Abstract
Background
Nitric oxide (NO) can induce apoptosis in megakaryocytes. Stimulatory function of NO on platelet production may be important in the pathophysiology of idiopathic thrombocytopenic purpura (ITP). NO is produced by three isoforms of NO synthase (NOS). The endothelial nitric oxide synthase (eNOS) isoform has been detected in platelets. Polymorphism of the eNOS gene, which supplies NO synthesis, changes the functions of this enzyme. In this study, the role of eNOS Glu298Asp gene polymorphism in etiopathogenesis, its course, and treatment of ITP was investigated.
Methods
Sixty-six patients [51 newly diagnosed ITP (ND-ITP), 15 chronic ITP (CH-ITP), and 60 healthy controls (HC)] were enrolled in this study.
Results
In all patients, the frequency of the GT genotype was 48.5%. The frequency of the GG genotype was determined to be 40.9% and the TT genotype was 10.6%. The most common allele in all patients was the G allele. eNOS Glu298Asp gene polymorphism might be a risk factor in the etiopathogenesis of ITP. Patients with the GG genotype were thought to have a high intention for CH-ITP. Patients with the GG genotype responded effectively to medical treatment using IVIG therapy. The presence of the G allele was observed to have a positive effect on the medical treatment of patients with CH-ITP, whereas the T allele exhibited a negative effect.
Oxidative stress and free radicals may be responsible for the pathogenesis and prognosis of idiopathic thrombocytopenic purpura (ITP), which is an autoimmune disorder. Under oxidative stress, lipoproteins and lipids in the cellular structure that undergo peroxidation. ITP-associated platelet destruction and bleeding may play a significant role in the elevation of lipid peroxidation and reduction in the antioxidant capacity of these patients [1-3].
High doses of nitric oxide (NO) result in the formation of toxic molecule peroxynitrite in the presence of cellular superoxide anion. Peroxynitrite may cause cellular injury with lipid peroxidation, DNA fragmentation and depletion of plasma antioxidants [4-7]. NO plays a critical role in preventing thrombus formation and leukocyte adhesion to endothelium. Its insufficient production is associated with thrombosis in clinical disorders of humans. It suppresses exocytosis of Weibel-Palade bodies, endothelial granules that mediate vascular inflammation and thrombosis, by regulating the activity of nethylmaleimide sensitive factor (NSF). NSF is a key component of the exocytic machinery, and NO inhibits NSF-mediated disassembly of soluble NSF attachment protein receptor complexes by nitrosylating critical cysteine residues of the factor [8].
An important mode of inactivation of NO is its reaction with superoxide anions to form the potent oxidant peroxynitrite. This compound can cause oxidative damage of biomolecules including proteins, lipids, and DNA [9].
Stimulatory function of NO on platelet production may be important in the pathophysiology of ITP. It also has important effects on platelets, mainly the inhibition of platelet aggregation [5]. Exogenous and endogenous forms of NO can induce apoptosis in megakaryocytes [10]. Previous studies have been conducted to explore the role of NO in the terminal stages of megakaryocytopoiesis, including megakaryocyte death [11]. Thrombopoetin acting at early stages of platelet formation is essential for proplatelet development, while NO is involved in the later platelet release phase. The concept of NO in megakaryocyte differentiation has clinical relevance because a NO depleted state could lead to increased numbers of megakaryocytes, but not increased platelet production [5].
NO is produced by three isoforms of NO synthase (NOS) (nNOS, iNOS, eNOS). Endothelial nitric oxide synthase (eNOS) is mostly expressed in endothelial cells. The isozyme has also been detected in platelets [9].
Polymorphism of the eNOS, gene which supplies nitric oxide synthesis, changes the functions of this enzyme. In this study, we investigated the role of eNOS Glu298Asp gene polymorphism in etiopathogenesis, its course and treatment of ITP. We described polymorphisms in patients with ITP for the first time.
This was a prospective study. Sixty-six patients including 51 newly diagnosed ITP (ND-ITP) and 15 chronic ITP (CH-ITP), and 60 healthy controls (HC) were enrolled in this study (Table 1).
The diagnosis of ND-ITP was made by detecting isolated thrombocytopenia (platelet counts less than 150×109/L), examining bone marrow aspiration biopsy specimens, and excluding other causes of thrombocytopenia. Patients with hereditary thrombocytopenia, active inflammation, antinuclear antibodies, a positive direct Coombs test, splenomegaly, and those receiving blood transfusion and/or drug therapy were excluded from the study.
The term ND-ITP was defined for all cases at diagnosis (within 3 months from diagnosis). The term CH-ITP was defined for patients with ITP lasting more than 12 months [12]. The treatment used was randomly selected among higher doses of methylprednisolone (HDMP, 30 mg/kg/d for 3, and then 20 mg/kg/d for 4 days), standard dose prednisolone (SDP, 2 mg/kg/day, over several weeks with a taper), intravenous immune globulin (IVIG, 0.5 mg/kg for 5 days), and anti-D (50 µg/kg) [13].
Accepted criteria for treatment responses were based on platelet counts as follows: 1) complete response, ≥100×109/L; 2) partial response, 50–100×109/L; 3) mild response, <50×109/L without any need for treatment, 4) unresponsive or refractory to treatment, <50×109/L, and requirement for treatment [14]. Approval from the Ethics Committee (FÜBAP 1598), and informed consent of the parents were obtained.
Venous blood samples obtained after 8–12 h fasting from cases diagnosed as ITP at the time of diagnosis were collected in tubes with K-EDTA for complete blood count, and each sample was kept frozen at -20°C until the time of isolation and DNA analysis. Blood samples were obtained from the control group who consulted with the healthy children polyclinic (polyclinics of Department of Healthy Children). The control group consisted of patients without any history of ITP or other diseases and bone marrow depressant use. Any blood samples obtained were subjected to the same above-mentioned conditions.
DNA purification was realized using a Wizard Genomic DNA Purification Kit (Promega, Madison, WI, USA). The primer used (oligonucleotide) in the analysis was purchased from Bio Basic (Bio Basic Inc., Ontario, Canada). General directions of usage were followed for the isolation of DNA. Nucleotide sequence of the purchased primer (primer sequences of eNOS gene) manifested F-5’-AAG GCA GGA GAC AGT GGA TGG A-3’, R-5’-CCC AGT CAA TCC CTT TGG TGC TCA-3’ polymorphisms (Fig. 1, Table 2). Polymorphisms were determined by Restriction Fragment Length Polymorphism- Polymerase Chain Reaction (RFLP-PCR).
The fragment Ban II belonging to the locus Glu298Asp GG/GT/TT genotype was amplified by PCR, cleaved by restriction endonuclease, subjected to 2% agarose gel electrophoresis, and genotyped. eNOS Glu298Asp gene polymorphism is shown in Fig. 1 [15]. As seen in Fig. 2 the T allele was detected at 248 base couple (bc), and G allele at 163 and 85 bc bands, respectively.
Statistical analysis was performed using the SPSS v. 12 package program. For intra- and intergroup analyses, a one-way analysis of variance (ANOVA) was applied, and for post- ANOVA tests, LSD and Tukey B tests were used. Differences in genotype distribution were evaluated using a chi-square test. The significance of G and T allele frequencies was evaluated using Fisher’s Exact Test. A P<0.05 was considered to be statistically significant.
Demographic characteristics of patients with ITP and the control group are shown in Table 2. In all patients with ITP and the ND-ITP, CH-ITP and HC groups, the frequencies of the GG genotype were recorded as 40.9%, 39.2%, 46.7% and 21.7%, respectively. The frequencies of the GT genotype were 48.5%, 51%, 40%, 70%, and the TT genotype were 10.6%, 9.8%, 13.3% and 8.3%, respectively. The GT genotype was the most common genotype in all patients with ITP among the ND-ITP and HC groups, while the GG genotype was predominantly found in patients with CH-ITP. The G allele was the most common allele in all patients with ITP among the ND-ITP and HC groups (Fig. 3, Table 3, 4).
Patients with the GG genotype were considered to have high intention for a chronic disease due to the high frequency of the GG genotype identified in patients with CH-ITP (P<0.05). Patients with ND-ITP, CH-ITP and possessing the GG genotype responded effectively to medical treatment using IVIG therapy (except anti-D). Patients with CH-ITP and possessing the GG and TT genotypes responded with resistance to medical treatment (Table 5, 6). The presence of a G allele had a positive effect on the medical treatment of patients with CH-ITP, whereas the T allele exhibited a negative effect. However, patients with GT and TT genotypes in the CH-ITP group were resistant to medical therapy (Table 6).
Pathogenesis of ITP includes autoimmune mechanisms resulting in cellular damage with the formation of hydrogen peroxide which is an oxidant yield. Oxidative stress and free radicals may be responsible for the pathogenesis of ITP [7].
NO, which was initially described as the endothelial derived relaxation factor, is an important messenger molecule involved in many physiological and pathological processes [16]. It is believed to be a major mediator in autoimmune diseases and is synthesized from arginine through the NOS enzyme [15-18]. NO concentration in the body regularly fluctuates at low levels and is controlled by eNOS and nNOS [6].
Endothelium derived NO causes vasorelaxation and also inhibits platelet adhesion and aggregation. Therefore, it maintains blood fluidity and prevents thrombosis. An important function of NO is the modulation of platelet production from the megakaryocytes. ITP can be associated with a decrease in NO bioavailability. Some of the treatment modalities for ITP may be exerting beneficial effects by increasing the levels of NO and thus improving platelet production [5, 15-18].
High doses of NO results in the formation of the toxic molecule peroxynitrite in the presence of cellular superoxide anion. Peroxynitrite can cause cellular injury with lipid peroxidation, DNA fragmentation and depletion of plasma antioxidants. Polymorphism of the eNOS gene which supplies NO synthesis changes the functions of this enzyme [4-7]. We wanted to investigate role of eNOS gene Glu298Asp polymorphism in ITP pathogenesis.
Of the 5 cases diagnosed as infants with ITP in our study, the GG genotype was observed in 3 cases and the GT genotype in 2 cases. Our infant ITP cases did not become chronic and no significant result was obtained in the low number of infants who had ITP.
NO is produced by three isoforms of NOS and these isoforms are encoded by different genes. The localization, regulation, catalytic properties, and inhibitory sensitivities of each isoform are different. Neuronal NOS (nNOS, NOS I) is constitutively expressed in central and peripheral neurons and some other cell types. Its functions include synaptic plasticity in the central nervous system, central regulation of blood pressure, smooth muscle relaxation, and vasodilatation via peripheral nitrergic nerves. Inducible NOS (iNOS, NOS II) can be expressed in many cell types in response to lipopolysaccharide, cytokines, or other agents. iNOS generates large amounts of NO that have cytostatic effects on parasitic target cells. iNOS contributes to the pathophysiology of inflammatory diseases and septic shock. Endothelial NOS (eNOS, NOS III) is mostly expressed in endothelial cells. The isozyme has also been detected in platelets, cardiac myocytes, neurons, syncytiotrophoblasts of the human placenta and kidney tubular epithelial cells. It keeps blood vessels dilated, controls blood pressure, and has numerous other vasoprotective and anti-atherosclerotic effects [9, 16].
There are approximately 161 single nucleotide polymorphisms identified in the eNOS gene to date. The eNOS gene is located on chromosome 7q35–36 and comprises 26 exons. Glu298Asp polymorphism occurs in the seventh exon of the eNOS gene by replacing the guanine (G) nucleotide with thymine (T). Glu298Asp polymorphism, which is of functional importance as it causes the conversion of glutamate (Glu) 298 to aspartate (Asp) in the structure of the enzyme and changes the protein sequence. This causes changes in enzyme function. In view of the physiological and pathophysiological importance of NO, the potential role of eNOS in the pathogenesis of various human diseases has been examined using its polymorphic variants as potential disease markers [19].
A relationship may exist between polymorphisms in the eNOS gene and NO level. eNOS Glu298Asp gene polymorphism has been recorded to reduce basal NO release and is associated with the development of atherosclerosis/hypertension [20].
A significantly lower nitrite/nitrate level was found in exon 7 Glu298Asp gene polymorphism in patients with coronary artery disease and those carrying the TT genotype and T allele [21]. We believe that the mechanisms affecting NO level could cause ITP pathogenesis and different subtypes.
The most common genotype in the ITP group was the GT genotype. The GG genotype was found predominantly in patients with CH-ITP. The G allele was the most common allele in the ND-ITP and HC groups (Fig 3, Table 3).
The presence of mutations in different disease groups has been studied to determine whether a relationship between eNOS gene polymorphisms and various diseases exists. Glu298Asp polymorphism is significantly higher in autoimmune diseases (e.g., systemic lupus erythematosus, rheumatoid arthritis, Behçet’s disease, Sjogren’s syndrome, vasculitis, Takayasu’s arteritis, Henoch Schönlein purpura, polyarteritis nodosa, Wegener granulomatosis, and Churg Straus syndrome) than healthy controls [22, 23]. eNOS polymorphisms that cause reduced NO production are associated with preeclampsia [24]. Although eNOS polymorphism has been studied in many autoimmune diseases, we did not identify any research on eNOS activity or eNOS Glu298Asp polymorphism in ITP.
In the ND-ITP group with the GG genotype; a better response to IVIG and SDP treatment occurred, while the response to anti-D was low. Those in the GT genotype appeared to respond better to HDMP and IVIG therapy, and they did not respond to SDP. Response to medical therapy in the TT genotype was excellent (Table 5). The response to medical therapy in the GG genotype was good in the CH-ITP group. Finally, treatment resistance was detected in the GT genotype and partial response was detected in the TT genotype (Table 6).
In conclusion in the present study, a significant correlation was found between ITP and eNOS Glu298Asp gene polymorphism. This correlation infers that eNOS Glu298Asp gene polymorphism might be a risk factor in the ethiopathogenesis of ITP. Patients with the GG genotype had a high intention for having a CH-ITP. Patients with ND-ITP, CH-ITP and those with the GG genotype responded effectively to medical treatment with IVIG therapy. The G allele exhibited a positive effect on the medical treatment of patients with CH-ITP, while the T allele exhibited a negative effect.
ACKNOWLEDGMENTS
The authors would like to thank the University of Firat Faculty of Medicine for financial support for our study.
REFERENCES
1. Wentworth P Jr, Jones LH, Wentworth AD, et al. 2001; Antibody catalysis of the oxidation of water. Science. 293:1806–11. DOI: 10.1126/science.1062722. PMID: 11546867. PMCID: PMC122400.


2. Jin CQ, Dong HX, Cheng PP, Zhou JW, Zheng BY, Liu F. 2013; Antioxidant status and oxidative stress in patients with chronic ITP. Scand J Immunol. 77:482–7. DOI: 10.1111/sji.12048. PMID: 23551069.
3. Cura M, Koç A, Aksoy N, Özdemir ZC. 2016; Effect of short-term, high-dose methylprednisolone on oxidative stress in children with acute immune thrombocytopenia. Blood Res. 51:261–7. DOI: 10.5045/br.2016.51.4.261. PMID: 28090489. PMCID: PMC5234230.


4. Bivalacqua TJ, Champion HC, Hellstrom WJ. 2002; Implications of nitric oxide synthase isoforms in the pathophysiology of Peyronie's disease. Int J Impot Res. 14:345–52. DOI: 10.1038/sj.ijir.3900872. PMID: 12454685.


5. Thachil J. 2009; Nitric oxide in immune thrombocytopenic purpura. Hematology. 14:59–62. DOI: 10.1179/102453309X385151. PMID: 19154666.


6. Hoffmann A, Gloe T, Pohl U. 2001; Hypoxia-induced upregulation of eNOS gene expression is redox-sensitive: a comparison between hypoxia and inhibitors of cell metabolism. J Cell Physiol. 188:33–44. DOI: 10.1002/jcp.1092. PMID: 11382920.
7. Polat G, Tamer L, Tanriverdi K, Gürkan E, Baslamisli F, Atik U. 2002; Levels of malondialdehyde, glutathione and ascorbic acid in idiopathic thrombocytopaenic purpura. East Afr Med J. 79:446–9. DOI: 10.4314/eamj.v79i8.8833. PMID: 12638848.
8. Mineo C. 2013; Inhibition of nitric oxide and antiphospholipid antibody-mediated thrombosis. Curr Rheumatol Rep. 15:324. DOI: 10.1007/s11926-013-0324-4. PMID: 23519891. PMCID: PMC3625922.


9. Förstermann U, Sessa WC. 2012; Nitric oxide synthases: regulation and function. Eur Heart J. 33:829–37. 837a–d. DOI: 10.1093/eurheartj/ehr304. PMID: 21890489. PMCID: PMC3345541.


10. Battinelli E, Loscalzo J. 2000; Nitric oxide induces apoptosis in megakaryocytic cell lines. Blood. 95:3451–9. DOI: 10.1182/blood.V95.11.3451. PMID: 10828028.


11. Battinelli E, Willoughby SR, Foxall T, Valeri CR, Loscalzo J. 2001; Induction of platelet formation from megakaryocytoid cells by nitric oxide. Proc Natl Acad Sci U S A. 98:14458–63. DOI: 10.1073/pnas.241427398. PMID: 11734646. PMCID: PMC64703.


12. Rodeghiero F, Stasi R, Gernsheimer T, et al. 2009; Standardization of terminology, definitions and outcome criteria in immune thrombocytopenic purpura of adults and children: report from an international working group. Blood. 113:2386–93. DOI: 10.1182/blood-2008-07-162503. PMID: 19005182.
13. Provan D, Stasi R, Newland AC, et al. 2010; International consensus report on the investigation and management of primary immune thrombocytopenia. Blood. 115:168–86. DOI: 10.1182/blood-2009-06-225565. PMID: 19846889. PMCID: PMC6880896.
14. Thude H, Gruhn B, Werner U, et al. 2004; Treatment of a patient with chronic immune thrombocytopenic purpura with rituximab and monitoring by flow cytometric analysis. Acta Haematol. 111:221–4. DOI: 10.1159/000077570. PMID: 15153715.
15. Garg UC, Hassid A. 1989; Nitric oxide-generating vasodilators and 8-bromo-cyclic guanosine monophosphate inhibit mitogenesis and proliferation of cultured rat vascular smooth muscle cells. J Clin Invest. 83:1774–7. DOI: 10.1172/JCI114081. PMID: 2540223. PMCID: PMC303890.


16. Alderton WK, Cooper CE, Knowles RG. 2001; Nitric oxide synthases: structure, function and inhibition. Biochem J. 357:593–615. DOI: 10.1042/bj3570593. PMID: 11463332. PMCID: PMC1221991.
17. Radomski MW, Palmer RM, Moncada S. 1987; Endogenous nitric oxide inhibits human platelet adhesion to vascular endothelium. Lancet. 2:1057–8. DOI: 10.1016/S0140-6736(87)91481-4. PMID: 2889967.
18. Kolb H, Kolb-Bachofen V. 1992; Nitric oxide: a pathogenetic factor in autoimmunity. Immunol Today. 13:157–60. DOI: 10.1016/0167-5699(92)90118-Q. PMID: 1379434.


19. Tesauro M, Thompson WC, Rogliani P, Qi L, Chaudhary PP, Moss J. 2000; Intracellular processing of endothelial nitric oxide synthase isoforms associated with differences in severity of cardiopulmonary diseases: cleavage of proteins with aspartate vs. glutamate at position 298. Proc Natl Acad Sci U S A. 97:2832–5. DOI: 10.1073/pnas.97.6.2832. PMID: 10717002. PMCID: PMC16015.


20. Veldman BA, Spiering W, Doevendans PA, et al. 2002; The Glu298Asp polymorphism of the NOS 3 gene as a determinant of the baseline production of nitric oxide. J Hypertens. 20:2023–7. DOI: 10.1097/00004872-200210000-00022. PMID: 12359981.
21. Yoon Y, Song J, Hong SH, Kim JQ. 2000; Plasma nitric oxide concentrations and nitric oxide synthase gene polymorphisms in coronary artery disease. Clin Chem. 46:1626–30. DOI: 10.1093/clinchem/46.10.1626. PMID: 11017941.


22. Serrano NC, Páez C, Correa PA, Anaya JM. 2004; Endothelial nitric oxide synthase gene polymorphism is associated with systemic lupus erythematosus. J Rheumatol. 31:2163–8. PMID: 15517628.
23. Kim JU, Chang HK, Lee SS, et al. 2003; Endothelial nitric oxide synthase gene polymorphisms in Behçet's disease and rheumatic diseases with vasculitis. Ann Rheum Dis. 62:1083–7. DOI: 10.1136/ard.62.11.1083. PMID: 14583572. PMCID: PMC1754357.
24. Oe Y, Ko M, Fushima T, et al. 2018; Hepatic dysfunction and throm-bocytopenia induced by excess sFlt1 in mice lacking endothelial nitric oxide synthase. Sci Rep. 8:102. DOI: 10.1038/s41598-017-18260-7. PMID: 29311569. PMCID: PMC5758763.


Fig. 2
Agarose gel electrophoresis and schematic view of PCR products cut by Ban II enzyme of eNOS gene Glu298Asp polymorphism. Case 1 and 2: GG genotype, Case 3 and 4: GT genotype, Case 5 and 6: TT genotype. T allele 248 base couple (bc), G allele 163 and 85 bc. M: 100 bc DNA dimension marker.
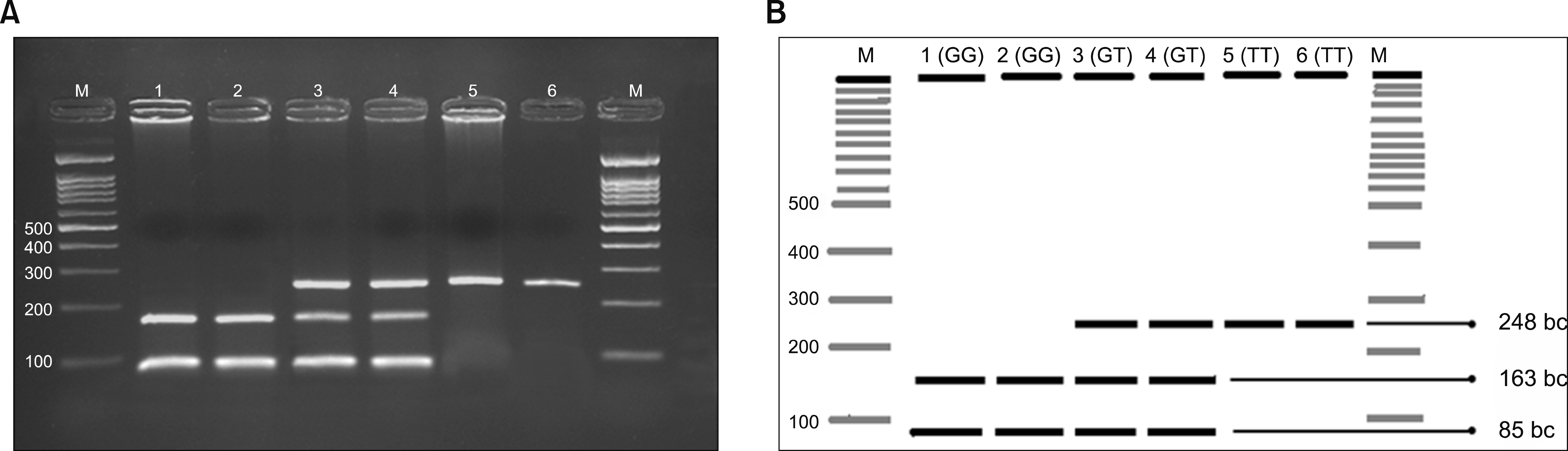
Fig. 3
Dispersion of eNOS Glu298Asp G/T genotype in the patient and control groups. 1a–4a=P<0.05, 1b–4b=P<0.05, 3c–4c=P<0.05, 2a–4a=P<0.05, 2b–4b=P<0.05, 3a–4a=P<0.05, 3b–4b=P<0.05.

Table 1
Demographic characteristics in idiopathic thrombocytopenic purpura (ITP) and control groups.
Table 2
Primer sequences of the eNOS gene.
F-5’-AAGGCAGGAGACAGTGGATGGA 3’ |
R-5’-CCCAGTCAATCCCTTTGGTGCTCA 3’ |
Table 3
Dispersion of genotype and frequency of G/T allele of eNOS gene polymorphism in the patient and control groups.
Table 4
Distribution of eNOS Glu298Asp GG/GT/TT genotypes among groups and its relation with age and thrombocyte parameters.
Table 5
Treatment responses categorized according to genotypes of the patients in the ND-ITP group.
Table 6
Treatment responses categorized according to genotypes of the patients in the CH-ITP group.