Abstract
The increased expression of receptors for advanced glycation end-product (RAGE) is known as a key player in the progression of vascular remodeling. However, the precise signal pathways regulating RAGE expression in vascular smooth muscle cells (VSMCs) in the injured vasculatures are unclear. Given the importance of mitogen-activated protein kinase (MAPK) signaling in cell proliferation, we investigated the importance of MAPK signaling in high-mobility group box 1 (HMGB1)-induced RAGE expression in VSMCs. In HMGB1 (100 ng/ml)-stimulated human VSMCs, the expression of RAGE mRNA and protein was increased in association with an increase in AGE-induced VSMC proliferation. The HMGB1-induced RAGE expression was attenuated in cells pretreated with inhibitors for ERK (PD98059, 10 μM) and p38 MAPK (SB203580, 10 μM) as well as in cells deficient in ERK and p38 MAPK using siRNAs, but not in cells deficient of JNK signaling. In cells stimulated with HMGB1, the phosphorylation of ERK, JNK, and p38 MAPK was increased. This increase in ERK and p38 MAPK phosphorylation was inhibited by p38 MAPK and ERK inhibitors, respectively, but not by JNK inhibitor. Moreover, AGE-induced VSMC proliferation in HMGB1-stimulated cells was attenuated in cells treated with ERK and p38 MAPK inhibitors. Taken together, our results indicate that ERK and p38 MAPK signaling are involved in RAGE expression in HMGB1-stimulated VSMCs. Thus, the ERK/p38 MAPK-RAGE signaling axis in VSMCs was suggested as a potential therapeutic target for vascular remodeling in the injured vasculatures.
Vascular injury initiates infiltration of inflammatory cells into damaged tissues, and causes subsequent release of endogenous damage-associated molecular patterns (DAMPs) including high-mobility group box 1 (HMGB1) [1]. HMGB1 released from activated immune cells or necrotic cells functions as an important inflammatory mediator when present extracellularly [2]. In atherosclerotic plaque, the level of HMGB1 was increased and suggested to be involved in vascular remodeling via the potentiation of inflammatory processes [3,4]. Recent evidences have also indicated that HMGB1 was suggested as a key player in the process of vascular remodeling in the injured vasculatures [5]. Although vascular smooth muscle cells (VSMCs) have been identified as a major source of HMGB1 in atherosclerotic lesions [1,6,7], the precise role played by VSMCs in vascular remodeling in the injured vasculatures is unclear.
When cells are exposed to physical and chemical stresses, the nuclear HMGB1 is translocated outside the cells and then extracellular HMGB1 binds directly to various cell membrane pattern recognition receptors including TLR2, TLR4 and receptors for advanced glycation end-product (RAGE) which were consistently expressed in VSMCs [8-10]. In previous studies, the expression of RAGE in VSMCs at the sites of vascular injury was markedly upregulated compared to that in normal healthy vessels [7,11], thereby enhancing AGE-induced VSMC proliferation [12]. Thus, HMGB1-RAGE interaction has been recognized as a key process involved in the development of vascular remodeling disease [11,13].
The regulation of VSMC proliferation within a normal vascular wall is tightly controlled through the organized balance of several humoral mediators [14,15]. During the atherosclerotic process, the enhanced production of proinflammatory cytokines affects VSMC proliferation, which may contribute to the intimal hyperplasia in atherosclerosis [16,17] Although VSMC proliferation has been implicated as a key process in the progression of vascular remodeling [18,19], the precise role of VSMCs in vascular remodeling in the injured vasculatures is unclear.
RAGE expression is upregulated in the lesion of vascular injury [20] and the increased RAGE expression in VSMCs was indicated as an important player in the development of proliferative vascular diseases [21]. Although the importance of AGE-RAGE interaction in the injured vasculatures has been well established in vascular remodeling [22,23], the intracellular signaling mediating VSMC proliferation remain unclear. Given the importance of mitogen-activated protein kinase (MAPK) signaling in the development of vascular remodeling in the injured vasculatures, the role of MAPK signaling in RAGE expression and AGE-induced cell proliferation in HMGB1-stimulated VSMCs were investigated in this study in order to develop effective therapeutic strategies for vascular remodeling.
Recombinant human HMGB1 was purchased from R&D Systems, Inc. (Minneapolis, MN, USA). AGE-BSA was purchased from BioVision (Milpitas, CA, USA). PD98059 (an ERK inhibitor), SP600125 (a JNK inhibitor) and SB203580 (a p38 MAPK inhibitor) were purchased from Enzo Life Sciences, Inc. (Ann Arbor, MI, USA). RAGE antibodies were purchased from Abcam (Cambridge, MA, USA). β-Actin antibody was purchased from Santa Cruz Biotechnology, Inc (Beverly, MA, USA). p44/42 MAPK (ERK1/2), SAPK/JNK, p38 MAPK, phospho-p44/42 MAPK (ERK1/2) (Thr202/Tyr204), phospho-SAPK/JNK (Thr183/Tyr185) and phospho-p38 MAPK (Thr180/Tyr182) antibodies were purchased from Cell Signaling Technology (Beverly, MA, USA). Horseradish peroxidase (HRP)-conjugated IgG antibody (Santa Cruz Biotechnology, Inc.) was used as the secondary antibody.
Human aortic VSMCs (hVSMCs) purchased from the ATCC (Manassas, VA, USA) were grown in culture dishes using smooth muscle cell growth medium (Gibco BRL, Grand Island, NY, USA), smooth muscle growth supplement (Gibco BRL), 10% fetal bovine serum, antibiotic-antimycotic solution (Gibco BRL). Cells were incubated at 37°C in a humidified atmosphere containing 5% CO2/95% air. hVSMCs between passages 4 and 8 were used for this experiment.
hVSMC proliferation rates were determined by using an MTT assay. In brief, hVSMCs (1 × 105 cells) were treated with a MTT working solution (EZ-Cytox; Daeil Laboratories, Seoul, Korea), and then incubated in a humidified atmosphere containing 5% CO2/95% air for 1 h at 37°C. For ELIZA analysis, the absorbance value of the solution was obtained at a wavelength of 450 nm. Relative proliferation rates were determined by comparing cells with control.
The expression of RAGE mRNA in hVSMCs was determined by RT-PCR using GAPDH as an internal control. In accordance with the manufacturer’s instructions, total RNA was isolated using Trizol reagent (Invitrogen, Carlsbad, NY, USA). RNA (1 μg) was reverse transcribed into cDNA using the ImProm-II reverse transcription system (Promega, Madison, WI, USA). PCR amplification was carried out using RAGE-specific primers (forward, 5´-GC TGT CAG CAT CAG CAT CAT-3´ reverse, 5´-AT TCA GTT CTG CAC GCT CCT-3´). Equal amounts of RT-PCR products obtained were separated on 1%–1.5% agarose gel and then stained with ethidium bromide. Signals from bands were quantified using the UN-SCAN-IT GEL 7.1 program and data were expressed as relative GAPDH densities.
hVSMC lysates were prepared in an ice-cold lysis buffer (Thermo Fisher Scientific, Waltham, MA, USA). Equal amounts of protein were separated on 8%–10% sodium dodecyl sulfate-polyacrylamide gels and then transferred to nitrocellulose membranes (Amersham-Pharmacia Biotech, Piscataway, NJ, USA). Membranes were blocked with 5% skim milk in Tris buffered saline with Tween-20 (TBST) at room temperature for 2 h and then incubated overnight with primary antibody at 4°C. Following this, membranes were washed with TBST, incubated with horseradish peroxidase-conjugated secondary antibody for 2 h at room temperature and then chemiluminescence intensities were measured using image capturing software (Amersham, Imager 680 version. 2.0). Membranes were re-blotted using anti-β-Actin antibody as an internal control. Signals from bands were quantified using the UN-SCAN-IT GEL 7.1 program and data were expressed as relative β-Actin densities.
siRNA for p44/42 MAPK (ERK1/2) (Cat # 6560), SAPK/JNK (Cat # 6232) and p38 MAPK (Cat # 6564) were purchased from Cell Signaling Technology. The siRNA negative control duplex was used as a control oligonucleotide. For siRNA transfection, hVSMCs were seeded and then transfected with MAPKs siRNA using Lipofectamine 2000 reagent (Invitrogen) according to the manufacturer’s protocol.
Results were expressed as the means ± standard error of the means (SEMs). One-way analysis of variance (ANOVA) followed by Dunnett multiple comparison test was performed. Student’s t-test was used to determine significant differences between two groups. Statistical significance was accepted for p-values < 0.05.
To investigate the effects of HMGB1 on RAGE expression in hVSMCs, the expression of RAGE mRNA and protein in cells stimulated with HMGB1 was determined by using RT-PCR and Western blot, respectively. In cultured hVSMCs stimulated with HMGB1 (100 ng/ml) for 0 to 48 h, the expression of RAGE mRNA started to increase at 6 h of HMGB1 stimulation, and then continuously increased until 24 h. Likewise, the RAGE protein expression in cells stimulated with HMGB1 started to increase at 12 h stimulation, and then peaked at 24 h after HMGB1 treatment (Fig. 1).
To increase RAGE expression in VSMCs, hVSMCs were treated with HMGB1 (100 ng/ml) for 24 h. Cell proliferation was induced by AGE and then measured using an MTT assay. As shown in Fig. 2, AGE (30 μg/ml)-induced cell proliferation was markedly increased in VSMCs stimulated with HMGB1 (100 ng/ml) compared to vehicle-treated cells. The inhibitory effects of anti-RAGE antibody on the AGE-induced proliferation in HMGB1-treated cells was more prominent than that in vehicle group. Thus, the RAGE signaling upregulated by HMGB1 was suggested as a key player in VSMC proliferation in the injured vasculatures.
To determine the potential participation of MAPK signaling in VSMC proliferation in the injured vasculatures, this study determined the time-dependent patterns of MAPK phosphorylation in VSMCs stimulated with HMGB1. In this study, hVSMCs were treated with HMGB1 (100 ng/ml) for 0 to 24 h, and then the levels of phosphorylated and total MAPK subfamilies were determined by Western blotting. As shown in Fig. 3A, the increase in ERK phosphorylation in cells stimulated with HMGB1 was peaked at 6 h, and maintained high levels until 24 h. The phosphorylation of JNK and P38 MAPK was time-dependently increased until 24 h after HMGB1 stimulation (Fig. 3B, C).
To determine the individual roles of MAPK subfamilies on HMGB1-induced RAGE expression in hVSMCs, RAGE expression was induced by HMGB1 in the presence of various MAPK inhibitors including inhibitors for ERK (PD98059, 10 μM), JNK (SP600125, 10 μM) and p38 MAPK (SB203580, 10 μM). As shown in Fig. 4, the HMGB1-induced RAGE expression was attenuated in cells pretreated with inhibitors for ERK and p38 MAPK, but not in cells treated with a JNK inhibitor. Likewise, HMGB-1-induced RAGE expression was significantly attenuated in cells deficient of ERK and p38 MAPK using their specific siRNAs, but not in JNK-deficient cells, suggesting a pivotal role of ERK and p38 MAPK signaling in RAGE expression in HMGB1-stimulated cells (Fig. 5).
To further determine crosstalk among ERK, JNK, and p38 MAPK signaling, the HMGB1-induced phosphorylation of ERK and p38 MAPK was determined in cells pretreated with various inhibitors for MAPKs. When VSMCs were stimulated with HMGB1 in the presence of p38 MAPK and JNK inhibitors, ERK phosphorylation was inhibited by SB203580 (10 μM), a P38 MAPK inhibitor, but not by JNK inhibitor. Also, the phosphorylation of p38 MAPK was attenuated by PD98059 (10 μM), an ERK inhibitor, but not by JNK inhibitor, suggesting a link between ERK and p38 MAPK signaling on RAGE expression in the HMGB1-stimulated cells (Fig. 6).
To further confirm whether ERK and p38 MAPK signalings are involved in the augmented proliferative effects of HMGB1 on AGE-stimulated hVSMCs, cells were treated with HMGB1 (100 ng/ml) for 24 h, and then cell proliferation was induced by AGE. In hVSMCs stimulated with HMGB1, AGE-induced cell proliferation was markedly increased, which was attenuated in cells pretreated with PD98059 (10 μM), an ERK inhibitor and SB203580 (10 μM), a p38 MAPK inhibitor, but not by a JNK inhibitor (Fig. 7). These results suggested a pivotal role of ERK and p38 MAPK signaling in regulating RAGE expression and subsequent VSMC proliferation induced by AGE.
The salient findings of this study are that the expression of RAGE mRNA and protein was increased in human VSMCs stimulated with HMGB1, which was accompanied by an augmented cell proliferation induced by AGE. Both HMGB1-induced RAGE expression and HMGB1-augmented effects of AGE-induced VSMC proliferation were markedly attenuated by inhibition of ERK and p38 MAPK signaling, suggesting a potential involvement of the ERK/p38 MAPK-RAGE signaling axis in vascular remodeling in the injured vasculatures.
The regulation of VSMC proliferation within a normal vascular wall is tightly controlled through the organized balance of various humoral mediators [14,15]. During the atherosclerotic process, the enhanced production of proinflammatory cytokines affects VSMC proliferation, which may contribute to intimal hyperplasia in atherosclerosis [16,17]. Among various cells in blood vessel walls, VSMCs are the major cell type and an increased VSMC proliferation is implicated in the pathogenesis of vascular remodeling [18,19]. Although VSMC has been suggested as a key player in vascular remodeling in the injured vasculatures, the precise molecular mechanisms involved in VSMC proliferation are unclear.
Vascular injury-induced infiltration of inflammatory cells into damaged tissues is followed by an increased production of endogenous DAMPs including HMGB1, a best characterized and pivotal DAMPs released into the injured vasculatures [1]. In atherosclerotic lesions, VSMCs, macrophage, and endothelial cells were identified as major sources of HMGB1 production [1,6,7]. The increased HMGB1 in atherosclerotic plaque is known to be involved in vascular remodeling via the potentiation of inflammatory processes [3,4]. Recent evidences have also indicated that HMGB1 released from the injured vasculatures was suggested as a key player mediating vascular remodeling [5]. Although VSMCs have been identified as a major vascular source of HMGB1 in atherosclerotic lesions [1,6,7], the precise role of HMGB1 on VSMC proliferation remains unidentified.
HMGB1 primarily resides in the nucleus of quiescent cells [24], and its release is increased when cells are activated in the injured tissues [1,6]. When cells are exposed to physical and chemical stresses, nuclear HMGB1 can be translocated to cytosol and then released outside the cells via active and passive pathways, and the extracellular HMGB1 may bind to cell surface receptors [8,9]. Among various cell membrane pattern recognition receptors, TLR2, TLR4, and RAGE were consistently expressed in VSMCs in our previous study [10]. In diabetes, the increasing evidence shows that RAGE is recognized as an important molecule involved in the development of vascular remodeling disease [13]. Also, the expression of RAGE in VSMCs at the sites of vascular injury was markedly upregulated compared to that in normal healthy vessels [7,11], thereby enhancing AGE-induced VSMC proliferation [12]. To investigate the mechanisms involved in HMGB1-mediated vascular remodeling, we investigated the regulatory effects of HMGB1 on the expression of RAGE in human VSMCs. In the present study, stimulation of VSMCs with HMGB1 increased RAGE expression, which was accompanied by an augment in AGE-induced cell proliferation. Thus, the HMGB1-RAGE signaling in hVSMCs was suggested as a pivotal pathway involved in vascular remodeling in the injured vasculature.
The MAPK signaling is activated in the injured vasculatures, and the activation of MAPK pathways is shown to be involved in the exaggerated neointimal hyperplasia after balloon injury [25]. In angiotensin II-induced VSMC proliferation, the importance of the increased phosphorylation of ERK1/2, JNK1/2, and p38 MAPK has been demonstrated [26]. Given the importance of MAPK signaling in the neointima hyperplasia in the injured vasculatures, we investigated the role of HMGB1 on the regulation of MAPK activities. In line with other previous study in which HMGB1 activates ERK1/2 and p38 MAPK in endothelial cells [27], the phosphorylation of ERK, JNK and p38 MAPK was increased in hVSMCs treated with HMGB1 in our present study. The increase in ERK phosphorylation in cells stimulated with HMGB1 was peaked at 6 h, and maintained high levels until 24 h. The phosphorylation of JNK and p38 MAPK was time-dependently increased until 24 h after HMGB1 stimulation. Typically, the activation of MAPK is an early event in cellular activation, thus continuous increment of MAPK phosphorylation might be caused by secondary activation by other stimuli including humoral factors. The long-term phosphorylation of MAPK signaling in our present study should be confirmed by future experiments.
The increased RAGE expression has been suggested as a key player in the progression of vascular remodeling. However, the precise mechanisms regulating RAGE expression in VSMCs in the injured vasculatures is unclear. To investigate the importance of MAPK signaling in an increased VSMC proliferation in the injured vasculatures, this study determined the role of MAPK signaling in HMGB1-induced RAGE expression. In cultured hVSMCs stimulated with HMGB1, RAGE mRNA and protein expression were markedly increased, which was attenuated in cells pretreated with inhibitors of ERK and p38 MAPK as well as in cells deficient of ERK and p38 MAPK using siRNAs, but not in cells deficient of JNK. In line with an increased RAGE expression in HMGB1-stimulated cells, VSMC proliferation induced by AGE was markedly augmented in cells stimulated with HMGB1, which was attenuated in cells pretreated with ERK and p38 MAPK inhibitors. Thus, it was suggested that ERK and p38 MAPK activated by HMGB1 mediate RAGE expression in VSMCs, which augments AGE-mediated VSMC proliferation.
To further determine crosstalk among ERK, JNK, and p38 MAPK signaling in vascular remodeling, the HMGB1-induced phosphorylation of ERK and p38 MAPK was determined in cells pretreated with various inhibitors for MAPKs. When VSMCs were stimulated with HMGB1 in the presence of p38 MAPK and JNK inhibitors, ERK phosphorylation was inhibited by a p38 MAPK inhibitor, but not by JNK inhibitor. Also, the phosphorylation of p38 MAPK was attenuated by an ERK inhibitor, but not by JNK inhibitor, suggesting a link between ERK and 38 MAPK signaling in HMGB1-stimulated cells.
Taken together, the results of our present study show that the activation of ERK and p38 MAPK signaling mediates RAGE expression in HMGB1-stimulated hVSMCs, which augments proliferation of human VSMC induced by AGE. Considering the importance of the phenotypic modulation of VSMCs in vascular remodeling, the HMGB1-ERK/p38 MAPK-RAGE signaling axis in VSMCs was suggested as a potential therapeutic target for vascular remodeling in the injured vasculatures.
Notes
REFERENCES
1. Land WG. 2013; Chronic allograft dysfunction: a model disorder of innate immunity. Biomed J. 36:209–228. DOI: 10.4103/2319-4170.117622. PMID: 24225188.


2. Hreggvidsdottir HS, Ostberg T, Wähämaa H, Schierbeck H, Aveberger AC, Klevenvall L, Palmblad K, Ottosson L, Andersson U, Harris HE. 2009; The alarmin HMGB1 acts in synergy with endogenous and exogenous danger signals to promote inflammation. J Leukoc Biol. 86:655–662. DOI: 10.1189/jlb.0908548. PMID: 19564572.


3. Kalinina N, Agrotis A, Antropova Y, DiVitto G, Kanellakis P, Kostolias G, Ilyinskaya O, Tararak E, Bobik A. 2004; Increased expression of the DNA-binding cytokine HMGB1 in human atherosclerotic lesions: role of activated macrophages and cytokines. Arterioscler Thromb Vasc Biol. 24:2320–2325. DOI: 10.1161/01.ATV.0000145573.36113.8a. PMID: 15374849.


4. Cai Y, Sukhova GK, Wong HK, Xu A, Tergaonkar V, Vanhoutte PM, Tang EH. 2015; Rap1 induces cytokine production in pro-inflammatory macrophages through NFκB signaling and is highly expressed in human atherosclerotic lesions. Cell Cycle. 14:3580–3592. DOI: 10.1080/15384101.2015.1100771. PMID: 26505215. PMCID: PMC4825742.
5. Zou H, Yang Y, Gao M, Zhang B, Ming B, Sun Y, Chen H, Tang X, Chen Z, Xiong P, Xu Y, Fang M, Tan Z, Gong F, Zheng F. 2014; HMGB1 is involved in chronic rejection of cardiac allograft via promoting inflammatory-like mDCs. Am J Transplant. 14:1765–1777. DOI: 10.1111/ajt.12781. PMID: 24984831.


6. Wang K, Li W, Yu Q, Guo B, Yang B, Zhang C, Li M, Li J, Hu S, Zheng Q, Song Z. 2017; High mobility group box 1 mediates interferon-γ-induced phenotypic modulation of vascular smooth muscle cells. J Cell Biochem. 118:518–529. DOI: 10.1002/jcb.25682. PMID: 27579780.


7. Porto A, Palumbo R, Pieroni M, Aprigliano G, Chiesa R, Sanvito F, Maseri A, Bianchi ME. 2006; Smooth muscle cells in human atherosclerotic plaques secrete and proliferate in response to high mobility group box 1 protein. FASEB J. 20:2565–2566. DOI: 10.1096/fj.06-5867fje. PMID: 17060403.


8. Kang R, Tang D, Schapiro NE, Loux T, Livesey KM, Billiar TR, Wang H, Van Houten B, Lotze MT, Zeh HJ. 2014; The HMGB1/RAGE inflammatory pathway promotes pancreatic tumor growth by regulating mitochondrial bioenergetics. Oncogene. 33:567–577. DOI: 10.1038/onc.2012.631. PMID: 23318458. PMCID: PMC3795800.


9. Andersson U, Tracey KJ. 2011; HMGB1 is a therapeutic target for sterile inflammation and infection. Annu Rev Immunol. 29:139–162. DOI: 10.1146/annurev-immunol-030409-101323. PMID: 21219181. PMCID: PMC4536551.


10. Jang EJ, Baek SE, Kim EJ, Park SY, Kim CD. 2019; HMGB1 enhances AGE-mediated VSMC proliferation via an increase in 5-LO-linked RAGE expression. Vascul Pharmacol. 118-119:106559. DOI: 10.1016/j.vph.2019.04.001. PMID: 30954689.


11. Leclerc E, Fritz G, Vetter SW, Heizmann CW. 2009; Binding of S100 proteins to RAGE: an update. Biochim Biophys Acta. 1793:993–1007. DOI: 10.1016/j.bbamcr.2008.11.016. PMID: 19121341.


12. Yan SD, Chen X, Fu J, Chen M, Zhu H, Roher A, Slattery T, Zhao L, Nagashima M, Morser J, Migheli A, Nawroth P, Stern D, Schmidt AM. 1996; RAGE and amyloid-beta peptide neurotoxicity in Alzheimer's disease. Nature. 382:685–691. DOI: 10.1038/382685a0. PMID: 8751438.


13. Bierhaus A, Nawroth PP. 2009; Multiple levels of regulation determine the role of the receptor for AGE (RAGE) as common soil in inflammation, immune responses and diabetes mellitus and its complications. Diabetologia. 52:2251–2263. DOI: 10.1007/s00125-009-1458-9. PMID: 19636529.


14. Newby AC. 2006; Matrix metalloproteinases regulate migration, proliferation, and death of vascular smooth muscle cells by degrading matrix and non-matrix substrates. Cardiovasc Res. 69:614–624. DOI: 10.1016/j.cardiores.2005.08.002. PMID: 16266693.


15. Rakesh K, Agrawal DK. 2005; Cytokines and growth factors involved in apoptosis and proliferation of vascular smooth muscle cells. Int Immunopharmacol. 5:1487–1506. DOI: 10.1016/j.intimp.2005.05.003. PMID: 16023601.


16. Doran AC, Meller N, McNamara CA. 2008; Role of smooth muscle cells in the initiation and early progression of atherosclerosis. Arterioscler Thromb Vasc Biol. 28:812–819. DOI: 10.1161/ATVBAHA.107.159327. PMID: 18276911. PMCID: PMC2734458.


17. Dinarello CA. 1996; Biologic basis for interleukin-1 in disease. Blood. 87:2095–2147. DOI: 10.1182/blood.V87.6.2095.bloodjournal8762095. PMID: 8630372.


18. Schwencke C, Schmeisser A, Walter C, Wachter R, Pannach S, Weck B, Braun-Dullaeus RC, Kasper M, Strasser RH. 2005; Decreased caveolin-1 in atheroma: loss of antiproliferative control of vascular smooth muscle cells in atherosclerosis. Cardiovasc Res. 68:128–135. DOI: 10.1016/j.cardiores.2005.05.004. PMID: 15950204.


19. Chistiakov DA, Sobenin IA, Orekhov AN, Bobryshev YV. 2015; Human miR-221/222 in physiological and atherosclerotic vascular remodeling. Biomed Res Int. 2015:354517. DOI: 10.1155/2015/354517. PMID: 26221589. PMCID: PMC4499635.


20. Burke AP, Kolodgie FD, Zieske A, Fowler DR, Weber DK, Varghese PJ, Farb A, Virmani R. 2004; Morphologic findings of coronary atherosclerotic plaques in diabetics: a postmortem study. Arterioscler Thromb Vasc Biol. 24:1266–1271. DOI: 10.1161/01.ATV.0000131783.74034.97. PMID: 15142859.
21. Simard E, Söllradl T, Maltais JS, Boucher J, D'Orléans-Juste P, Grandbois M. 2015; Receptor for advanced glycation end-products signaling interferes with the vascular smooth muscle cell contractile phenotype and function. PLoS One. 10:e0128881. DOI: 10.1371/journal.pone.0128881. PMID: 26248341. PMCID: PMC4527751.


22. Schmidt AM, Stern DM. 2000; RAGE: a new target for the prevention and treatment of the vascular and inflammatory complications of diabetes. Trends Endocrinol Metab. 11:368–375. DOI: 10.1016/S1043-2760(00)00311-8. PMID: 11042467.


23. Schmidt AM, Yan SD, Yan SF, Stern DM. 2001; The multiligand receptor RAGE as a progression factor amplifying immune and inflammatory responses. J Clin Invest. 108:949–955. DOI: 10.1172/JCI200114002. PMID: 11581294. PMCID: PMC200958.


24. Umahara T, Uchihara T, Koyama S, Hashimoto T, Akimoto J, Haraoka J, Iwamoto T. 2014; Local extension of HMGB1 in atherosclerotic lesions of human main cerebral and carotid arteries. Histol Histopathol. 29:235–242. DOI: 10.14670/HH-29.235. PMID: 23929500.
25. Indolfi C, Torella D, Cavuto L, Davalli AM, Coppola C, Esposito G, Carriero MV, Rapacciuolo A, Di Lorenzo E, Stabile E, Perrino C, Chieffo A, Pardo F, Chiariello M. 2001; Effects of balloon injury on neointimal hyperplasia in streptozotocin-induced diabetes and in hyperinsulinemic nondiabetic pancreatic islet-transplanted rats. Circulation. 103:2980–2986. DOI: 10.1161/01.CIR.103.24.2980. PMID: 11413090.


26. Won SM, Park YH, Kim HJ, Park KM, Lee WJ. 2006; Catechins inhibit angiotensin II-induced vascular smooth muscle cell proliferation via mitogen-activated protein kinase pathway. Exp Mol Med. 38:525–534. DOI: 10.1038/emm.2006.62. PMID: 17079869.


27. Liang Y, Hou C, Kong J, Wen H, Zheng X, Wu L, Huang H, Chen Y. 2015; HMGB1 binding to receptor for advanced glycation end products enhances inflammatory responses of human bronchial epithelial cells by activating p38 MAPK and ERK1/2. Mol Cell Biochem. 405:63–71. DOI: 10.1007/s11010-015-2396-0. PMID: 25862459.


Fig. 1
Effects of HMGB1 on RAGE expression in hVSMCs.
The cultured hVSMCs were stimulated with HMGB1 (100 ng/ml) for 0 to 48 h, and then the expression of RAGE mRNA and protein was determined by using RT-PCR and Western blot, respectively. GAPDH and β-Actin were used as internal controls. Blots are representative of 5 independent experiments. Bottom: Relative intensities were quantified and expressed as the means ± SEMs of 5 independent experiments. HMGB1, high-mobility group box 1; RAGE, receptors for advanced glycation end-product; hVSMCs, human vascular smooth muscle cells; MW, molecular weight. *p < 0.05, **p < 0.01 vs. corresponding value at 0 h.

Fig. 2
Effects of HMGB1 on AGE-induced proliferation of hVSMCs.
The cultured hVSMCs were stimulated with HMGB1 (100 ng/ml) for 24 h, and then cell proliferation was induced by AGE (30 μg/ml) for 24 h in the presence or absence of anti-RAGE (10 μg/ml). Cell proliferation determined by an MTT assay was quantified and expressed as the means ± SEMs of 3–4 independent experiments. HMGB1, high-mobility group box 1; hVSMCs, human vascular smooth muscle cells. **p < 0.01 vs. corresponding control in non-AGE, ##p < 0.01 vs. corresponding control in vehicle, and ††p < 0.01 vs. corresponding anti-RAGE effects in vehicle.
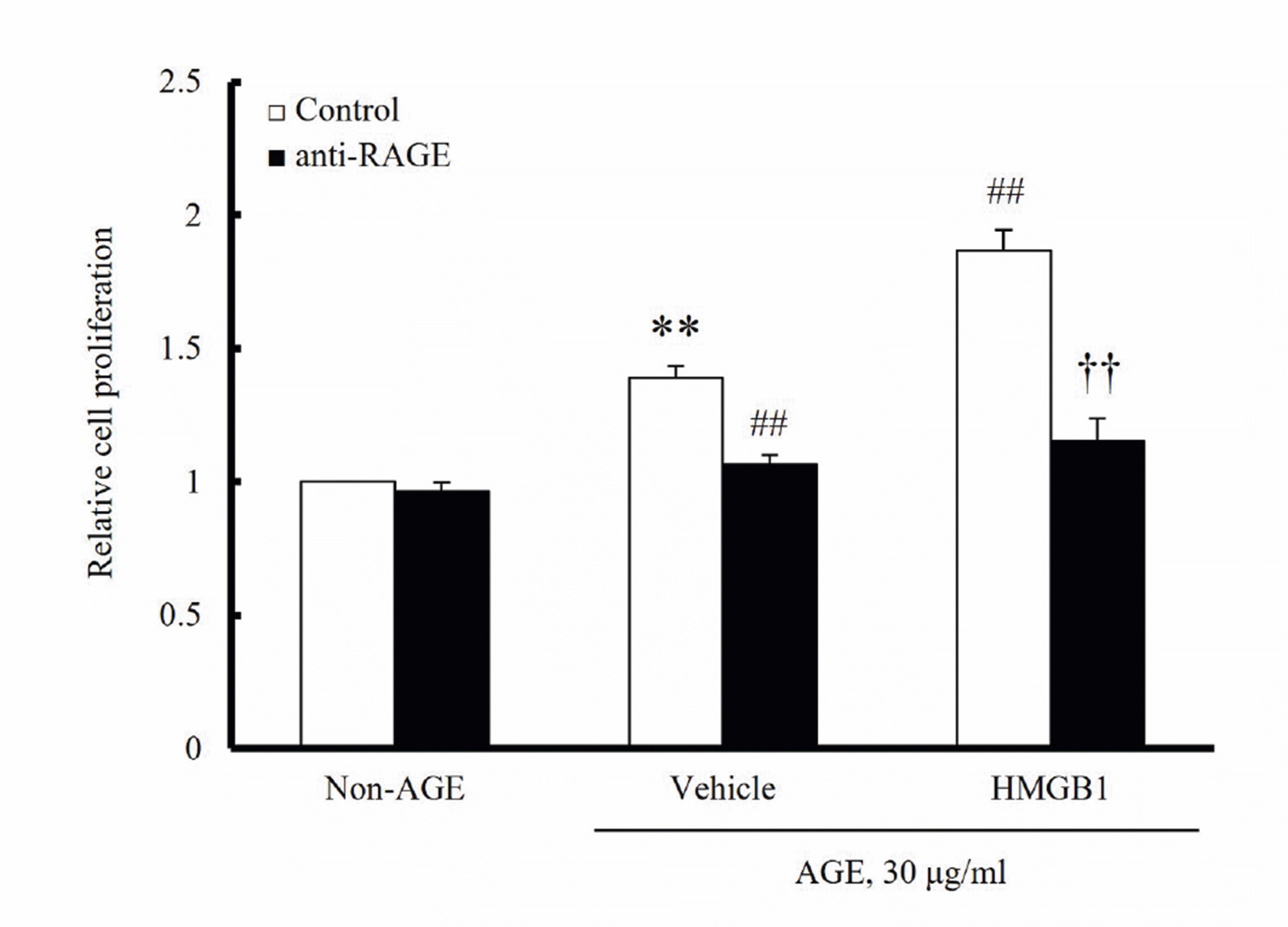
Fig. 3
Characteristics of ERK, JNK, and p38 MAPK phosphorylation in HMGB1-stimulated hVSMCs.
hVSMCs were stimulated with HMGB1 (100 ng/ml) for 0 to 24 h, and then the levels of phosphorylated and total ERK (A), JNK (B), and p38 MAPK (C) were determined by Western blotting. Blots are representative of 3–4 independent experiments. Bottom: Relative intensities of phosphorylated to total ERK, JNK, and p38 MAPK were quantified and expressed as the means ± SEMs of 3–4 independent experiments. MAPK, mitogen-activated protein kinase; HMGB1, high-mobility group box 1; hVSMCs, human vascular smooth muscle cells. *p < 0.05, **p < 0.01 vs. value in 0 h.
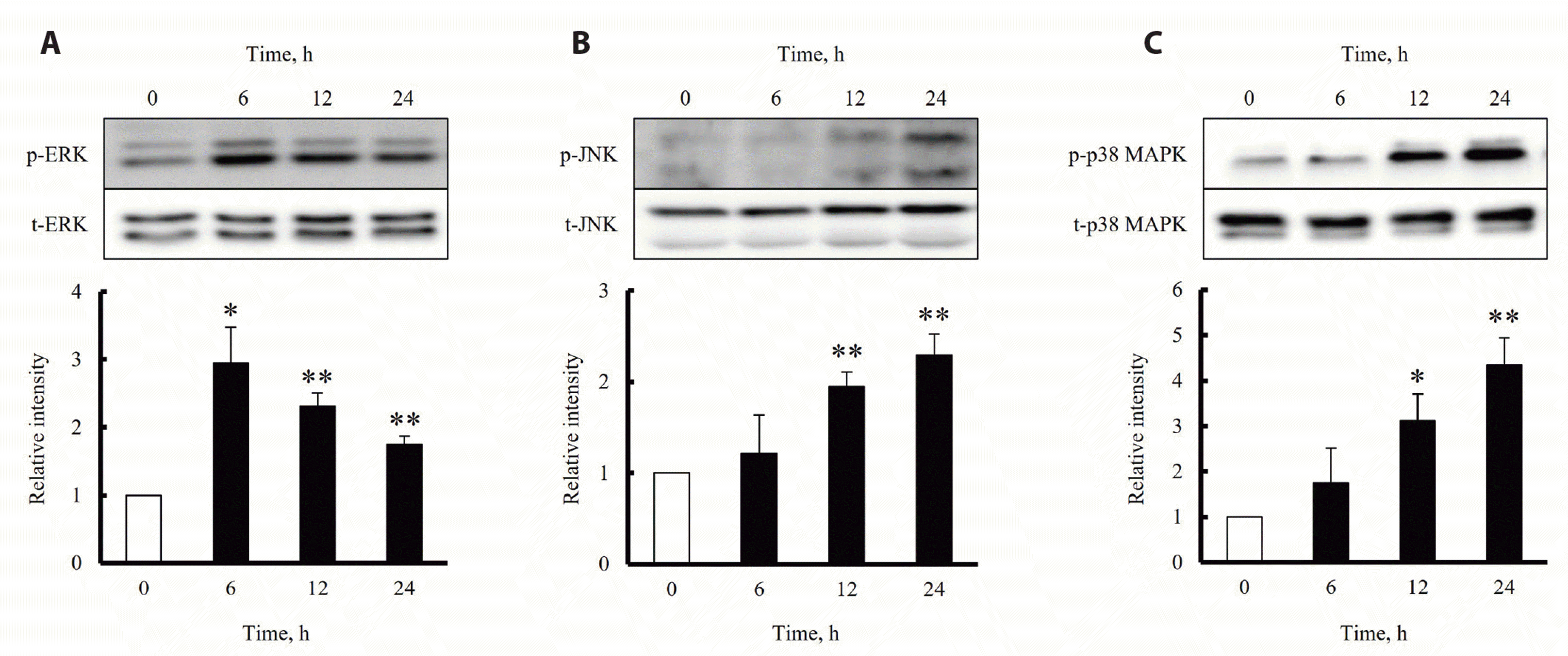
Fig. 4
Involvement of MAPK signaling in HMGB1-induced RAGE expression in hVSMCs.
hVSMCs were treated with inhibitors of ERK (PD98059, 10 μM), JNK (SP600125, 10 μM), and p38 MAPK (SB203580, 10 μM) for 30 min, and then cells were stimulated with HMGB1 (100 ng/ml) for 24 h. The expression of RAGE in HMGB1-stimulated cells was determined by Western blot using β-Actin as an internal control. Blots are representative of 5 independent experiments. Bottom: Relative RAGE intensities to β-Actin were quantified, and data were expressed as the means ± SEMs of 5 independent experiments. MAPK, mitogen-activated protein kinase; HMGB1, high-mobility group box 1; RAGE, receptors for advanced glycation end-product; hVSMCs, human vascular smooth muscle cells. **p < 0.01 vs. corresponding value in control. #p < 0.05 vs. corresponding value in vehicle.

Fig. 5
Individual role of MAPK signaling in HMGB1-induced RAGE expression in hVSMCs.
hVSMCs were transfected with siRNAs for (A) ERK (10 μM), (B) JNK (10 μM), and (C) p38 MAPK (10 μM) for 48 h, and then cells were stimulated with HMGB1 (100 ng/ml) for 24 h. RAGE expression in HMGB1-stimulated cells was determined by Western blot. β-Actin was used as internal controls. Blots are representative of 5 independent experiments. Bottom: Relative intensities of MAPK subfamilies and RAGE to β-Actin were quantified and expressed as the means ± SEMs of 5 independent experiments. MAPK, mitogen-activated protein kinase; HMGB1, high-mobility group box 1; RAGE, receptors for advanced glycation end-product; hVSMCs, human vascular smooth muscle cells. **p < 0.01 vs. corresponding value in negative control.

Fig. 6
Crosstalk among MAPK subfamilies in HMGB1-stimulated hVSMCs.
To determine crosstalk among ERK, JNK, and p38 MAPK signaling, the phosphorylation of ERK and p38 MAPK in cells stimulated with HMGB1 was determined in the presence of various inhibitors for MAPKs. (A) hVSMCs were treated with inhibitors for p38 MAPK (SB203580, 10 μM) and JNK (SP600125, 10 μM) for 30 min, and then stimulated with HMGB1 (100 ng/ml) for 24 h. The levels of phosphorylated and total ERK were determined by Western blotting. Blots are representative of 5 independent experiments. (B) hVSMCs were treated with inhibitors for ERK (PD98059, 10 μM) and JNK (SP600125, 10 μM) for 30 min, and then stimulated with HMGB1 (100 ng/ml) for 24 h. The levels of phosphorylated and total p38 MAPK were determined by Western blotting. Blots are representative of 5 independent experiments. Bottom: Relative intensities of phosphorylated to total ERK and p38 MAPK were quantified and expressed as the means ± SEMs of 5 independent experiments. MAPK, mitogen-activated protein kinase; HMGB1, high-mobility group box 1; hVSMCs, human vascular smooth muscle cells. *p < 0.05, **p < 0.01 vs. corresponding controls. #p < 0.05 vs. corresponding vehicle.
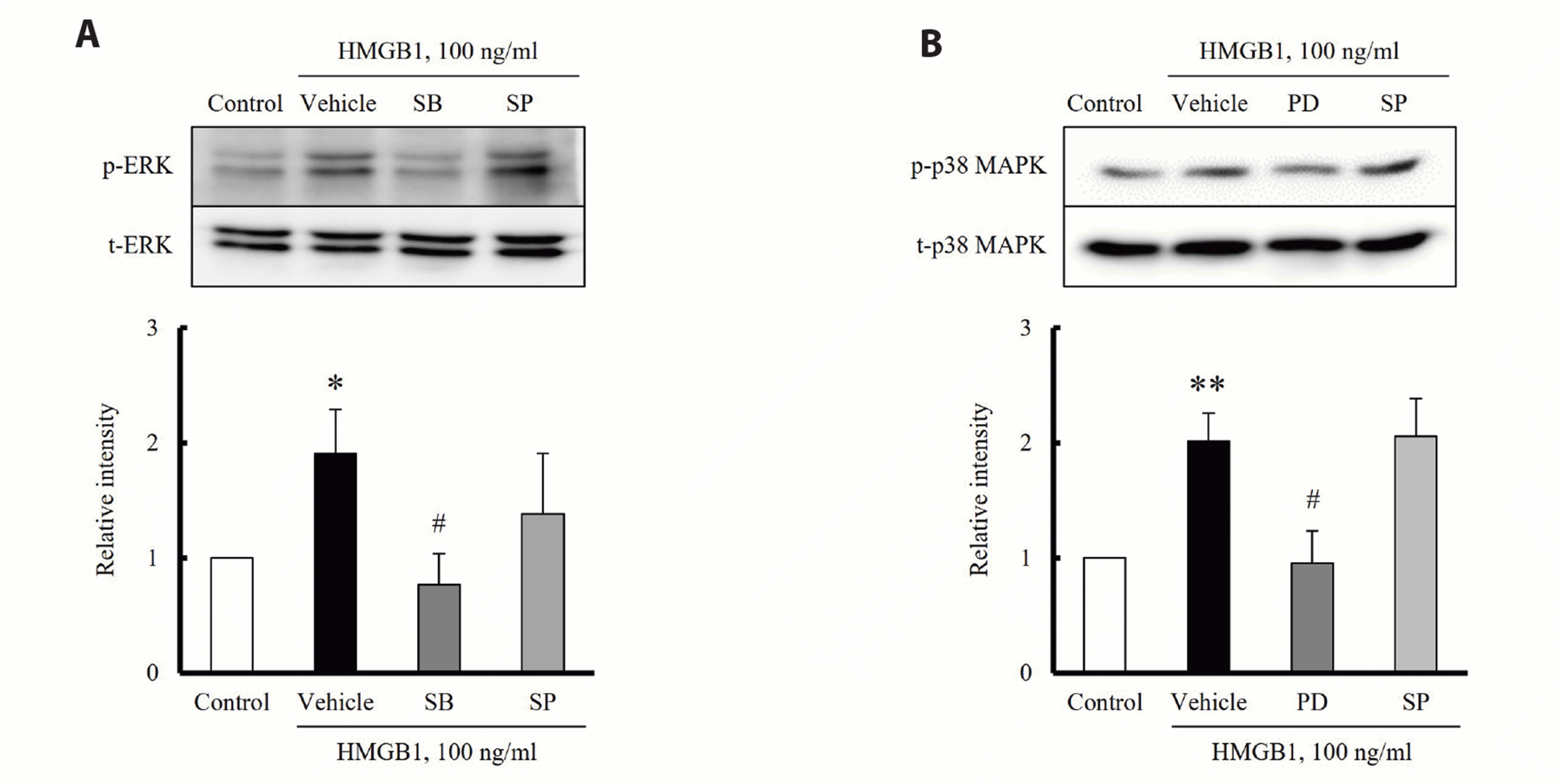
Fig. 7
Role of ERK and p38 MAPK signaling on HMGB1-augmented proliferation in AGE-stimulated VSMCs.
hVSMCs were pre-treated with HMGB1 (100 ng/ml) for 24 h in the presence of various inhibitors for MAPKs including ERK (PD98059, 10 μM), JNK (SP600125, 10 μM), and p38 MAPK (SB203580, 10 μM), and then stimulated with AGE (30 μg/ml) for 24 h. Cell proliferation was determined by an MTT assay. Relative cell proliferation was quantified and expressed as the means ± SEMs of 5 independent experiments. MAPK, mitogen-activated protein kinase; HMGB1, high-mobility group box 1; VSMCs, vascular smooth muscle cells; hVSMCs, human VSMCs. **p < 0.01 vs. corresponding control, #p < 0.05 vs. corresponding value in non-HMGB1, ††p < 0.01 vs. corresponding value in vehicle.
