Abstract
Anterior cruciate ligament is a commonly damaged ligament of the knee. Reconstruction of this ligament usually entails the use of graft harvested from the same subject (i.e., autografts). Several tendons, for example quadriceps, patellar or semitendinosus tendon can be used as an autograft. The composition of the tendons is similar to the anterior cruciate ligament but there is no data that directly compares the compositions of the quadriceps, patellar and semitendinosus tendons. This study quantified and compared the tenocyte distribution and collagen content of these tendons from cadavers of South Africans of European Ancestry. The tenocyte distribution and collagen content were assessed using the ImageJ software. The results showed similarities in the collagen content across the tendons in both sexes (P>0.05). The tenocyte distribution was significantly higher in the quadriceps (P=0.019) or semitendinosus (P=0.016) tendon than in the patellar tendon in the female but no difference was seen in the male (P=0.872). This shows that a large harvestable area may not be directly associated with a more abundant collagen content or tenocyte distribution in the tendon. However, sex-specific tenocyte distribution is an important observation that underpins the possible influence of underlying biological factors on the composition of each tendon and this requires further investigations. In all, this study will contribute to knowledge and assist orthopaedic surgeons in making an informed decision on the choice of graft.
The knee joint is the largest and most complex synovial joint in the human body. Despite the complexity of the knee joint, it is stabilized by ligaments and tendons surrounding the joint. The anterior cruciate ligament (ACL) and the posterior cruciate ligament are important and strong intra-articular ligaments of the knee that contribute to the knee stability by preventing undesirable displacements of the tibia on the femur [1-4]. The ACL fibres are arranged in unique patterns to become taut during flexion or extension thus preventing anterior displacements of the tibia, knee hyperextension [2, 3] and resisting secondary valgus and varus forces [5]. With the ACL lying outside the articular cavity but enclosed within the fibrous membrane, the ACL is prone to injuries during rotational movement of the knee [6, 7] and may tear during a non-contact deceleration or a physical impact in some contact sports e.g. rugby and football [8].
An injured or torn ACL requires a surgical repair [9]. The goal of achieving and maintaining a long-term knee health and stability after an injury is realisable due to ACL reconstruction using autografts [4] which are harvested from the same individual to reconstruct the damaged or torn ligament. This is possible because of similarities in properties (e.g. size and strength) between graft and ACL [10]. The commonest tendons used as autograft are the quadriceps tendon (QT), the patellar tendon (PT), and the semitendinosus tendon (ST) [11, 12]. Despite the popularity of autografts, the choice of graft remains a preference of surgeons [13] as there is no structured ‘scale’ that speaks to the suitability of a graft source in an individual in order to guide the choice of graft [14, 15]. As it stands, many surgeons still rely on their surgical training and the success rates in their practices when choosing a graft [12]. It is quite challenging to reach a consensus on the best graft because factors such as length, harvestable tendon area, thickness, tensile strength, ease of graft harvesting and healing are important factors to consider on a graft choice but unfortunately all of these factors are not obtainable in a single tendon [16]. Other factors of importance are rapid healing, low donor site morbidity, immediate rigid fixation and similar structural properties that mimic the mechanical properties of ACL [16, 17].
The present study investigated the microstructure of the commonly used autografts (e.g., QT, PT, and ST) for ACL reconstruction by quantifying and comparing the tenocyte distribution and collagen content in these tendons. This study hypothesized that the microstructure of the tendons could shed more light into the usability of each graft with respect to healing at the donor site and knee recovery in individuals. Understanding this will contribute to knowledge and assist surgeons in their choice of graft.
Tissue samples of the QT, PT, and ST were only collected from specific cadavers (i.e. from scatter remains according to the Body Donation Policy of the School of Anatomical Sciences, University of the Witwatersrand). This study was covered by the Human Research Ethics Committee (Medical) Committee of the University of the Witwatersrand (Ethics Waiver Number: W-CJ-140604-1). Tissue samples of the QT, PT, and ST of the right leg were collected from female (n=9; age range, 72–93 years) and male (n=7; age range, 59–85 years) cadavers of South Africans of European Ancestry. With the cadaver in a supine position and the knee flexed at an angle of about 45°, the tendons of interest were exposed by carefully reflecting the skin, subcutaneous fascia, fat, fascia lata, and crural fascia overlying the tendons. Tissue block of the QT or PT (1 cm×0.5 cm) was removed from the central half of the tendon (Fig. 1A, B) and then tissue block of about 1 cm long was cut half-way along the ST tendon (Fig. 1C) but with the cadaver in a prone position and the lower limb in full extension. All tissue blocks were immediately placed in a 10% buffered formalin and stored at 4°C until further histological processing.
Each tissue block was prepared and sectioned longitudinally at 9 μm thickness using a sliding microtome (Leica, Wetzlar, Germany). A one in four serial section was used for each stain and then mounted on to a 0.5% gelatine-coated slide. The slides with the sections were kept at room temperature to dry overnight before staining with either H&E to reveal the general microstructure and to quantify the distribution of tenocytes or Masson’s Trichrome (MT) to reveal collagen fibers and to quantify its distribution.
Photomicrographs of each section were taken at every 2-mm interval along the length of the section using a digital camera attached to a Zeiss Axioscope microscope (Zeiss, Oberkochen, Germany) at times 63 objective lens. All images were acquired under similar settings on the microscope. The photomicrograph was then fed into the ImageJ software where a 6-frame counting grid (220 μm2) was superimposed on the image for ease of counting. The number of tenocytes in the six frames were then counted. The tenocyte distribution per image was calculated by dividing the total number of tenocytes in the 6-frame grid by the area of the 6-frame grid.
Photomicrographs of MT-stained sections were captured using a digital camera attached to a Zeiss Axioscope microscope at times 10 objective lens. For each section, photomicrographs were taken at every 3-mm interval along the length of the section. All images were also acquired under similar settings on the microscope. All acquired digitized images were stored in a jpeg file format with 24-bit RGB according to the color deconvolution plugin of the ImageJ software. The method using the ImageJ software as described by Chen et al. [18] was used to quantify the collagen fibers. With the scale bar set on the software, colors on the image were separated from overlapping regions using the color deconvolution plugin of the software. This thus deconvolved the image into red, blue and green i.e. RGB components using the orthonormal transformation of the RGB information of the image. In this case, the green component represents the collagen fibers [18]. A threshold was then set by manually adjusting the entire green-colored component (i.e. the collagen fibers) until they were highlighted in red following which the highlighted area per image was then analyzed. The collagen distribution (%) for each image was calculated by dividing the area covered by collagen by the size (area) of the image (65,450 μm2).
The data obtained were not normally distributed (i.e. non-parametric) according to the Shapiro–Wilk test. A Kruskal–Wallis test was used to assess differences in the distribution of tenocytes or collagen distribution across the tendons (i.e., QT, PT, and ST). In addition, sex difference in each tendon was conducted using a Mann–Whitney test. All statistical analyses were performed using the SPSS software (version 22.0; IBM Corp., Armonk, NY, USA). Statistical difference of 5% was regarded as significant for all the statistical analyses.
At the microscopic level, the three tendons have similar micro-architectural arrangements (Fig. 2). Fascicles made up of aggregates of collagen molecules were organised side-by-side and end-to-end along the tendon as seen in the H&E or MT stains. Several fascicles aggregate to then form the tendon fibres. The tenocytes appeared blue-stained in the H&E (Fig. 2A–C). The tenocytes were mostly spindle or flat in shape and they were sparsely distributed along the fascicles in the form of longitudinal arrays. Numerous collagen deposits appeared green stained in the MT staining (Fig. 2D–F). The collagen distribution was conspicuous, and their distribution seemed widely distributed in all the tendons assessed except in some areas where collagen distribution was more dense.
The descriptive analyses of the tenocyte distribution per tendon for both sexes are shown in Table 1. Boxplots showing the characteristics of the data (i.e. the quartile ranges and median) for both sexes are shown in Fig. 3. In the female, the tenocyte distribution was significantly different across the tendons (P=0.006). PT had the lowest tenocyte distribution and post hoc analyses using a Dunn’s test showed that the tenocyte distribution in the PT was significantly lower than the QT (P=0.019) or the ST (P=0.016). However, the tenocyte distribution was not significantly different across the tendons in the male (P=0.872). For each tendon, there was no sex difference in the tenocyte distribution (P>0.05).
The average percentage of collagen distribution per tendon in the female and the male cadavers is shown in Table 2. A boxplot showing the characteristics of the data in both sexes is shown in Fig. 4. Despite the ST having the lowest percentage of collagen distribution in both sexes, a Kruskal–Wallis one-way analysis of variance showed that the percentage of collagen distribution was not significantly different across the tendons in the female (P=0.383) or in the male (P=0.567). In addition, a Mann–Whitney test showed no sex difference in the collagen distribution in each tendon (P>0.05).
The microstructure and composition of harvestable tissues are important in order to determine their suitability as a graft. To further provide additional information that will be useful to surgeons on their choice of graft, the present study compared the tenocyte distribution and the collagen content in the QT, PT, and ST. The tenocytes and collagen contribute to the strength of tendons which are essential for the success of an ACL reconstruction. From the present observation, the microstructure of the QT, PT, and ST was similar. They were generally composed of closely packed collagen fibres with tenocytes interspersed within the collagen bundles [19, 20].
Tendon strength is attributed to collagen content and the QT has an advantage over the PT because it has a more (approximately 20%) collagen content [20] which enables the QT to endure a higher load to failure and strength than the PT [21, 22]. Considering the surface areas of the QT and the PT, the implication of this is that the remaining collagen content after the harvesting of a graft should be sufficient for the QT to function more satisfactorily than the PT with a lesser collagen content due to its surface area. This is evident by reports that showed that the QT produces a better functional and clinical outcome with no serious donor site morbidity than the PT [20, 23]. To reiterate, the QT is also considered a biomechanically efficient alternative for ACL reconstruction that is considered safe and reproducible with an abundant harvestable tissue [21, 24-27]. Unfortunately, the present study did not find a significant difference in the collagen distributions of the tendons despite the ST having the lowest collagen distribution in both sexes.
Tenocytes facilitate the healing of tendon tissue and the regeneration of tissue after harvesting [19]. Similar to collagen, QT is assumed to produce a better healing than the PT or ST due to its large surface area and the abundance of tenocytes to initiate a more rapid healing at the donor site and the production of more collagen to sustain the remaining tendon after harvest. Thus, the cell activities should promote a faster return of knee functions when a QT was harvested compared to the PT or ST. The findings of the present study do not support this assumption as there was no significant difference in the tenocyte distribution across the tendons in the male population assessed. However, this assumption is in alignment with the findings in the female population that showed that tenocyte distribution was significantly higher in the QT or in the ST than in the PT. This is also consistent with Hadjicostas et al. [19, 20] that reported a significantly higher tenocyte distribution in the ST than in the PT [19] and also a significantly higher tenocyte distribution in the QT than in the PT [20].
Interestingly, the whole of the ST can regenerate after being harvested [28-30] where post-harvest hematoma may act as a scaffold for the mesenchymal stem cells to invade the harvest site and then initiate tenocyte proliferation and collagen production [30]. In most cases, the ST regenerates to its full length with a microstructure similar to the non-harvested tendon [29]. However, this phenomenon of ST remodelling and regeneration is not seen in all patients [31] and could be as a result of added strain on the hamstring muscle as patients that experienced unsuccessful remodelling reported experiencing a sudden sharp/stabbing pain in the posterior aspect of the thighs [31]. To prevent the onset of this problem, hamstring strengthening exercises in the first month post-surgery is not recommended and should be completely avoided in order to increase the chances of tissue regeneration and remodelling [31]. This ability of the ST to regenerate can be likened to the findings of the present study in that the high distribution of tenocytes in the ST in the female may be associated with its ability to regenerate as it is a known fact that tenocytes are important for tendon regeneration and healing however tenocyte proliferation is controlled by growth factors e.g. platelet-derived growth factor receptor or cytokines [19, 20]. At the same time, the interactions between the biological and growth factors cannot be overlooked where sex hormones may act as a regulator of growth factors [32]. The present study thus suggests that there may be an influence of underlying biological factors that may be responsible for the unique observation seen in the female with respect to the tenocyte distribution in the ST.
The reason for the female-specific tenocyte distribution patterns in the present study remains unknown but it highlights the possible impact of biological differences (e.g. hormones) on the healing process and the return of knee functions. Interestingly, hormone imbalance or surges during menstrual cycle, ovulatory phase or pregnancy cause laxity of the ACL ligaments and knee stiffness [6, 33-35]. Likewise improved knee function has been reported to be better in the male than in the female [36]. More so, females generally have a hypermobile and highly flexible joints than males [33]. Thus factors such as anatomic, mechanical, hormonal and genetic factors are important factors for increased risk of ACL injuries in female compared to male [37] and which are also suggested to play an important role in the healing process and return of knee function at both the harvest site and ACL-reconstructed knee. Further investigations are needed to ascertain the roles of these factors. In addition, the collagen distributions across the tendons were similar despite the large surface area of the QT (unpublished report). It would also be interesting to investigate changes in the collagen content at the harvest sites of commonly used ACL autografts in different sexes as this will shed more light into the tendon healing processes and may further reveal the possible impact of biological factors on tendon healing.
In conclusion, the QT provides an abundant harvestable tissue which is superior to either the PT or ST but its large harvestable area may not be directly associated with a more abundant collagen content or tenocyte distribution. The QT and ST further confirm their suitability for use as autografts for ACL reconstruction based on their significantly high tenocyte distribution but why this observation was female-specific remains unknown. Thus, sex-specific differences in the tenocyte distribution is an important observation that underpins the possible influence of underlying biological factors on the composition of each tendon and which needs to be investigated. This study provides additional cues on the differences in composition of commonly used autografts and highlights the possible role of biological factors on tendon composition. In all, this study will contribute to knowledge and assist orthopaedic surgeons in making an informed decision on the choice of graft.
Acknowledgements
The authors sincerely thank those who donated their bodies to science so that anatomical research could be performed. Results from such research can potentially increase mankind's overall knowledge that can then improve patient care. Therefore, these donors and their families deserve our highest gratitude. We are grateful to the School of Anatomical Sciences of the University of the Witwatersrand for giving access to the Human Collections and to Mrs. H. Ali for assistance with histology.
Notes
References
1. Petersen W, Tillmann B. 2002; Anatomy and function of the anterior cruciate ligament. Orthopade. 31:710–8. German. DOI: 10.1007/s00132-002-0330-0. PMID: 12426749.
2. Zhu J, Zhang X, Ma Y, Zhou C, Ao Y. 2012; Ultrastructural and morphological characteristics of human anterior cruciate ligament and hamstring tendons. Anat Rec (Hoboken). 295:1430–6. DOI: 10.1002/ar.22527. PMID: 22807249.


3. Cerulli G, Placella G, Sebastiani E, Tei MM, Speziali A, Manfreda F. 2013; ACL reconstruction: choosing the graft. Joints. 1:18–24. PMID: 25606507. PMCID: PMC4295687.
4. Lalwani R, ivastava R Sr, Kotgirwar S, Athavale SA. 2020; New insights in anterior cruciate ligament morphology: implications for anterior cruciate ligament reconstruction surgeries. Anat Cell Biol. 53:398–404. DOI: 10.5115/acb.20.119. PMID: 33012726. PMCID: PMC7769096.


5. Quatman CE, Hewett TE. 2009; The anterior cruciate ligament injury controversy: is "valgus collapse" a sex-specific mechanism? Br J Sports Med. 43:328–35. DOI: 10.1136/bjsm.2009.059139. PMID: 19372087. PMCID: PMC4003572.


6. Shultz SJ. 2015; ACL injury risk in the physically active: why are females more susceptible? Kinesiol Rev. 4:52–62. DOI: 10.1123/kr.2014-0076.


7. van Zyl R, van Schoor AN, du Toit PJ, Louw EM. 2016; Clinical anatomy of the anterior cruciate ligament and pre-operative prediction of ligament length. SA Orthop J. 15:47–52. DOI: 10.17159/2309-8309/2016/v15n4a7.


8. Hurley ET, Calvo-Gurry M, Withers D, Farrington SK, Moran R, Moran CJ. 2018; Quadriceps tendon autograft in anterior cruciate ligament reconstruction: a systematic review. Arthroscopy. 34:1690–8. DOI: 10.1016/j.arthro.2018.01.046. PMID: 29628380.


9. Sayampanathan AA, Howe BK, Bin Abd Razak HR, Chi CH, Tan AH. 2017; Epidemiology of surgically managed anterior cruciate ligament ruptures in a sports surgery practice. J Orthop Surg (Hong Kong). 25:2309499016684289. DOI: 10.1177/2309499016684289. PMID: 28142351.


10. Shelton WR, Fagan BC. 2011; Autografts commonly used in anterior cruciate ligament reconstruction. J Am Acad Orthop Surg. 19:259–64. DOI: 10.5435/00124635-201105000-00003. PMID: 21536625.


11. Frank R, Higgins J, Bernardoni E, Cvetanovich G, Bush-Joseph CA, Verma NN, Bach BR Jr. 2017; Anterior cruciate ligament reconstruction basics: bone-patellar tendon-bone autograft harvest. Arthrosc Tech. 6:e1189–94. DOI: 10.1016/j.eats.2017.04.006. PMID: 29354416. PMCID: PMC5621981.


12. Mall NA, Matava MJ, Wright RW, Brophy RH. 2012; Relation between anterior cruciate ligament graft obliquity and knee laxity in elite athletes at the National Football League combine. Arthroscopy. 28:1104–13. DOI: 10.1016/j.arthro.2011.12.018. PMID: 22421564.


13. Macaulay AA, Perfetti DC, Levine WN. 2012; Anterior cruciate ligament graft choices. Sports Health. 4:63–8. DOI: 10.1177/1941738111409890. PMID: 23016071. PMCID: PMC3435898.


14. Romanini E, D'Angelo F, De Masi S, Adriani E, Magaletti M, Lacorte E, Laricchiuta P, Sagliocca L, Morciano C, Mele A. 2010; Graft selection in arthroscopic anterior cruciate ligament reconstruction. J Orthop Traumatol. 11:211–9. DOI: 10.1007/s10195-010-0124-9. PMID: 21181226. PMCID: PMC3014473.


15. Sun J, Wei XC, Li L, Cao XM, Li K, Guo L, Lu JG, Duan ZQ, Xiang C, Wei L. 2020; Autografts vs synthetics for cruciate ligament reconstruction: a systematic review and meta-analysis. Orthop Surg. 12:378–87. DOI: 10.1111/os.12662. PMID: 32180364. PMCID: PMC7189042. PMID: 66884f9852f1451ba8de4b08e150d934.


16. Hijazi MM, Khan MA, Altaf FMN, Ahmed MR, Alkhushi AG, Sakran AMEA. 2015; Quadriceps tendon and patellar ligament; a morphometric study. Prof Med J. 22:1192–5. DOI: 10.29309/TPMJ/2015.22.09.1135.


17. Goldblatt J, Fitzsimmons SE, Balk E, Richmond JC. 2005; Reconstruction of the anterior cruciate ligament: meta-analysis of patellar tendon versus hamstring tendon autograft. Arthroscopy. 21:791–803. DOI: 10.1016/j.arthro.2005.04.107. PMID: 16012491.


18. Chen Y, Yu Q, Xu CB. 2017; A convenient method for quantifying collagen fibers in atherosclerotic lesions by ImageJ software. Int J Clin Exp Med. 10:14904–10.
19. Hadjicostas P, Soucacos PN, Paessler HH, Koleganova N, Berger I. 2007; Morphologic and histologic comparison between the patella and hamstring tendons grafts: a descriptive and anatomic study. Arthroscopy. 23:751–6. DOI: 10.1016/j.arthro.2007.02.002. PMID: 17637411.


20. Hadjicostas PT, Soucacos PN, Berger I, Koleganova N, Paessler HH. 2007; Comparative analysis of the morphologic structure of quadriceps and patellar tendon: a descriptive laboratory study. Arthroscopy. 23:744–50. DOI: 10.1016/j.arthro.2007.01.032. PMID: 17637410.


21. Shani R, Umpierez E, Nasert M, Hiza EA, Xerogeanes J. 2016; Biomechanical comparison of quadriceps and patellar tendon grafts in anterior cruciate ligament reconstruction. Arthroscopy. 32:71–5. DOI: 10.1016/j.arthro.2015.06.051. PMID: 26382635.


22. Krebs N, Yaish A, O'Neill N. 2019; Anatomic evaluation of the quadriceps tendon in cadaveric specimens: application for anterior cruciate ligament reconstruction graft choice. Spartan Med Res J. 4:7961. DOI: 10.51894/001c.7961. PMID: 33655157. PMCID: PMC7746052.


23. Cavaignac E, Pailhé R, Murgier J, Reina N, Lauwers F, Chiron P. 2014; Can the gracilis be used to replace the anterior cruciate ligament in the knee? A cadaver study. Knee. 21:1014–7. DOI: 10.1016/j.knee.2014.07.010. PMID: 25108844.


24. Stäubli HU, Schatzmann L, Brunner P, Rincón L, Nolte LP. 1999; Mechanical tensile properties of the quadriceps tendon and patellar ligament in young adults. Am J Sports Med. 27:27–34. DOI: 10.1177/03635465990270011301. PMID: 9934415.


25. DeAngelis JP, Fulkerson JP. 2007; Quadriceps tendon--a reliable alternative for reconstruction of the anterior cruciate ligament. Clin Sports Med. 26:587–96. DOI: 10.1016/j.csm.2007.06.005. PMID: 17920954.


26. Geib TM, Shelton WR, Phelps RA, Clark L. 2009; Anterior cruciate ligament reconstruction using quadriceps tendon autograft: intermediate-term outcome. Arthroscopy. 25:1408–14. DOI: 10.1016/j.arthro.2009.06.004. PMID: 19962067.


27. Slone H, Romine SE, Premkumar A, Xerogeanes JW. 2015; Quadriceps tendon autograft for anterior cruciate ligament reconstruction: a comprehensive review of current literature and systematic review of clinical results. Arthroscopy. 31:541–54. DOI: 10.1016/j.arthro.2014.11.010. PMID: 25543249.


28. Eriksson K, Kindblom LG, Hamberg P, Larsson H, Wredmark T. 2001; The semitendinosus tendon regenerates after resection: a morphologic and MRI analysis in 6 patients after resection for anterior cruciate ligament reconstruction. Acta Orthop Scand. 72:379–84. DOI: 10.1080/000164701753542041. PMID: 11580127.


29. Stevanović V, Blagojević Z, Petković A, Glišić M, Sopta J, Nikolić V, Milisavljević M. 2013; Semitendinosus tendon regeneration after anterior cruciate ligament reconstruction: can we use it twice? Int Orthop. 37:2475–81. DOI: 10.1007/s00264-013-2034-y. PMID: 23982635. PMCID: PMC3843191.


30. Suydam SM, Cortes DH, Axe MJ, Snyder-Mackler L, Buchanan TS. 2017; Semitendinosus tendon for ACL reconstruction: regrowth and mechanical property recovery. Orthop J Sports Med. 5:2325967117712944. DOI: 10.1177/2325967117712944. PMID: 28680900. PMCID: PMC5490845.


31. Nakamae A, Ochi M, Deie M, Adachi N. 2012; Unsuccessful regeneration of the semitendinosus tendon harvested for anterior cruciate ligament reconstruction: report of two cases. Orthop Traumatol Surg Res. 98:932–5. DOI: 10.1016/j.otsr.2012.07.011. PMID: 23123037.


32. Spelsberg TC, Subramaniam M, Riggs BL, Khosla S. 1999; The actions and interactions of sex steroids and growth factors/cytokines on the skeleton. Mol Endocrinol. 13:819–28. DOI: 10.1210/mend.13.6.0299. PMID: 10379881.


33. Harmon KG, Ireland ML. 2000; Gender differences in noncontact anterior cruciate ligament injuries. Clin Sports Med. 19:287–302. DOI: 10.1016/S0278-5919(05)70204-0. PMID: 10740760.


34. Park SK, Stefanyshyn DJ, Ramage B, Hart DA, Ronsky JL. 2009; Alterations in knee joint laxity during the menstrual cycle in healthy women leads to increases in joint loads during selected athletic movements. Am J Sports Med. 37:1169–77. DOI: 10.1177/0363546508330146. PMID: 19289541.


35. Csintalan RP, Inacio MC, Funahashi TT. 2008; Incidence rate of anterior cruciate ligament reconstructions. Perm J. 12:17–21. DOI: 10.7812/TPP/07-140. PMID: 21331205. PMCID: PMC3037119.


36. Kim DK, Park WH. 2015; Sex differences in knee strength deficit 1 year after anterior cruciate ligament reconstruction. J Phys Ther Sci. 27:3847–9. DOI: 10.1589/jpts.27.3847. PMID: 26834366. PMCID: PMC4713805.


37. Vaudreuil N, Roe J, Salmon L, Servien E, van Eck C. 2020; Management of the female anterior cruciate ligament: current concepts. J ISAKOS. 5:123–7. DOI: 10.1136/jisakos-2019-000332.


Fig. 1
Illustrations of the approximate position where tissue block (dotted lines and drawn to scale for each tendon) was removed from the QT (A), PT (B), or ST (C). Tissue block of 1 cm×0.5 cm was removed at the centre of the QT or PT while 1 cm long tendon was removed at the mid-way of the semitendinosus tendon. QT, quadriceps tendon; P, patella; VM, vastus medialis; VL, vastus lateralis; PT, patellar tendon; ST, semitendinosus tendon; S, semitendinosus.
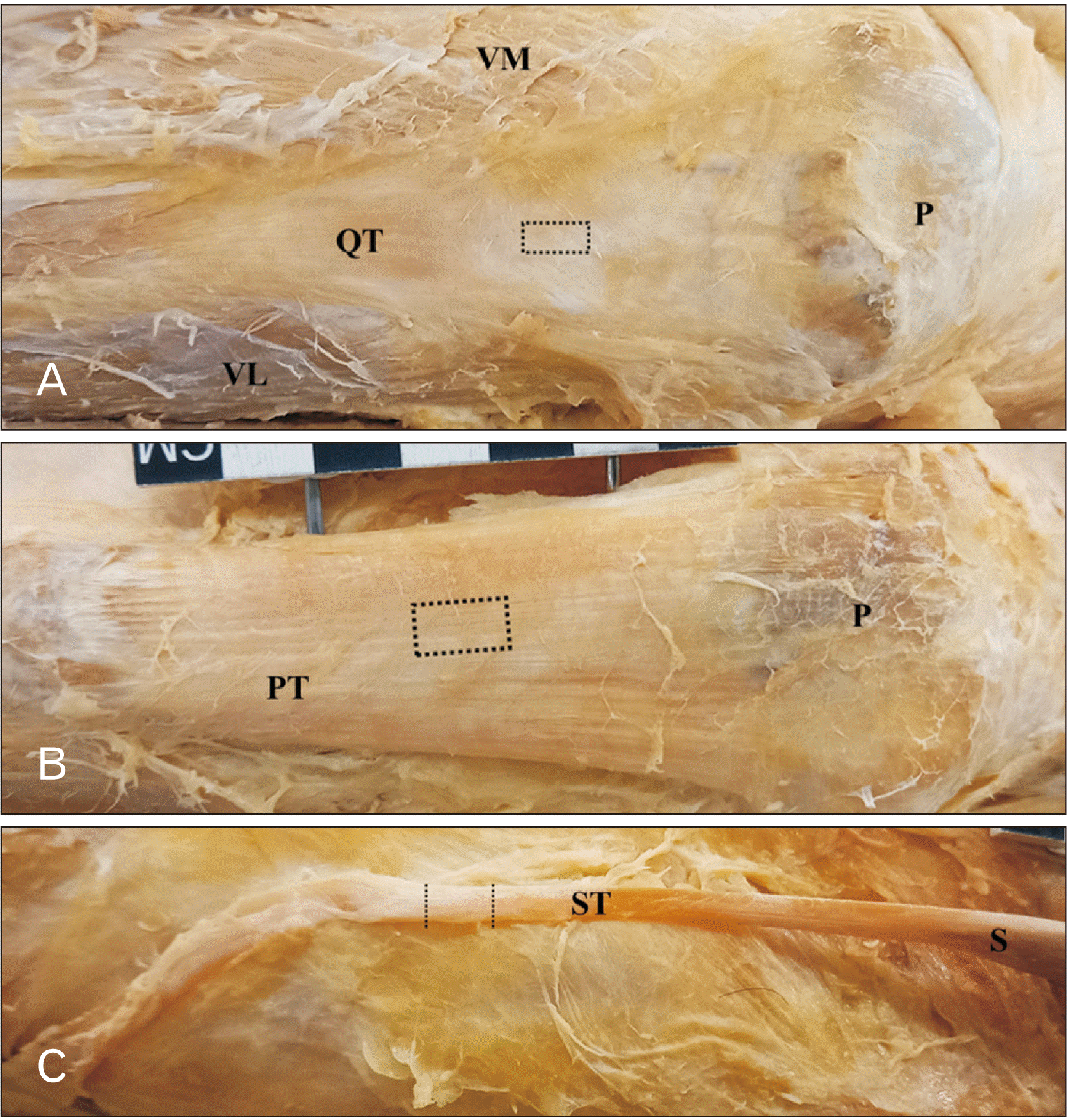
Fig. 2
Photomicrographs showing representative longitudinal sections of the quadriceps tendon (QT) (A, D), the patellar tendon (PT) (B, E), and the semitendinosus tendon (ST) (C, F). The micro-architecture of the three tendons were closely identical with the fascicles arranged in parallel and end-to-end in the longitudinal plane along the length of the tendons. In the H&E staining (A–C) (×63 objective lens), the blue-stained fibroblasts appeared spindle or flat in shape and were uniformly distributed in all the tendons. In the MT staining (D–F) (×10 objective lens), collagen appeared green-stained and appeared to be widely distributed in the fascicles.
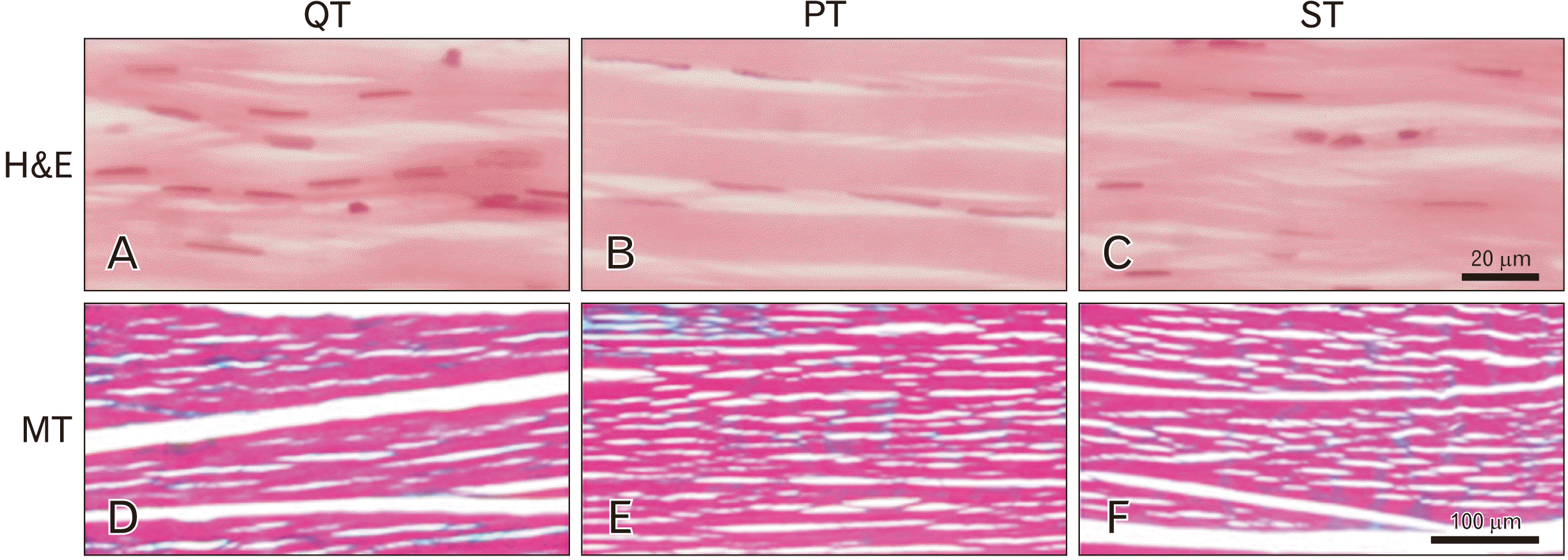
Fig. 3
A box plot showing the comparison of the tenocyte distribution across the tendons (QT, PT, and ST) in the female and the male cadavers. Tenocyte distribution is significantly lower in the PT than in the QT or in the ST in the female cadavers but not in the male cadaver. QT, quadriceps tendon; PT, patellar tendon; ST, semitendinosus tendon. *P<0.05.
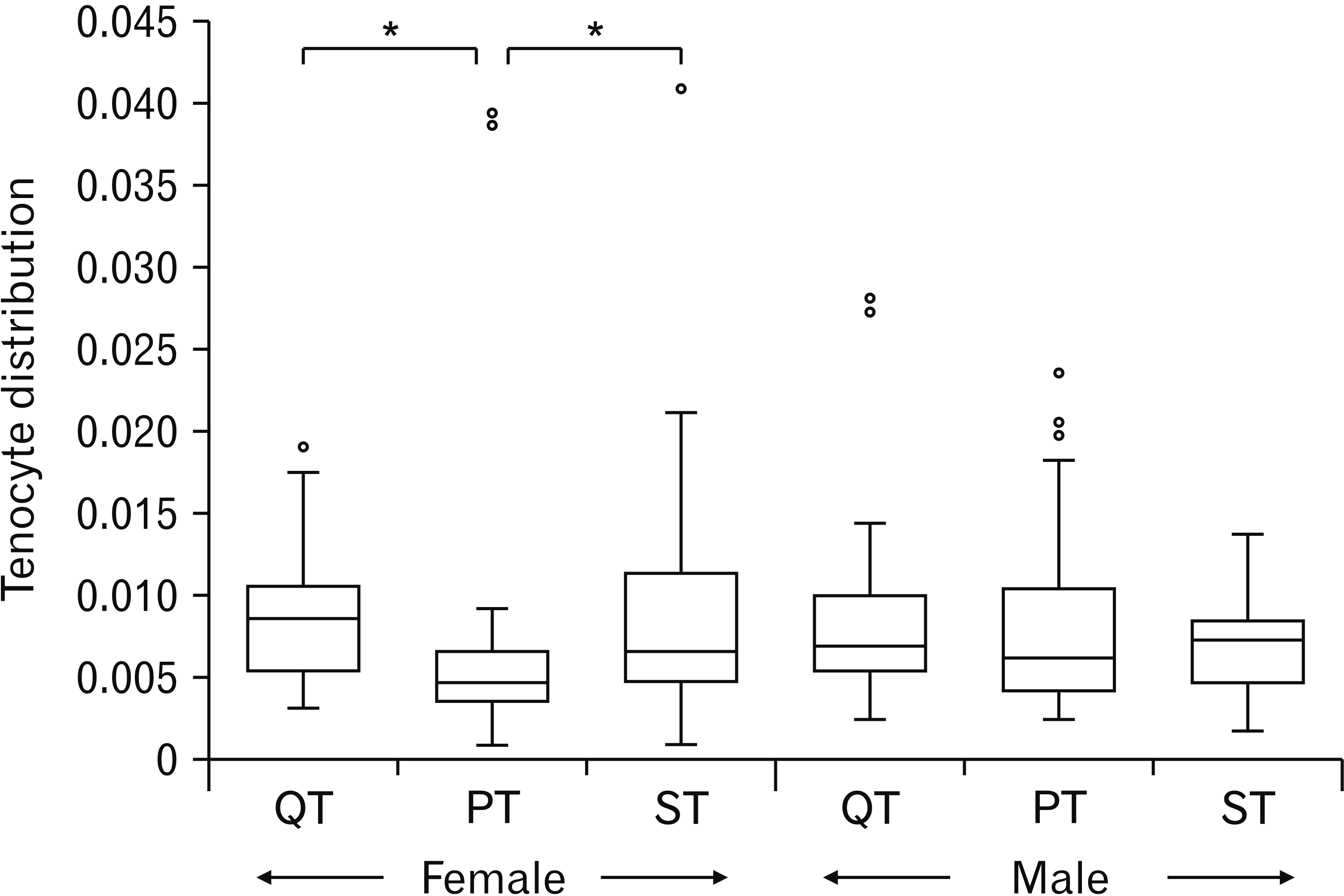
Fig. 4
A box plot showing the comparison of the percentage distribution of collagen across the tendons (QT, PT, and ST) in the female and the male cadavers. Percentage collagen distribution across the tendons is similar for both sexes. QT, quadriceps tendon; PT, patellar tendon; ST, semitendinosus tendon.
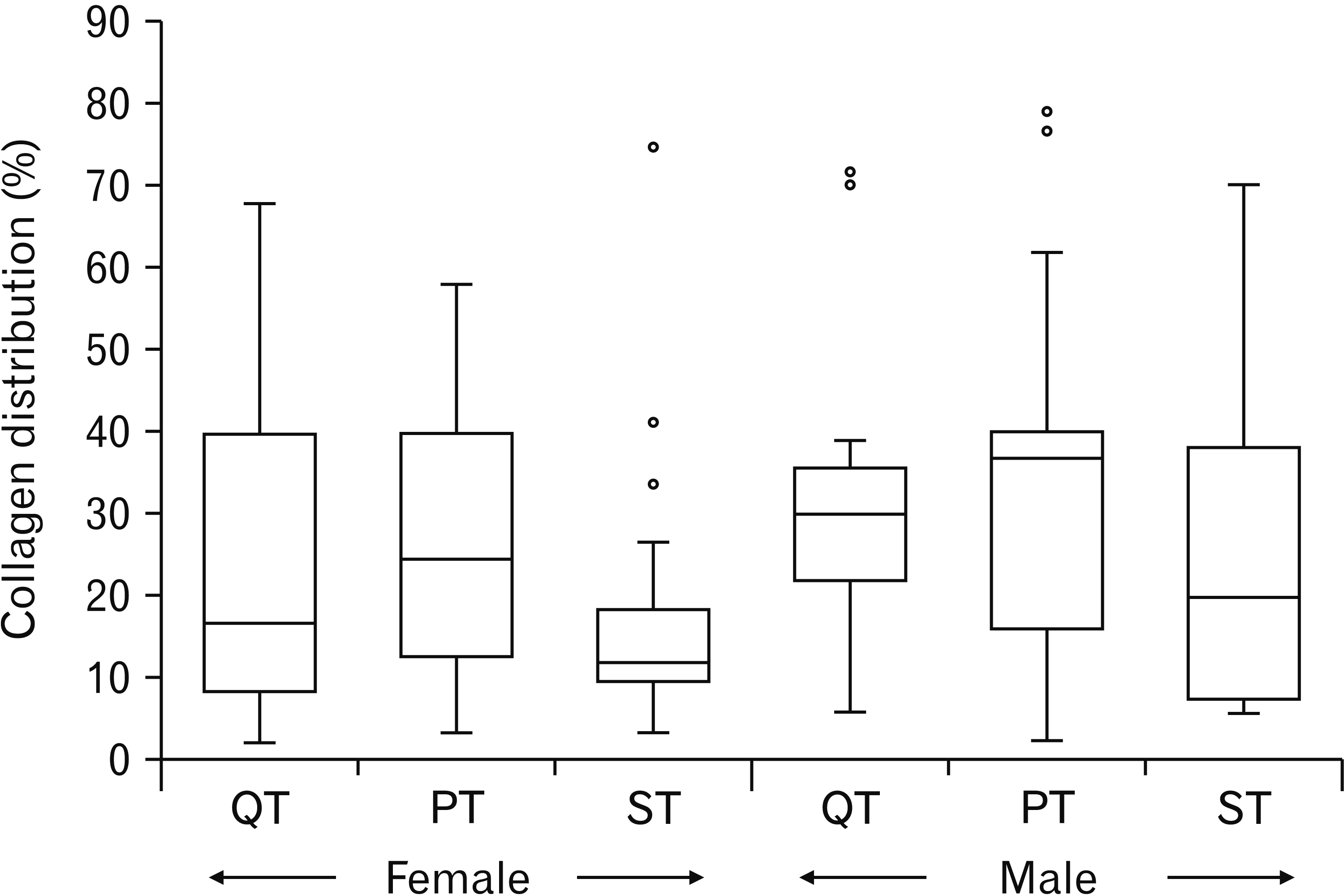
Table 1
Descriptive statistics of tenocyte distribution in the QT, PT, and ST
Sex | QT | PT | ST | P-value | ||||||||
---|---|---|---|---|---|---|---|---|---|---|---|---|
No. of images assessed | Mean (SD) | Median | No. of images assessed | Mean (SD) | Median | No. of images assessed | Mean (SD) | Median | ||||
Female | 42 | 0.008 (0.004) | 0.008 | 31 | 0.007 (0.009) | 0.005 | 42 | 0.009 (0.007) | 0.006 | 0.006* | ||
Male | 29 | 0.008 (0.006) | 0.007 | 34 | 0.008 (0.006) | 0.006 | 30 | 0.007 (0.003) | 0.007 | 0.872 |
Table 2
Descriptive statistics of collagen distribution in the QT, PT, and ST