Abstract
Gastric cancer stem cells (GCSCs) are a major cause of radioresistance and chemoresistance in gastric cancer (GC). Therefore, targeting GCSCs is regarded as a powerful strategy for the effective treatment of GC. Atorvastatin is a widely prescribed cholesterol-lowering drug that inhibits 3-hydroxy-3-methylglutaryl-coenzyme A reductase, a rate-limiting enzyme in the mevalonate pathway. The anticancer activity of atorvastatin, a repurposed drug, is being investigated; however, its therapeutic effect and molecular mechanism of action against GCSCs remain unknown. In this study, we evaluated the anticancer effects of atorvastatin on MKN45-derived GCSCs. Atorvastatin significantly inhibited the proliferative and tumorsphere-forming abilities of MKN45 GCSCs in a mevalonate pathway-independent manner. Atorvastatin induced cell cycle arrest at the G0/G1 phase and promoted apoptosis by activating the caspase cascade. Furthermore, atorvastatin exerted an antiproliferative effect against MKN45 GCSCs by inhibiting the expression of cancer stemness markers, such as CD133, CD44, integrin α6, aldehyde dehydrogenase 1A1, Oct4, Sox2, and Nanog, through the downregulation of β-catenin, signal transducer and activator of transcription 3, and protein kinase B activities. Additionally, the combined treatment of atorvastatin and sorafenib, a multi-kinase targeted anticancer drug, synergistically suppressed not only the proliferation and tumorsphere formation of MKN45 GCSCs but also the in vivo tumor growth in a chick chorioallantoic membrane model implanted with MKN45 GCSCs. These findings suggest that atorvastatin can therapeutically eliminate GCSCs.
Gastric cancer (GC), a malignant tumor that develops in the gastrointestinal tract, is the fifth most commonly diagnosed cancer and the fourth leading cause of cancer-related deaths worldwide [1]. GC is caused by environmental factors, such as Helicobacter pylori infection and poor dietary habits, and genetic factors [2]. Surgical resection and chemotherapy remain the standard treatment modality for GC; however, the prognosis of advanced or metastatic GC remains poor.
The persistent presence of cancer stem cells (CSCs) is associated with the poor prognosis of patients [3]. CSCs are a subpopulation of tumors that are characterized by self-renewal, multilineage differentiation, and tumor initiation abilities [2]. Several stem cell regulators, including CD44, CD133, integrin α6, aldehyde dehydrogenase 1A1 (ALDH1A1), Oct4, Sox2, and Nanog, have been identified as major biomarkers for gastric cancer stem cells (GCSCs) [4,5]. GC cells positive for cell surface proteins CD133, CD44, and integrin α6 have higher tumorigenic and metastatic potential than the negative subpopulation [3]. ALDH1A1 overexpression induces cancer stem-like features and chemoresistance in GC cells [6]. The transcription factors Oct4, Sox2, and Nanog play critical roles in maintaining the pluripotency and self-renewal characteristics of GCSCs [7]. Therefore, the inhibition of these cancer stemness regulators is necessary to effectively eliminate GCSCs.
Drug repurposing is the application of drugs to diseases other than those approved for original indications [8]. Preclinical and clinical investigations on pharmacokinetics and pharmacodynamics of repurposed drugs have previously been completed; therefore, there is growing interest to discover new repurposed drugs with anticancer activity. Statins are potent competitive inhibitors of 3-hydroxy-3-methylglutaryl-coenzyme A (HMG-CoA) reductase (HMGCR), which is a rate-limiting enzyme in the mevalonate pathway, approved by the Food and Drug Administration (FDA) as a lipid-lowering drug [9]. Recent studies have reported the potential of statins as repurposed drugs with anticancer activities [10,11]. Statin drugs, including simvastatin, lovastatin, and atorvastatin, inhibit proliferation and induce apoptosis of several different cancer cells by inhibiting the prenylation of various proteins, such as members of the Ras and Rho GTPase families [10-12]. Recently, statins have been reported to inhibit the proliferation and self-renewal ability of CSCs [13,14]. Lovastatin inhibited the stemness properties and induces apoptosis of nasopharyngeal carcinoma CSCs [13]. Simvastatin suppressed invasion and migration, and induced spheroid disassembly and cell death in ovarian CSCs by decreasing stemness and epithelial-mesenchymal transition (EMT) marker expression and inactivating the Hippo/YAP/RhoA pathway in a mevalonate synthesis-dependent manner [14]. Atorvastatin, in combination with celecoxib and tipifarnib, inhibited the proliferation of pancreatic CSCs by reducing the expression of stemness markers, including CD44, CD133, and ALDH1A1, through the inactivation of protein kinase B (AKT) and nuclear factor-κB (NF-κB) [15]. However, the anticancer effects of statins on GCSCs remain unclear. In this study, we investigated the anticancer effect and underlying molecular mechanism of atorvastatin, a lipophilic statin, on MKN45-derived GCSCs.
Atorvastatin, DL-Mevalonolactone, and sorafenib were purchased from Tocris Bioscience (Bristol, UK), Tokyo Chemical Industry (Tokyo, Japan), and Sigma-Aldrich (Saint Louis, MO, USA), respectively. RPMI-1640 was obtained from Corning Cellgro (Manassas, VA, USA). DMEM/F12 and trypsin were obtained from HyClone (Marlborough, MA, USA). Epidermal growth factor (EGF) and basic fibroblast growth factor (bFGF) were obtained from Prospecbio (East Brunswick, NJ, USA). Fetal bovine serum (FBS), Matrigel, penicillin-streptomycin-amphotericin B, heparin, and Accutase were purchased from R&D systems (Minneapolis, MN, USA), BD Biosciences (San Jose, CA, USA), Lonza (Basel, Switzerland), Sigma-Aldrich, and EMD Millipore (Temecula, CA, USA), respectively. B-27 serum-free supplement, L-glutamine, and penicillin/streptomycin were obtained from Gibco (Grand Island, NY, USA). The CellTiter-Glo 2.0 Cell Viability Assay kit was purchased from Promega (Madison, WI, USA). The Muse Annexin V & Dead Cell and Muse Cell Cycle kits were purchased from Luminex Corporation (Austin, TX, USA). Anti-CDK4 (#sc-70831) and anti-HMGCR (#sc-271595) antibodies were purchased from Santa Cruz Biotechnology (Dallas, TX, USA). Antibodies against cyclin D1 (#2922), cleaved caspase-9 (#9501), cleaved caspase-3 (#9661), PARP (#9542), ALDH1A1 (#12035), integrin α6 (#3750), CD44 (#37259), CD133 (#64326), Nanog (#3580), Oct4 (#2750), Sox2 (#3579), phospho-GSK3β (#9322), GSK3β (#9315), β-catenin (#9562), phospho-STAT3 (#9145), STAT3 (#9139), phospho-ERK1/2 (#9101), ERK1/2 (#9102), phospho-AKT (#4060), AKT (#9272), phospho-NF-κB p65(#3033), NF-κB p65 (#8242), rabbit IgG (#7074), and mouse IgG (#7076) were purchased from Cell Signaling Technology (Danvers, MA, USA). Anti-β-actin (#ab6276) antibody was purchased from Abcam (Cambridge, UK).
The human gastric adenocarcinoma cell line, MKN45 was obtained from the Korean Cell Line Bank (Seoul, Korea). The cells were grown in RPMI-1640 supplemented with 10% FBS and 1% penicillin–streptomycin–amphotericin B. To propagate GCSCs, MKN45 cells were cultured in DMEM/F12 containing 1× B-27, 5 µg/ml heparin, 2 mM L-glutamine, 20 ng/ml EGF, 20 ng/ml bFGF, and 1% penicillin/streptomycin as described previously [16,17]. Tumorspheres grown in serum-free media were subcultured by dissociation using Accutase. The adherent and tumorsphere cells were maintained at 37°C in a humidified CO2 incubator with 5% CO2 (Thermo Scientific, Vantaa, Finland).
MKN45-derived GCSCs (5 × 103 cells/well) were seeded in a 96-white-well culture plate using serum-free media and treated with the different concentrations of each compound for 7 days. Cell proliferation was measured using the CellTiter-Glo 2.0 Cell Viability Assay as described previously [16,17]. Luminescence was detected using a multimode microplate reader (BioTek, Inc., Winooski, VT, USA). The IC50 value from the obtained data was analyzed using the GraphPad Prism 5 (GraphPad Software, La Jolla, CA, USA).
MKN45-derived GCSCs (3 × 103 cells/well) were seeded in a 96-well culture plate using serum-free media and treated with the different concentrations of each compound for 7 days. The number of tumorspheres > 70 µm in diameter was counted under an optical microscope (Olympus, Tokyo, Japan).
MKN45-derived GCSCs (1 × 105 cells/well) were seeded in a 60-mm culture plate using serum-free media and treated with the different concentrations of each compound for 4 days. The cells were harvested, fixed with 70% ethanol, and stained with 200 µl of Muse Cell Cycle reagent as described previously [16,17]. Cell cycle plots were obtained using the Guava Muse Cell Analyzer (MuseSoft_V1.8.0.3; Luminex Corporation).
MKN45-derived GCSCs (1 × 105 cells/well) were seeded in a 60-mm culture plate using serum-free media and treated with the different concentrations of atorvastatin for 4 days. The cells were collected and stained with 100 µl of Muse Annexin V & Dead Cell reagent as described previously [16,17]. Cellular apoptosis was quantitatively analyzed using the Guava Muse Cell Analyzer.
The cell lysates were separated using 7.5%–15% sodium dodecyl sulfate-polyacrylamide gel electrophoresis. The separated proteins were transferred to polyvinylidene difluoride membranes (EMD Millipore, Hayward, CA, USA), and the blots were blocked and immunolabeled with the primary antibodies (dilution 1:500–1:10,000) as described previously [16,17]. After washing with TBST, the membranes were incubated with horseradish peroxidase-conjugated secondary antibodies (dilution 1:3,000). Immunolabeling was detected using an enhanced chemiluminescence kit (Bio-Rad Laboratories, Hercules, CA, USA) according to the manufacturer’s instructions. The band density was analyzed using ImageJ 1.5 software (NIH, Bethesda, MD, USA). Expression levels were determined as the normalized ratio of each target protein to β-actin or phospho-protein to total protein.
To evaluate the effects of the compounds on GC tumorigenesis in vivo, a modified CAM assay was performed as described previously [16]. Briefly, fertilized chicken eggs were incubated at 37°C in a humidified egg incubator for 6 days, and the eggshell membrane was carefully peeled away. MKN45-derived GCSCs (1 × 106 cells/egg) were mixed with Matrigel (30 µl/egg) in the absence or presence of the compounds and implanted onto the CAM inside the silicone ring. After incubation for 7 days, the tumor formed on the CAM was retrieved, and the tumor weight was measured.
The results are expressed as the mean ± standard deviation from at least three independent experiments. Statistical analysis was performed by analysis of variance with Tukey’s post-hoc test using SPSS 9.0 software (SPSS Inc., Chicago, IL, USA). Statistical significance was set at p < 0.05.
To propagate the CSC population from the GC cell line, MKN45 cells were grown in a serum-free spheroid suspension culture. The stem-like properties of GC cells are accompanied by upregulation of stemness-related factors. The expression levels of several key GCSC markers, including CD133, Oct4, and Sox2, were significantly increased in MKN45 tumorsphere cells cultured in serum-free media containing EGF and bFGF compared to MKN45 adherent cells cultured in 10% FBS-supplemented media (Fig. 1A). These data indicate that serum-free spheroid culture can expand the MKN45-derived GCSC population. In this study, we used MKN45 GCSCs at passage 3 with the highest expression levels of stem cell markers that promote the cancer stem-like features.
To evaluate whether atorvastatin affects the proliferation of GCSCs, the MKN45-derived GCSCs were treated with atorvastatin at various concentrations for 7 days, and cell proliferation was measured using an ATP-monitoring luminescence assay. Atorvastatin dose-dependently inhibited the proliferation of MKN45 GCSCs with 2.1 µM of IC50 value (Fig. 1B). Moreover, atorvastatin reduced the number and size of tumorspheres formed by MKN45 GCSCs (Fig. 1C, D). These results demonstrate that atorvastatin inhibits the proliferation of GCSCs. Based on the IC50 value, we further assessed the effects of atorvastatin on MKN45 GCSCs at concentrations ranging from 0–10 µM.
Next, we investigated whether the antiproliferative effect of atorvastatin on MKN45 GCSCs is related to HMGCR activity inhibition. No significant difference in HMGCR protein expression level was observed between MKN45 adherent and tumorsphere cells (Fig. 1E). Interestingly, treatment with mevalonate did not affect the suppressive effect of atorvastatin on the proliferation and tumorsphere formation of MKN45 GCSCs (Fig. 1F–H). These data suggest that atorvastatin exerts an anticancer effect against MKN45 GCSCs in a manner independent of the mevalonate-mediated pathway.
To determine whether atorvastatin exerted an antiproliferative effect on MKN45 GCSCs by regulating the cell cycle and apoptosis, we first investigated the effect of atorvastatin on the cell cycle distribution of MKN45 GCSCs by flow cytometry. Atorvastatin caused a significant increase in the cell population in the G0/G1 phase, along with a decrease in the cell population in the S and G2/M phases, compared to the untreated control cells (Fig. 2A). Next, apoptosis of MKN45 GCSCs was analyzed by flow cytometry using Annexin V-FITC and PI dual staining. Atorvastatin increased the proportion of apoptotic cells in a dose-dependent manner compared with that in the untreated control group (Fig. 2B).
Furthermore, we elucidated the molecular mechanism of cell cycle arrest and the apoptosis-inducing effect of atorvastatin in MKN45 GCSCs. Atorvastatin significantly decreased the protein expression of cyclin-dependent kinase 4 (CDK4) and cyclin D1, which are important regulators of the transition from the G0/G1 phase to the S phase of the cell cycle, in MKN45 GCSCs (Fig. 2C). Moreover, atorvastatin markedly increased the protein levels of cleaved caspase-9, cleaved caspase-3, and cleaved poly (ADP-ribose) polymerase (PARP) in MKN45 GCSCs. Collectively, these results demonstrated that the antiproliferative effect of atorvastatin on MKN45 GCSCs resulted from the induction of G0/G1 cell cycle arrest and caspase-dependent apoptosis.
We investigated whether atorvastatin affected the expression of key stemness-related markers in GCSCs. The results showed that atorvastatin effectively suppressed the expression of cancer stemness regulators, including CD44, CD133, integrin α6, ALDH1A1, Oct4, Sox2, and Nanog, in MKN45 GCSCs (Fig. 3A).
Several signaling pathways, such as Wnt/β-catenin, JAK/STAT, AKT, ERK1/2, and NF-κB, contribute to the self-renewal, tumor progression, and chemoresistance of GCSCs by upregulating the expression of cancer stemness markers [4,18]. Thus, we assessed the effect of atorvastatin on the upstream signaling pathways in MKN45 GCSCs. Atorvastatin increased the protein expression level of glycogen synthase kinase 3β (GSK3β) by inhibiting its phosphorylation, thereby resulting in a significant decrease in β-catenin protein expression (Fig. 3B). In addition, atorvastatin significantly inhibited the expression of phosphorylated STAT3 and AKT compared to their total protein expression levels. However, atorvastatin did not cause an obvious decrease in the expression levels of phosphorylated ERK1/2 and NF-κB. These results imply that atorvastatin inhibits the expression of cancer stemness-related markers by downregulating the Wnt/β-catenin, STAT3, and AKT signaling pathways in MKN45 GCSCs.
Sorafenib is a tyrosine kinase inhibitor that shows anticancer effects against various tumors, including GC; however, its clinical application is often limited by drug resistance [19]. To further explore the therapeutic potential of atorvastatin in GCSCs, we evaluated the effect of atorvastatin on the chemosensitivity of MKN45 GCSCs to sorafenib. The combination of atorvastatin and sorafenib synergistically inhibited both the proliferative and tumorsphere formation abilities of MKN45 GCSCs (Fig. 4A–C). Moreover, the combined treatment of the two drugs more strongly induced cell cycle arrest at the G0/G1 phase compared to single-drug treatment (Fig. 4D).
Next, we assessed the combination effects of atorvastatin and sorafenib on GC tumorigenesis using a CAM tumor model injected with MKN45 GCSCs. Co-treatment with atorvastatin and sorafenib more potently reduced the tumor weight in comparison with single-drug treatment, indicating that the combined treatment of the two drugs synergistically suppressed the growth of MKN45 GCSCs both in vitro and in vivo (Fig. 4E). These findings suggest that atorvastatin can be used in combination with sorafenib to treat GC by targeting GCSCs.
GC is a common malignant tumor worldwide, with a poor prognosis due to recurrence, metastasis, and drug resistance [3]. GCSCs significantly contribute to the refractory features of GC and are thus regarded as an important target of anticancer drugs to improve the outcomes of patients with GC [2]. Accumulating evidence has shown that statins exhibit potential anticancer activity in GC, alone or in combination therapy [20,21]. In GC cell lines, statins inhibited proliferation and activated apoptosis [20]. They synergistically reduced tumor growth in conjunction with radiation or chemotherapy in GC animal models [21]. In addition, statins improved overall survival of patients with GC after surgery and adjuvant chemotherapy [22]. However, the anticancer effects of statins on GCSCs remain unclear. In the present study, we demonstrated, for the first time, the antiproliferative effect and underlying molecular mechanism of atorvastatin against MKN45 GCSCs. Atorvastatin inhibited the proliferation of MKN45 GCSCs by inducing cell cycle arrest at the G0/G1 phase and promoting caspase-dependent apoptosis.
Although statins are approved only for the treatment of hypercholesterolemia, increasing evidence suggests that they are useful against other diseases, including cancer [23-25]. Atorvastatin is administered as a clinical treatment for hypercholesterolemia at 10–80 mg/day [26]. Several nationwide cohort studies have revealed that the administration of statins reduced the risk of GC and its overall mortality [27,28]. In several human cancer types, the antitumor mechanisms of statins are associated with the inhibition of HMGCR, which converts HMG-CoA to mevalonate [23,24]. The blockade of mevalonate synthesis by statins consequently interrupts post-translational modifications, such as farnesylation and geranylgeranylation, of several proteins that are essential for cancer cell proliferation and survival, including Ras and Rho GTPases [10-12]. Moreover, activation of the mevalonate pathway promotes EMT and the stemness properties of cancer cells [14]. However, recent studies have revealed that statins exert HMGCR-independent therapeutic effects [25]. Simvastatin and atorvastatin exhibit mevalonate metabolism-independent antibacterial and anti-inflammatory activities, respectively [25]. Interestingly, our results showed that the inhibitory effect of atorvastatin on the proliferation of MKN45 GCSCs might not result from the suppression of mevalonate-mediated pathways. Therefore, further studies are needed to elucidate the mevalonate pathway-independent mechanism responsible for the anti-proliferative effect of atorvastatin in GCSCs.
Several signaling pathways, such as Wnt/β-catenin, STAT3, AKT, ERK1/2, and NF-κB, play important roles in maintaining the stem-like features of GC cells by increasing the expression of major cancer stemness markers, including CD44, CD133, integrin α6, ALDH1A1, Sox2, Oct4, and Nanog [4,5,18]. Our data demonstrated that atorvastatin inhibited the expression of cancer stemness regulators by downregulating the Wnt/β-catenin, STAT3, and AKT signaling pathways in MKN45 GCSCs. Recent studies have reported the crosstalk between these signaling molecules in various cancers, including GC. The PI3K/AKT pathway activates the Wnt/GSK3β/β-catenin signaling pathway, which in turn upregulates the transcriptional activity of STAT3 [29,30]. Therefore, atorvastatin may exhibit anticancer effects against MKN45 GCSCs by impeding the crosstalk between the Wnt/β-catenin, STAT3, and AKT signaling pathways.
In recent studies, fluvastatin and lovastatin have shown synergistic anticancer effects in combination with a FDA-approved multi-kinase inhibitor, sorafenib, in hepatocelluar and advanced renal cell carcinomas, respectively [19,31]. Atorvastatin also shows enhanced anticancer effects in combination with other clinical drugs, such as docetaxel, aspirin, celecoxib, and tipifarnib, in several cancer cells [15,32,33]. However, combination therapy with atorvastatin and sorafenib in GCSCs has not yet been evaluated. Our results demonstrated that atorvastatin significantly increased the chemosensitivity of MKN45 GCSCs to sorafenib both in vitro and in vivo. Therefore, these findings suggest that atorvastatin is an attractive anticancer drug to eradicate GCSCs, either alone or in combination with sorafenib.
Notes
REFERENCES
1. Sung H, Ferlay J, Siegel RL, Laversanne M, Soerjomataram I, Jemal A, Bray F. 2021; Global cancer statistics 2020: GLOBOCAN estimates of incidence and mortality worldwide for 36 cancers in 185 countries. CA Cancer J Clin. 71:209–249. DOI: 10.3322/caac.21660. PMID: 33538338.


2. Addeo M, Di Paola G, Verma HK, Laurino S, Russi S, Zoppoli P, Falco G, Mazzone P. 2021; Gastric cancer stem cells: a glimpse on metabolic reprogramming. Front Oncol. 11:698394. DOI: 10.3389/fonc.2021.698394. PMID: 34249759. PMCID: PMC8262334. PMID: 5113d6d411584c32ae8304ef46ea252b.


3. Li K, Dan Z, Nie YQ. 2014; Gastric cancer stem cells in gastric carcinogenesis, progression, prevention and treatment. World J Gastroenterol. 20:5420–5426. DOI: 10.3748/wjg.v20.i18.5420. PMID: 24833872. PMCID: PMC4017057.


4. Hsieh HL, Yu MC, Cheng LC, Yeh TS, Tsai MM. 2022; Molecular mechanism of therapeutic approaches for human gastric cancer stem cells. World J Stem Cells. 14:76–91. DOI: 10.4252/wjsc.v14.i1.76. PMID: 35126829. PMCID: PMC8788185.


5. Fukamachi H, Seol HS, Shimada S, Funasaka C, Baba K, Kim JH, Park YS, Kim MJ, Kato K, Inokuchi M, Kawachi H, Yook JH, Eishi Y, Kojima K, Kim WH, Jang SJ, Yuasa Y. 2013; CD49f(high) cells retain sphere-forming and tumor-initiating activities in human gastric tumors. PLoS One. 8:e72438. DOI: 10.1371/journal.pone.0072438. PMID: 24015244. PMCID: PMC3756075. PMID: 9aeb12924f07494a82525baa8726fe5e.


6. Nishikawa S, Konno M, Hamabe A, Hasegawa S, Kano Y, Ohta K, Fukusumi T, Sakai D, Kudo T, Haraguchi N, Satoh T, Takiguchi S, Mori M, Doki Y, Ishii H. 2013; Aldehyde dehydrogenase high gastric cancer stem cells are resistant to chemotherapy. Int J Oncol. 42:1437–1442. DOI: 10.3892/ijo.2013.1837. PMID: 23440340.


7. Liu J, Ma L, Xu J, Liu C, Zhang J, Liu J, Chen R, Zhou Y. 2013; Spheroid body-forming cells in the human gastric cancer cell line MKN-45 possess cancer stem cell properties. Int J Oncol. 42:453–459. DOI: 10.3892/ijo.2012.1720. PMID: 23229446. PMCID: PMC3583623.


8. Sleire L, Førde HE, Netland IA, Leiss L, Skeie BS, Enger PØ. 2017; Drug repurposing in cancer. Pharmacol Res. 124:74–91. DOI: 10.1016/j.phrs.2017.07.013. PMID: 28712971.


9. Rimpelová S, Kolář M, Strnad H, Ruml T, Vítek L, Gbelcová H. 2021; Comparison of transcriptomic profiles of MiaPaCa-2 pancreatic cancer cells treated with different statins. Molecules. 26:3528. DOI: 10.3390/molecules26123528. PMID: 34207840. PMCID: PMC8226792. PMID: c244182daf034fcaba7cfcb59a3d4577.


10. Alizadeh J, Zeki AA, Mirzaei N, Tewary S, Rezaei Moghadam A, Glogowska A, Nagakannan P, Eftekharpour E, Wiechec E, Gordon JW, Xu FY, Field JT, Yoneda KY, Kenyon NJ, Hashemi M, Hatch GM, Hombach-Klonisch S, Klonisch T, Ghavami S. 2017; Mevalonate cascade inhibition by simvastatin induces the intrinsic apoptosis pathway via depletion of isoprenoids in tumor cells. Sci Rep. 7:44841. DOI: 10.1038/srep44841. PMID: 28344327. PMCID: PMC5366866.


11. Ma Q, Gao Y, Xu P, Li K, Xu X, Gao J, Qi Y, Xu J, Yang Y, Song W, He X, Liu S, Yuan X, Yin W, He Y, Pan W, Wei L, Zhang J. 2019; Atorvastatin inhibits breast cancer cells by downregulating PTEN/AKT pathway via promoting Ras homolog family member B (RhoB). Biomed Res Int. 2019:3235021. DOI: 10.1155/2019/3235021. PMID: 31011573. PMCID: PMC6442491.


12. Wong WW, Clendening JW, Martirosyan A, Boutros PC, Bros C, Khosravi F, Jurisica I, Stewart AK, Bergsagel PL, Penn LZ. 2007; Determinants of sensitivity to lovastatin-induced apoptosis in multiple myeloma. Mol Cancer Ther. 6:1886–1897. DOI: 10.1158/1535-7163.MCT-06-0745. PMID: 17575117.


13. Peng Y, He G, Tang D, Xiong L, Wen Y, Miao X, Hong Z, Yao H, Chen C, Yan S, Lu L, Yang Y, Li Q, Deng X. 2017; Lovastatin inhibits cancer stem cells and sensitizes to chemo- and photodynamic therapy in nasopharyngeal carcinoma. J Cancer. 8:1655–1664. DOI: 10.7150/jca.19100. PMID: 28775785. PMCID: PMC5535721.


14. Kato S, Liberona MF, Cerda-Infante J, Sánchez M, Henríquez J, Bizama C, Bravo ML, Gonzalez P, Gejman R, Brañes J, García K, Ibañez C, Owen GI, Roa JC, Montecinos V, Cuello MA. 2018; Simvastatin interferes with cancer 'stem-cell' plasticity reducing metastasis in ovarian cancer. Endocr Relat Cancer. 25:821–836. DOI: 10.1530/ERC-18-0132. PMID: 29848667.


15. Xu XT, Chen J, Ren X, Ma YR, Wang X, Ma YY, Zhao DG, Zhou RP, Zhang K, Goodin S, Li DL, Zheng X. 2021; Effects of atorvastatin in combination with celecoxib and tipifarnib on proliferation and apoptosis in pancreatic cancer sphere-forming cells. Eur J Pharmacol. 893:173840. DOI: 10.1016/j.ejphar.2020.173840. PMID: 33359649.


16. Han JM, Kim YJ, Jung HJ. 2022; Discovery of a new CaMKII-targeted synthetic lethal therapy against glioblastoma stem-like cells. Cancers (Basel). 14:1315. DOI: 10.3390/cancers14051315. PMID: 35267623. PMCID: PMC8909660. PMID: 314dfc13954b42699bcd0921802c58e0.


17. Kim YJ, Yuk N, Shin HJ, Jung HJ. 2021; The natural pigment violacein potentially suppresses the proliferation and stemness of hepatocellular carcinoma cells in vitro. Int J Mol Sci. 22:10731. DOI: 10.3390/ijms221910731. PMID: 34639072. PMCID: PMC8509727. PMID: e3bed64d3de248c69a1be395d86d558f.


18. Yang L, Shi P, Zhao G, Xu J, Peng W, Zhang J, Zhang G, Wang X, Dong Z, Chen F, Cui H. 2020; Targeting cancer stem cell pathways for cancer therapy. Signal Transduct Target Ther. 5:8. DOI: 10.1038/s41392-020-0110-5. PMID: 32296030. PMCID: PMC7005297.


19. Cheng Y, Luo R, Zheng H, Wang B, Liu Y, Liu D, Chen J, Xu W, Li A, Zhu Y. 2017; Synergistic anti-tumor efficacy of sorafenib and fluvastatin in hepatocellular carcinoma. Oncotarget. 8:23265–23276. DOI: 10.18632/oncotarget.15575. PMID: 28423574. PMCID: PMC5410302.


20. Liu Q, Xia H, Zhou S, Tang Q, Zhou J, Ren M, Bi F. 2020; Simvastatin inhibits the malignant behaviors of gastric cancer cells by simultaneously suppressing YAP and β-catenin signaling. Onco Targets Ther. 13:2057–2066. DOI: 10.2147/OTT.S237693. PMID: 32210573. PMCID: PMC7074824.
21. Follet J, Corcos L, Baffet G, Ezan F, Morel F, Simon B, Le Jossic-Corcos C. 2012; The association of statins and taxanes: an efficient combination trigger of cancer cell apoptosis. Br J Cancer. 106:685–692. DOI: 10.1038/bjc.2012.6. PMID: 22294184. PMCID: PMC3322964.


22. Yang PR, Tsai YY, Chen KJ, Yang YH, Shih WT. 2020; Statin use improves overall survival of patients with gastric cancer after surgery and adjuvant chemotherapy in Taiwan: a nationwide matched cohort study. Cancers (Basel). 12:2055. DOI: 10.3390/cancers12082055. PMID: 32722425. PMCID: PMC7464880. PMID: d90b338dfa9a446883d714540cc7f0ad.


23. Göbel A, Zinna VM, Dell'Endice S, Jaschke N, Kuhlmann JD, Wimberger P, Rachner TD. 2020; Anti-tumor effects of mevalonate pathway inhibition in ovarian cancer. BMC Cancer. 20:703. DOI: 10.1186/s12885-020-07164-x. PMID: 32727400. PMCID: PMC7388525. PMID: 9646a631756b47db9076b98eec6dd654.


24. Jiang P, Mukthavaram R, Chao Y, Nomura N, Bharati IS, Fogal V, Pastorino S, Teng D, Cong X, Pingle SC, Kapoor S, Shetty K, Aggrawal A, Vali S, Abbasi T, Chien S, Kesari S. 2014; In vitro and in vivo anticancer effects of mevalonate pathway modulation on human cancer cells. Br J Cancer. 111:1562–1571. DOI: 10.1038/bjc.2014.431. PMID: 25093497. PMCID: PMC4200085.


25. Lüthje P, Walker S, Kamolvit W, Mohanty S, Pütsep K, Brauner A. 2019; Statins influence epithelial expression of the anti-microbial peptide LL-37/hCAP-18 independently of the mevalonate pathway. Clin Exp Immunol. 195:265–276. DOI: 10.1111/cei.13217. PMID: 30216432. PMCID: PMC6330647.


26. Gunache RO, Bounegru AV, Apetrei C. 2021; Determination of atorvastatin with voltammetric sensors based on nanomaterials. Inventions. 6:57. https://doi.org/10.3390/inventions6030057. DOI: 10.3390/inventions6030057. PMID: 2da12e42f9fe4545873803da2940615a.


27. Kwon MJ, Kang HS, Kim JH, Kim JH, Kim SH, Kim NY, Nam ES, Min KW, Choi HG. 2021; Association between statin use and gastric cancer: a nested case-control study using a national health screening cohort in Korea. Pharmaceuticals (Basel). 14:1283. DOI: 10.3390/ph14121283. PMID: 34959682. PMCID: PMC8707102. PMID: e11e083dab7f430ea09fe6a62eaaedb4.


28. Cho MH, Yoo TG, Jeong SM, Shin DW. 2021; Association of aspirin, metformin, and statin use with gastric cancer incidence and mortality: a nationwide cohort study. Cancer Prev Res (Phila). 14:95–104. DOI: 10.1158/1940-6207.CAPR-20-0123. PMID: 32938643.


29. Anand M, Lai R, Gelebart P. 2011; β-catenin is constitutively active and increases STAT3 expression/activation in anaplastic lymphoma kinase-positive anaplastic large cell lymphoma. Haematologica. 96:253–261. DOI: 10.3324/haematol.2010.027086. PMID: 20971814. PMCID: PMC3031693.


30. Cross DA, Alessi DR, Cohen P, Andjelkovich M, Hemmings BA. 1995; Inhibition of glycogen synthase kinase-3 by insulin mediated by protein kinase B. Nature. 378:785–789. DOI: 10.1038/378785a0. PMID: 8524413.


31. Bil J, Zapala L, Nowis D, Jakobisiak M, Golab J. 2010; Statins potentiate cytostatic/cytotoxic activity of sorafenib but not sunitinib against tumor cell lines in vitro. Cancer Lett. 288:57–67. DOI: 10.1016/j.canlet.2009.06.022. PMID: 19632769.


32. Chen X, Liu Y, Wu J, Huang H, Du Z, Zhang K, Zhou D, Hung K, Goodin S, Zheng X. 2016; Mechanistic study of inhibitory effects of atorvastatin and docetaxel in combination on prostate cancer. Cancer Genomics Proteomics. 13:151–160. PMID: 26912805. PMCID: PMC5203772.
33. He Y, Huang H, Farischon C, Li D, Du Z, Zhang K, Zheng X, Goodin S. 2017; Combined effects of atorvastatin and aspirin on growth and apoptosis in human prostate cancer cells. Oncol Rep. 37:953–960. DOI: 10.3892/or.2017.5353. PMID: 28075470.


Fig. 1
Atorvastatin inhibits the proliferation of MKN45 gastric cancer stem cells (GCSCs) in a mevalonate pathway-independent manner.
(A) Expression levels of key GCSC markers in MKN45 adherent and tumorsphere cells during serial cell passaging (passages 1–3) under different culture conditions. The protein expression was analyzed by Western blotting. β-actin was used as a loading control. ***p < 0.001. Each value represents the mean ± SD from three independent experiments. (B) Effect of atorvastatin on the proliferation of MKN45 GCSCs. The cells were treated with atorvastatin (0–25 µM) for 7 days. Cell proliferation was measured using the CellTiter-Glo luminescent assay system. ***p < 0.001 vs. the control. (C, D) Effect of atorvastatin on the tumorsphere-forming ability of MKN45 GCSCs. The cells were treated with atorvastatin (0–25 µM) for 7 days. The number of tumorspheres was counted under an optical microscope (scale bar, 100 μm). ***p < 0.001 vs. the control. (E) Protein expression levels of 3-hydroxy-3-methylglutaryl-coenzyme A reductase (HMGCR) in MKN45 adherent and tumorsphere cells. (F–H) Effect of atorvastatin on the proliferation (F) and tumorsphere-forming ability (G, H) of MKN45 GCSCs in the absence or presence of mevalonate. The cells were treated with atorvastatin and mevalonate for 7 days (scale bar, 100 μm). ***p < 0.001.

Fig. 2
Atorvastatin induces cell cycle arrest and apoptosis in MKN45 gastric cancer stem cells (GCSCs).
(A–C) MKN45 GCSCs were treated with atorvastatin for 4 days. (A) The cell cycle distribution was evaluated using a Muse Cell Analyzer with Muse Cell Cycle kit following the manufacturer’s instructions. (B) Apoptotic cells were detected using a Muse Cell Analyzer with Muse Annexin V & Dead Cell kit following the manufacturer’s instructions. (C) The protein expression levels of the cell cycle and apoptosis regulators were detected by western blotting. β-actin was used as a loading control. PARP, poly (ADP‐ribose) polymerase; CDK4, cyclin-dependent kinase 4. **p < 0.01, ***p < 0.001 vs. the control.
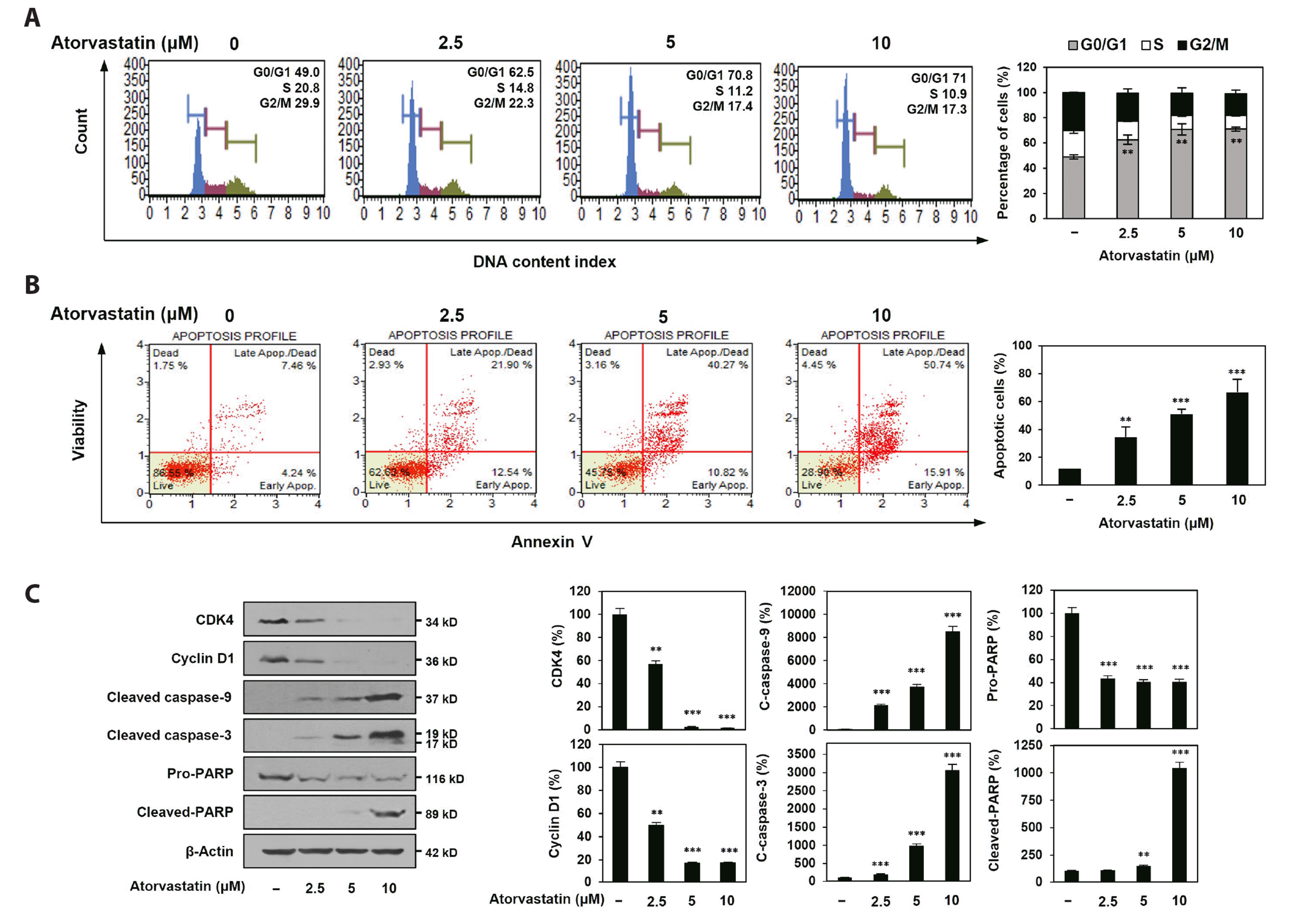
Fig. 3
Atorvastatin downregulates the expression of cancer stemness markers and Wnt/β-catenin/signal transducer and activator of transcription 3 (STAT3)/protein kinase B (AKT) signaling pathways in MKN45 gastric cancer stem cells (GCSCs).
(A, B) MKN45 GCSCs were treated with atorvastatin for 2 days. The protein expression levels were determined by western blotting. β-actin was used as an internal control. ALDH1A1, aldehyde dehydrogenase 1A1; NF-κB, nuclear factor-κB; GSK3β, glycogen synthase kinase 3β. **p < 0.01, ***p < 0.001 vs. the control.

Fig. 4
Atorvastatin increases the chemosensitivity of MKN45 gastric cancer stem cells (GCSCs) to sorafenib in vitro and in vivo.
(A–C) MKN45 GCSCs were treated with the indicated concentrations of sorafenib and atorvastatin for 7 days. (A) Cell proliferation was measured using the CellTiter-Glo luminescent assay system. (B, C) The number of formed tumorspheres was counted under an optical microscope (scale bar, 100 μm). (D) MKN45 GCSCs were treated with the indicated concentrations of sorafenib and atorvastatin for 2 days. Cell cycle distribution was evaluated using a Muse Cell Analyzer with Muse Cell Cycle kit. (E) MKN45 GCSCs were mixed with Matrigel in the absence or presence of sorafenib (5 µg/egg) and atorvastatin (10 µg/egg) and implanted onto the chorioallantoic membrane (CAM) surface inside the silicone ring of fertilized chick eggs. After incubation for 7 days, the CAMs were photographed, the formed tumors were retrieved, and the tumor weight was calculated. NT, no treatment. *p < 0.05, **p < 0.01, ***p < 0.001 vs. the compound alone.
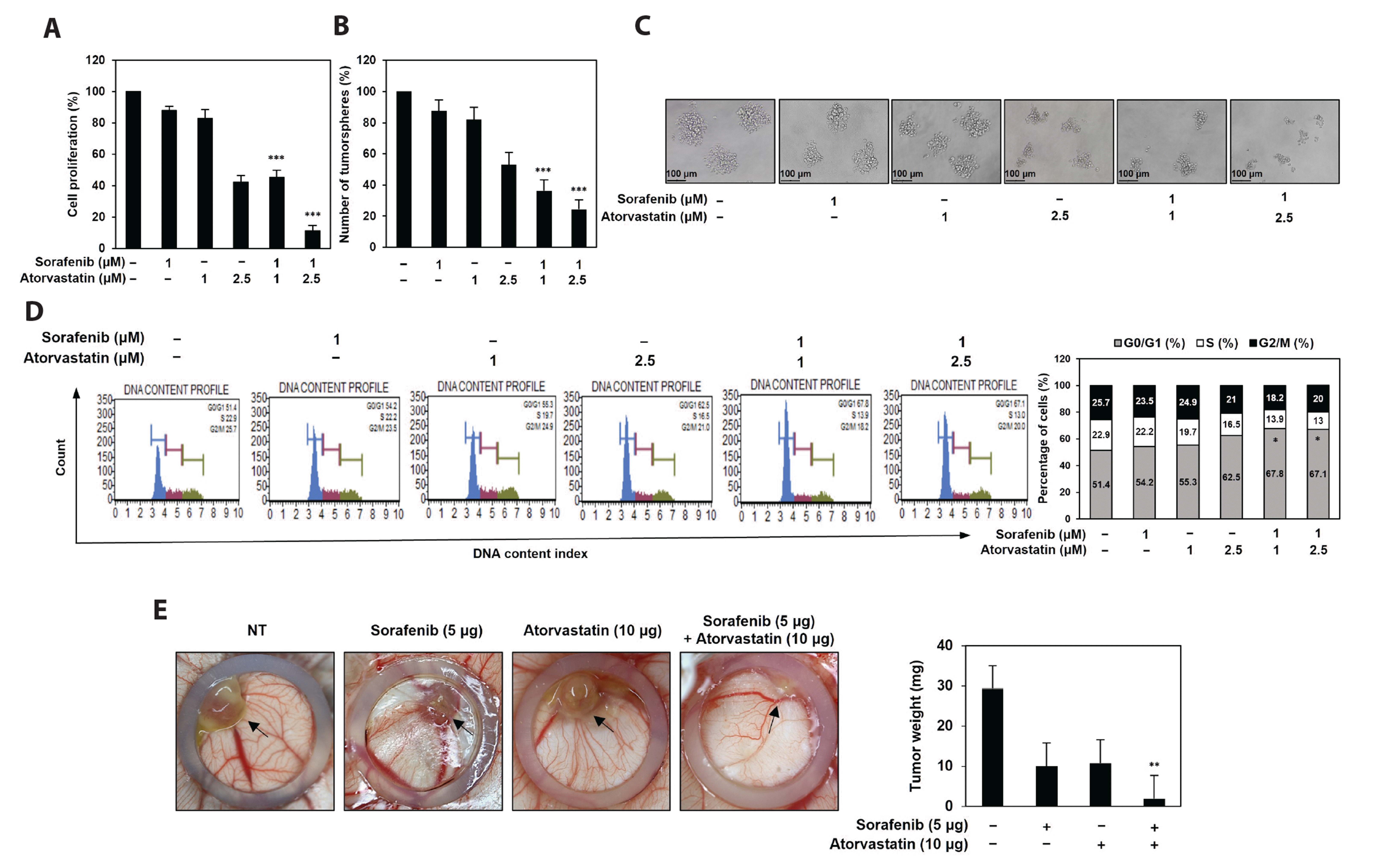