Genetic mutations that occur in blood cancer can be broadly classified into nucleotide sequence mutations, including duplications or deletions; fusion gene mutations; and abnormal gene overexpression. Among these genetic mutations, gene fusion not only plays an important role in the development of blood cancers such as leukemia, it is also important as an essential marker for diagnosis, risk assessment, optimal treatment selection, and residual lesion detection. Most clinical hematology laboratories use conventional cytogenetic testing, fluorescent in situ hybridization (FISH), and multiplex reverse transcriptase-PCR to detect gene fusion mutations [1, 2]. However, the above methods currently used have great limitations in detecting various gene fusions that occur in blood cancer. To overcome these shortcomings, RNA sequencing (RNA-seq) methods are being introduced in clinical laboratories. However, the RNA-seq method is not widely used due to the complexity of the data processing and interpretation. The authors developed a targeted RNA-seq panel (KBB-RNAseq NGS-Leukemia- PHB; KBlueBio Inc., Hwasun, Korea) and bioinformatics pipeline that can be used for targeted RNA-seq to detect gene fusions, sequence variants, and altered gene expression, and is more convenient than traditional RNA-seq methods for use in clinical hematology laboratories [3]. The targeted RNA-seq panel and analysis pipeline, developed using information technology with an automated reporting system and commercialized by the authors, was subjected to a comparative evaluation with a commercialized targeted RNA- seq panel (ArcherTM FusionPlexTM Heme Panel version 2, Archer DX, Boulder, CA, USA) using leukemia patient samples.
The targeted RNA-seq system for detecting gene fusions in blood cancers developed by the authors comprised 84 genes, including the self-discovered prohibitin as a companion diagnostic marker for hematological malignancies, and was optimized by MiseqDx (Illumina, San Diego, CA, USA) after library preparation using the capture hybridization method. The test performance of this system was evaluated using bone marrow specimens that were collected when diagnosing 93 patients with leukemia [15 with acute myeloid leukemia (AML), 35 with adult B-acute lymphoid leukemia (B-ALL), 30 with childhood B-ALL, and 13 with T-acute lymphoid leukemia (T-ALL)]. Out of all 93 leukemia patients, tier 1 or tier 2 gene fusions were observed in 72 (77%) patients. Fusion gene mutations were detected in 83% (25/30) of the childhood B-ALL and 94% of the adult B-ALL samples (33/35). In patients with AML or T-ALL, fusion gene mutations were found in 53% (8/15) and 46% (6/13), respectively (Fig. 1).
A commercial targeted RNA-seq analysis system developed by constructing a cDNA library for specific genes using anchored multiplex PCR [3] was compared to the detection system (KBB-RNAseq NGS-Leukemia-PHB) developed by the authors [4]. For this comparative evaluation, four cases of B-ALL, two cases of AML, and one case each of acute promyelocytic leukemia and T-ALL were used. The results of the two analysis systems were consistent for seven of the eight comparatively evaluated patients, however, B-ALL accompanied by an IGH-CRLF2 gene fusion was detected only in the panel developed by the authors (Table 1). Philadelphia chromosome (Ph)-ALL is found in 20–25% of B-ALL cases, and it is known that approximately 61% of the fusion gene mutations related to the CRLF2 gene are causative genes. Ph-like B-ALL has a very poor patient prognosis and has been reclassified as a new subtype of ALL [5, 6]. Therefore, the diagnosis of Ph-like B-ALL through the genetic analysis of ALL patients, especially B-ALL patients, is very important for treating leukemia with precision medicine. Our targeted RNA-seq analysis system was found to be more effective at detecting Ph-like B-ALL than the cDNA library-based targeted RNA-seq using anchored multiplex PCR, which is currently commercially available and used in some clinical testing sites [4].
Information technology (IT)-based data analytics software (NGeneAnalySys, NGeneBio, Seoul, Korea) was used to analyze the data produced by the next-generation sequencing (NGS) equipment using the RNA-seq panel (KBB-RNAseq NGS-Leukemia-PHB) developed by the authors (Supplementary Fig. 1). After selecting the data generated by the NGS equipment, the data are uploaded to the analysis server, the panel type is selected, and an automatic analysis is performed. The status of the analysis can be checked in real-time, as can the list of mutations found by the automatic analysis and the correct classification of the results according to the importance of the mutations. Variation filtering using annotation information is performed, a clinical report is first prepared, and a final report is issued after confirming the patient, test information, and the type and importance of the detected mutation. Information (VAF, HGVS, sequence, COSMIC, population, and IGV information check), a mutation analysis according to guidelines such as association for molecular pathology, a quality control report, and a customizable clinical report are provided.
In conclusion, our targeted RNA-seq system accurately and efficiently detected various fusion gene mutations that occur in acute leukemia. Importantly, our targeted RNA-seq panel detected the fusion gene mutations that cause Ph-like B-ALL more accurately than a commercially-available targeted system.
Acknowledgments
The authors would like to thank Dr. Veena Selvaratnam, a consultant hematologist from Hospital Ampang, Malaysia, for providing clinical information on other confirmed cases in Malaysia and the medical team involved in the management of this patient. All authors contributed equally to the writing of this manuscript.
REFERENCES
1. Kim HR, Kang MG, Lee YE, et al. 2018; Spectrum of mitochondrial genome instability and implication of mitochondrial haplogroups in Korean patients with acute myeloid leukemia. Blood Res. 53:240–9. DOI: 10.5045/br.2018.53.3.240. PMID: 30310792. PMCID: PMC6170299.


2. Park JH, Kang MG, Kim HR, et al. 2020; Changing the frequency and spectra of chromosomal aberrations in Korean patients with acute leukemia in a tertiary care hospital. Blood Res. 55:225–45. DOI: 10.5045/br.2020.2020255. PMID: 33303709. PMCID: PMC7784131.
3. Lim HJ, Lee JH, Lee SY, et al. 2021; Diagnostic validation of a clinical laboratory-oriented targeted RNA sequencing system for detecting gene fusions in hematologic malignancies. J Mol Diagn. 23:1015–29. DOI: 10.1016/j.jmoldx.2021.05.008. PMID: 34082071.
4. Engvall M, Cahill N, Jonsson BI, Höglund M, Hallböök H, Cavelier L. 2020; Detection of leukemia gene fusions by targeted RNA-sequencing in routine diagnostics. BMC Med Genomics. 13:106. DOI: 10.1186/s12920-020-00739-4. PMID: 32727569. PMCID: PMC7388219. PMID: 5e85005c5d9c4e89b00736435ddcd638.
5. Jain N, Roberts KG, Jabbour E, et al. 2017; Ph-like acute lymphoblastic leukemia: a high-risk subtype in adults. Blood. 129:572–81. DOI: 10.1182/blood-2016-07-726588. PMID: 27919910. PMCID: PMC5290985.


6. El-Menshawy N, Abd-Aziz SM, Elkhamisy EM, Ebrahim MA. 2018; Leukemia propagating cells in Philadelphia chromosome-positive ALL: a resistant phenotype with an adverse prognosis. Blood Res. 53:138–44. DOI: 10.5045/br.2018.53.2.138. PMID: 29963520. PMCID: PMC6021579.


Fig. 1
Frequency of gene fusions detected in various types of leukemia using our targeted RNA-seq system. (A) Black bars indicate the frequency of gene fusions detected in patients with each leukemia type. Gene fusion was detected in 77% (72 patients) of all the 93 leukemia patients. Genetic fusion mutations were observed in 94% (33/35) of adult B-ALL patients and 83% (25/30) of pediatric B-ALL patients. (B) Gene fusion pattern and frequency for each type of leukemia. Among the gene fusions found in adult B-ALL (N=35), the most common fusion gene was BCR-ABL1 (24/33, 73%), and the most common fusion gene mutation in childhood B-ALL was ETV6-RUNX1 (4/26, 15%).
Abbreviation: RNA-seq, RNA sequencing.
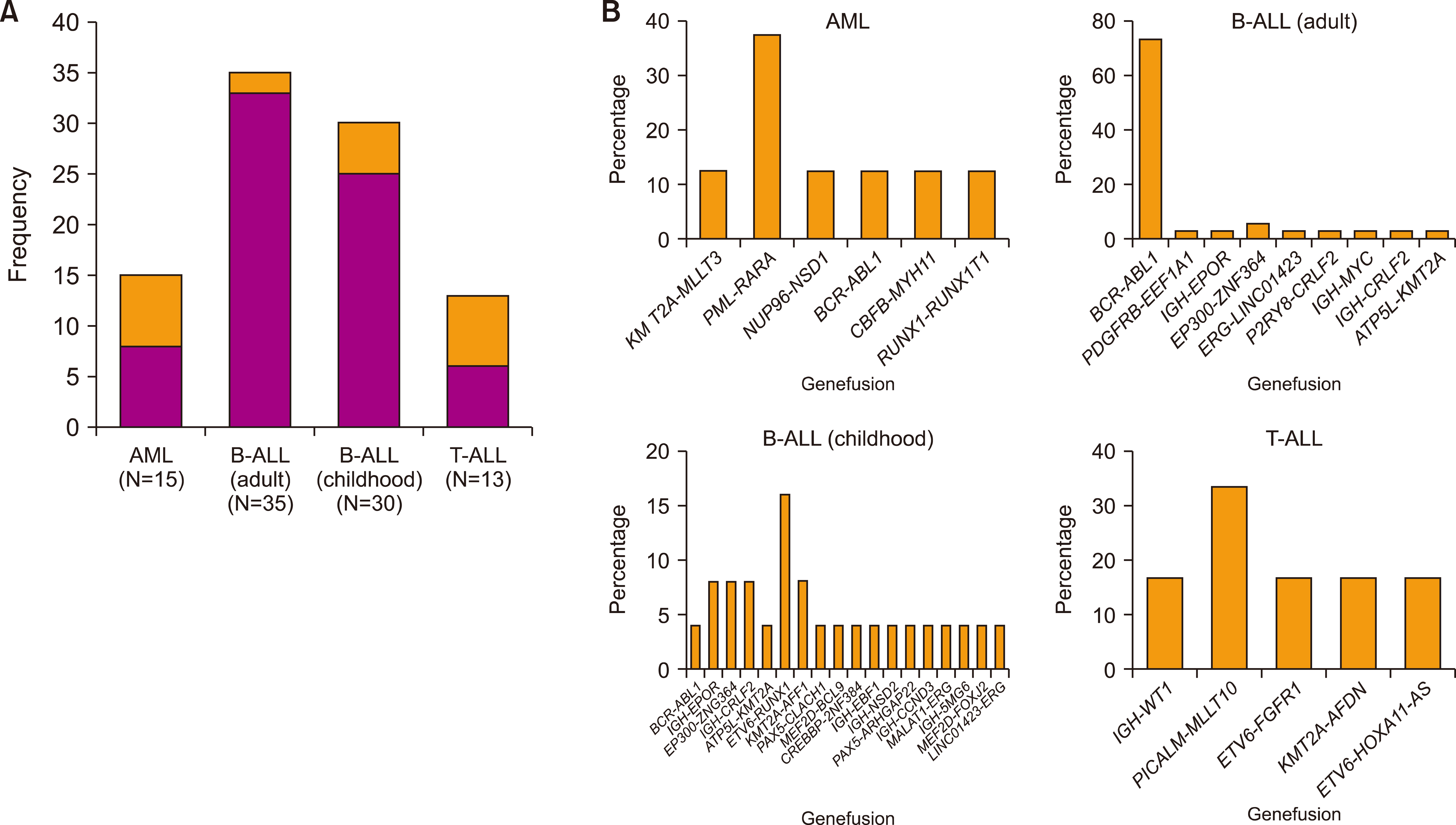
Table 1
Comparative evaluation with a commercially available targeted RNA-seq panel.
Sample no. | Diagnosis | Multiplex RT-PCR or FISH | Commercial targeted RNA-seq system [4] | Breakpoint | Our targeted RNA-seq system [3] | Breakpoint |
---|---|---|---|---|---|---|
1 | APL | PML-RARA | PML-RARA(ex6-ex3) | chr15:74325755 | PML-RARA(ex6-ex3) | chr15:74325755 |
chr17:38504568 | chr17:38504568 | |||||
2 | B-ALL | BCR-ABL1 | BCR-ABL1(ex1-ex2) | chr22:23524426 | BCR-ABL1(ex1-ex2) | chr22:23524426 |
chr9:133729451 | chr9:133729451 | |||||
BCR-ABL1(ex2-ex1) | chr22:23595986 | |||||
chr9:133589842 | ||||||
3 | AML | RUNX1-RUNX1T1 | RUNX1-RUNX1T1(ex6-ex2) | chr21:36231771 | RUNX1-RUNX1T1(ex6-ex2) | chr21:36231771 |
chr8:93029591 | chr8:93029591 | |||||
RUNX1-RUNX1T1(ex6-ex1) | chr21:36231771 | RUNX1-RUNX1T1(ex6-ex3) | chr21:36231771 | |||
chr8:93074855 | chr8:93074937 | |||||
RUNX1-RUNX1T1(ex6-ex1) | chr21:36231771 | RUNX1-RUNX1T1(ex6-ex1) | chr21:36231875 | |||
chr8:93074937 | chr8:93074774 | |||||
4 | B-ALL | ETV6-RUNX1 | ETV6-RUNX1(ex5-in2) | chr12:12022900 | ETV6-RUNX1(ex5-ex3) | chr21:36265260 |
chr21:36265263 | chr12:12022903 | |||||
ETV6-RUNX1(ex5-ex3) | chr12:12022903 | ETV6-RUNX1(ex5-ex4) | chr21:36259393 | |||
chr21:36265260 | chr12:1202290 | |||||
ETV6-RUNX1(ex5-ex4) | chr12:12022903 | |||||
chr21:36259393 | ||||||
ETV6-RUNX1(ex5-in2) | chr12:12022903 | |||||
chr21:36340571 | ||||||
5 | B-ALL | Negative | P2RY8-CRLF2(ex1-ex1) | chrX:1655814 | P2RY8-CRLF2(ex1-ex1) | chrX:1655814 |
chrX:1331530 | chrX:1331529 | |||||
EIF4E3-FOXP1(ex7-ex3) | chr3:71739161 | |||||
chr3:71542706 | ||||||
6 | B-ALL | Negative | Negative | IGH-CRLF2 | chr14:106329453 | |
chrX:1351126 | ||||||
IGH-CRLF2 | chr14:106322373 | |||||
chrX:1351121 | ||||||
IGH-CRLF2 | chr14:106330849 | |||||
chrX:1351119 | ||||||
7 | AML | CBFB-MYH11 | CBFB-MYH11(ex5-ex33) | chr16:67116211 | CBFB-MYH11(ex5-ex33) | |
chr16:15814908 | ||||||
CBFB-MYH11(ex5-ex33) | chr16:67116242 | |||||
chr16:15814908 | ||||||
8 | T-ALL | Negative | PICALM-MLLT10(ex17-ex4) | chr11:85692172 | PICALM-MLLT10(ex17-ex4) | chr11:85692172 |
chr10:21875223 | chr10:21875223 | |||||
PICALM-MLLT10(ex17-ex4) | chr11:85689133 | PICALM-MLLT10(ex7-ex3) | chr11:85687725 | |||
chr10:21875228 | chr10:21827841 | |||||
PICALM-MLLT10(ex17-ex3) | chr11:85692184 | PICALM-MLLT10(ex19-ex3) | chr11:85670103 | |||
chr10:21827830 | chr10:21827841 |