group (n = 6 rats each group) (#P = 0.009). One-way ANOVA was used to compare data in the three groups. The significance level was determined at P < 0.05. The error bars indicate mean ± standard deviation. CCI-ION: chronic constriction injury of the infraorbital nerve, SHED: stem cells from human exfoliated deciduous teeth.
