Abstract
Abdominal aortic aneurysm (AAA) is a life-threatening disorder worldwide. Fibroblast growth factor 21 (FGF21) was shown to display a high level in the plasma of patients with AAA; however, its detailed functions underlying AAA pathogenesis are unclear. An in vitro AAA model was established in human aortic vascular smooth muscle cells (HASMCs) by angiotensin II (Ang-II) stimulation. Cell counting kit-8, wound healing, and Transwell assays were utilized for measuring cell proliferation and migration. RT-qPCR was used for detecting mRNA expression of FGF21 and activating transcription factor 4 (ATF4). Western blotting was utilized for assessing protein levels of FGF21, ATF4, and markers for the contractile phenotype of HASMCs. ChIP and luciferase reporter assays were implemented for identifying the binding relation between AFT4 and FGF21 promoters. FGF21 and ATF4 were both upregulated in Ang-II-treated HASMCs. Knocking down FGF21 attenuated Ang-II-induced proliferation, migration, and phenotype switch of HASMCs. ATF4 activated FGF21 transcription by binding to its promoter. FGF21 overexpression reversed AFT4 silencing-mediated inhibition of cell proliferation, migration, and phenotype switch. ATF4 transcriptionally upregulates FGF21 to promote the proliferation, migration, and phenotype switch of Ang-II-treated HASMCs.
Fibroblast growth factor 21 (FGF21) is a secreted endocrine factor that acts as a pivotal regulator of metabolic function [1]. FGF21 has been indicated to be implicated in a diversity of biological processes, such as autophagy and apoptosis [2,3]. FGF21 alleviates endoplasmic reticulum stress-induced apoptosis to mitigate vascular calcification in rats [4]. FGF21 attenuates high-fat diet-mediated endothelial dysfunction by upregulating antioxidant genes in apolipoprotein E-deficient mice [5]. Moreover, FGF21 ameliorates hypertension and vascular dysfunction induced by angiotensin II (Ang-II) in mice by activating angiotensin-converting enzyme 2/angiotensin-(1-7) axis [6]. The evidence indicates that FGF21 exerts a critical role in vascular diseases. Importantly, it was shown that FGF21 displayed a high level in the plasma of patients with abdominal aortic aneurysm (AAA) in comparison to that in the control groups and FGF21 high expression was closely associated with clinical characteristics of AAA [7]. Nevertheless, the detailed function of FGF21 in AAA is unclear.
Activating transcription factor 4 (ATF4) is a multifunctional transcription regulatory protein involved in diverse biological processes in response to various stimuli [8]. It has been illustrated that metformin can induce FGF21 upregulation in an ATF4-dependent manner [9]. Moreover, FGF21 promoter has three response elements for ATF4, indicating that ATF4 may be implicated in regulating FGF21 expression [10]. Additionally, ATF4 was shown to be upregulated in a mouse AAA model induced by Ang-II [11]. However, whether ATF4 can regulate FGF21 expression in AAA is unclarified.
Vascular smooth muscle cells (VSMCs) are the main inherent cells in the tunica media of abdominal wall, and it was reported that dysfunction of VSMCs contributed to the pathogenesis of AAA [12]. Ang-II, a vasoactive peptide, exerts a crucial effect on the pathophysiological development of AAA; Ang-II-induced AAA models have been used in many studies for investigating AAA [13-15].
Herein, we probed the functions of FGF21 in an Ang-II-induced in vitro AAA model in human aortic VSMCs (HASMCs). It was hypothesized that FGF21 might be regulated by ATF4 to affect proliferation, migration and phenotype switch of HASMCs. The results might provide a new clue for treating AAA.
Primary HASMCs were obtained from American Type Culture Collection (PCS-100-012; ATCC, Manassas, VA, USA) and incubated in Dulbecco’s Modified Eagle’s Medium (DMEM; Invitrogen, Carlsbad, CA, USA) containing 10% fetal bovine serum (FBS; Hyclone, Salt Lake City, UT, USA) and 1% penicillin-streptomycin (Invitrogen) at 37°C in a humidified incubator with 5% CO2. Cells at passage 3–5 were used for subsequent experiments. Cells were treated with different concentrations of Ang-II (0–100 nM; Sigma, St. Louis, MO, USA) for 24 h. In some experiments, HASMCs were stimulated with 100 nM Ang-II for 24 h to mimic AAA in vitro.
Total RNA was isolated from HASMCs using TRIzol reagent (Thermo Scientific, Waltham, MA, USA). Approximately 1 μg of total RNA was reverse transcribed with M-MLV-reverse transferase using the PrimeScript RT Reagent Kit (Takara, Dalian, China) to obtain cDNA. RT-qPCR was implemented with 2X Power SYBR-Green PCR Master Mix (Applied Biosystems, Foster City, CA, USA) on a CFX96TM Real‐Time PCR Detection System (Bio‐Rad, Hercules, CA, USA). Relative expression of FGF21 and ATF4 was computed using the 2–ΔΔCt method and normalized to GAPDH. Primer sequences are listed as follows:
FGF21
Forward: 5’-GTCAAGACATCCAGGTTCC-3’
Reverse: 5’-AGTGGAGCGATCCATACAG-3’
ATF4
Forward: 5’-CAAGGAGGATGCCTTCTCC-3’
Reverse: 5’-GTCGAACTCCTTCAAATCCA-3’
GAPDH
Forward: 5’-TCAAGATCATCAGCAATGCC-3’
Reverse: 5’-CGATACCAAAGTTGTCATGGA-3’
Proteins were extracted from HASMCs using RIPA buffer (Beyotime, Shanghai, China) and quantified with a BCA assay kit (Bio-Rad). Equal amounts of protein samples (20 μg) were resolved by 10% SDS-PAGE and transferred onto polyvinylidene difluoride membranes (Thermo Scientific). After blocked with 5% defatted milk, the membranes were incubated at 4°C overnight with primary antibodies against: FGF21 (ab171941, 1:1,000), ATF4 (ab184909, 1:1,000), α-SMA (ab124964, 1:10,000), SM22α (ab155272, 1:1,000), Tubulin (ab176560, 1:2,000) (all from Abcam, Cambridge, MA, USA), followed by incubation with the secondary antibody (ab96899; Abcam). Eventually, protein bands were visualized with an enhanced chemiluminescence kit (Fdbio Science, Hangzhou, China) and quantified with ImageJ software (GE Healthcare, Beijing, China).
To knock down FGF21 or ATF4, small interference RNA targeting FGF21 (si-FGF21) or ATF4 (si-ATF4) and corresponding negative controls (si-NC) were transfected into HASMCs. PcDNA3.1/FGF21 and empty pcDNA3.1 vector were transfected into HASMCs for overexpressing FGF21. All plasmids were obtained from GenePharma (Shanghai, China). Cell transfection was implemented using Lipofectamine 2000 (Invitrogen). After 48 h, the transfection efficiency was detected by RT-qPCR.
HASMCs with indicated treatment were inoculated in 96-well plates (2 × 105 cells/well) and incubated for 24 h. Then, CCK-8 solution (10 μl; Dojindo, Kumamoto, Japan) was added to each well and cultured for another 2 h at 37°C. The optical density was measured at 450 nm using a microplate reader (BMG Labtech, Offenburg, Germany).
Indicated HASMCs were inoculated in 6-well plates (1 × 105 cells/well) until 80% confluence. Afterwards, a sterile 10 μl plastic pipette tip was utilized to scratch the plates and cells were further cultured for 24 h. Image-Pro Plus 6.0 software (Media Cybernetics, Shanghai, China) was used to record cell movement at 0 and 24 h. Cell migratory capability was measured as: (original scratch width-current scratch width) / original scratch width.
Cell migration was also examined using Transwell chambers (8 μm; Corning Inc., Corning, NY, USA). HASMCs (5 × 104 cells) suspended in 100 μl serum-free DMEM were added into the upper chambers and DMEM (500 μl) containing 10% FBS was placed into the lower chambers. After treatment for 24 h, cotton swabs were utilized to gently wiped off the nonmigratory cells. The migratory cells were fixed in 4% formaldehyde, stained with 0.1% crystal violet solution and counted under an Eclipse Ti-s microscope (Olympus, Tokyo, Japan).
ChIP assay was implemented using EpiQuik Chromatin immunoprecipitation Assay Kit (AmyJet, Wuhan, China) following the manufacturer’s protocols. Briefly, HASMCs were fixed with 1% formaldehyde and then treated with glycine for 5 mins. Afterwards, the chromatin was sonicated into DNA fragments (200–400 bp), and anti-ATF4 and control anti-IgG (Abcam) were used to precipitate the chromatin. Then quantification of the precipitated DNA was performed using RT-qPCR.
ATF4 binding site in FGF21 promoter sequence was predicted by JASPAR website (https://jaspar.genereg.net/). Wild type (Wt) FGF21 promoter sequence containing ATF4 binding site and the mutant (Mut) sequence were separately subcloned into pGL3 vector (Promega Corporation, Fitchburg, WI, USA) to establish Wt or Mut pGL3-FGF21 promoter. Then these vectors were co-transfected with si-ATF4 or si-NC into HASMCs using Lipofectamine 3000 (Invitrogen). The luciferase activity was assessed with Dual-Luciferase Reporter Assay System (Promega Corporation) 48 h post-transfection.
Data are presented as the mean ± standard deviation. SPSS 21.0 software (IBM Co., Armonk, NY, USA) was used for statistical analysis. Differences between two groups were analyzed with Student’s t-test, while those among more groups were evaluated using analysis of variance (ANOVA) followed by Turkey’s post-hoc analysis. Each experiment was implemented in triplicate. p ˂ 0.05 was considered as statistically significant.
HASMCs were treated with different concentrations of Ang-II to mimic the in vitro AAA model. The impact of Ang-II on cell proliferation was examined by CCK-8 assay. As displayed by the results, Ang-II induced HASMC proliferation in a dose-dependent manner and cell proliferation was shown to be significantly increased when the concentration of Ang-II reached 10 nM (Fig. 1A). We then detected FGF21 expression in HASMCs exposed to different concentrations of Ang-II. Notably, FGF21 expression at mRNA and protein levels was elevated as the concentration of Ang-II increased (Fig. 1B–D).
To probe the potential role of FGF21 in the in vitro AAA model, FGF21 was knocked down in HASMCs stimulated with 100 nM Ang-II for 24 h. The efficiency of FGF21 knockdown was confirmed by RT-qPCR and western blotting (Fig. 2A–C). Then, the proliferation, migration and phenotype switch of HASMCs were analyzed. As shown in Fig. 2D, knocking down FGF21 markedly abated Ang-II-induced increase in HASMC proliferation. Results from wound healing assay displayed that FGF21 silencing counteracted Ang-II-induced HASMC migration (Fig. 2E, F). This was further confirmed by Transwell assay (Fig. 2E, G). Moreover, we detected the protein levels of SM22α and α-SMA, the markers for contractile phenotype of HASMCs. Notably, Ang-II caused the downregulation of SM22α and α-SMA in HASMCs, while this effect was partially reversed by FGF21 depletion (Fig. 2H). Collectively, FGF21 silencing can restrain Ang-II-induced proliferation, migration and phenotype switch of HASMCs.
Subsequently, we explored the potential mechanism responsible for FGF21 upregulation in Ang-II-stimulated HASMCs. It was reported that FGF21 expression could be regulated by the transcription factor ATF4 [10]. Here, we tested whether ATF4 was involved in regulating FGF21 expression in HASMCs. As displayed in Fig. 3A–C, Ang-II dose-dependently upregulated AFT4 in HASMCs. Moreover, we searched JASPAR database using FGF21 promoter sequence and a ATF4-binding site in FGF21 promoter sequence was predicted (Fig. 3D, E). ChIP assay elucidated the binding relation between ATF4 and FGF21 promoter (Fig. 3F). Then, we knocked down ATF4 in HASMCs with si-ATF4. As shown by western blotting, knocking down ATF4 not only decreased ATF4 protein expression, but also caused the downregulation of FGF21 protein expression in HASMCs (Fig. 3G, H). Additionally, ATF4 silencing decreased the luciferase activity of Wt FGF21 promoter but had no significant impact on the luciferase activity after mutation (Fig. 3I), further confirming the binding relation between AFT4 and FGF21 promoter. The above results indicate that ATF4 activates FGF21 transcription in HASMCs.
To further elucidate the role of ATF4/FGF21 axis in AAA in vitro, si-ATF4 and FGF21 overexpression vector were co-transfected into HASMCs before treatment of Ang-II. The efficiency of FGF21 overexpression was confirmed by RT-qPCR as well as western blotting (Fig. 4A–C). CCK-8 assay displayed that silencing ATF4 suppressed cell proliferation, while overexpression FGF21 reversed this effect induced by si-ATF4 (Fig. 4D). Wound healing and Transwell assays both revealed that FGF21 overexpression offset ATF4 knockdown-induced inhibition of cell migratory capability (Fig. 4E–G). Moreover, protein levels of SM22α and α-SMA were increased in si-ATF4-transfected group, while the levels were decreased in the group simultaneously transfected with si-ATF4 and FGF21 overexpression vector (Fig. 4H), indicating that FGF21 promoted phenotype switch of HASMCs. Hence, the above data reveal that ATF4 facilitates HASMC proliferation, migration and phenotype switch by activating FGF21 transcription. A schematic view of the investigated model was shown in Fig. 5.
AAA is a vascular degenerative disorder defined as irreversible dilation of abdominal aorta that occurs mostly in elderly men [16]. Patients with AAA are usually asymptomatic, but once a rupture occurs, the patient may suffer from severe abdominal pain, low blood pressure and hemorrhage and have a high risk of death [17]. It is estimated that the mortality rate is up to 90% upon AAA rupture [18]. Open and endovascular aneurysm repair surgery are the main therapeutic strategies for AAA; however, they are not applicable for small AAA (<rmsep 55 mm in diameter) [19]. Additionally, there is currently no effective pharmacotherapy available for AAA treatment [20]. These mean that patients who are not suitable for surgery or have small AAA have no active treatment options. Hence, better understanding of AAA pathogenesis and finding more effective approaches for AAA therapy are urgently needed.
VSMCs are the principal component of the tunica media of the aortic wall and exert their function in the maintenance of vascular tone [21]. Emerging evidence has demonstrated that dysfunction of VSMCs, including excessive proliferation and migration and phenotype switch, contributes to the progression of vascular diseases, including AAA [22]. The fully differentiated VSMCs show a contractile phenotype and in response to vascular injury, VSMCs switch from a contractile state to a synthetic phenotype, characterized by elevated proliferative and migrative capabilities and decreased levels of contractile markers [23]. Here we established an AAA model in HASMCs induced by Ang-II and probed the potential role of FGF21 in Ang-II-stimulated HASMCs.
FGF21, a key regulator of metabolism, has been indicated to exert significant effects on the development of multiple vascular disorders. For example, in pulmonary arterial hypertension, FGF21 alleviates endothelial dysfunction and inflammation induced by hypoxia by regulating miR-27b mediated PPARγ pathway [24]. FGF21 protects against atherosclerosis by inhibiting vascular endothelial cell pyroptosis mediated by NLRP3 inflammasome [25]. Importantly, a high level of FGF21 in the plasma of patients with AAA was observed and FGF21 expression was positively correlated with AAA diameters and hypertension rate [7]. This indicated a close correlation between FGF21 the disease progression. Consistent with the previous study mentioned above, we found in this study that Ang-II dose-dependently induced FGF21 expression in HASMCs. Then, loss-of-function assays were implemented to examine the role of FGF21 in regulating HASCM proliferation, migration and phenotype switch. The results displayed that FGF21 silencing significantly inhibited the proliferative and migratory capabilities as well as phenotype switch of HASMCs, indicating that FGF21 might exert a promoting effect on the progression of AAA.
To explore the upregulation mechanism of FGF21 in Ang-II-treated HASMCs, bioinformatics analysis and a series of assays were performed. It was found that ATF4 was upregulated as the concentration of Ang-II increased in HASMCs and ATF4 activated FGF21 transcription by binding to its promoter. These results were in accord with previous studies which showed that FGF21 promoter has three response elements for ATF4 and ATF4 can induce FGF21 upregulation [9,10]. ATF4 is a widely expressed mammalian DNA binding protein that is implicated in a variety of pathological and physiological processes, such as autophagy, drug resistance and apoptosis [26,27]. It has been shown that ATF4 upregulation can induce VSMC to proliferate [28]. ATF4 is involved in vascular injury through the activation of a signaling pathway involving PERK, eIF2α and CHOP, key molecules in endoplasmic reticulum stress [29]. Additionally, it was demonstrated that ATF4 displayed an increased level in Ang-II-treated murine aortas [11]. To further verify the role of ATF4/FGF21 axis in regulating the behaviors of Ang-II-induced HASMCs, rescue experiments were conducted. The results revealed that knocking down ATF4 markedly restrained the proliferation and migration and decreased expression of markers for the contractile phenotype of HASMCs. However, these effects were abated by FGF21 overexpression, indicating that ATF4/FGF21 axis contributed to the pathogenesis of AAA.
In conclusion, we probed the role of FGF21 in the Ang-II-induced AAA model in HASMCs. The results reveal that ATF4 transcriptionally upregulates FGF21 to promote the proliferation, migration and phenotype switch of Ang-II-treated HASMCs. Our findings might provide a novel therapeutic strategy for AAA.
Notes
REFERENCES
1. Mason BL, Minhajuddin A, Czysz AH, Jha MK, Gadad BS, Mayes TL, Trivedi MH. 2022; Fibroblast growth factor 21 (FGF21) is increased in MDD and interacts with body mass index (BMI) to affect depression trajectory. Transl Psychiatry. 12:16. DOI: 10.1038/s41398-021-01679-y. PMID: 35017468. PMCID: PMC8752780. PMID: 741a0a0f9d014ac49f40f05279d94e70.


2. Kakoty V, K C S, Tang RD, Yang CH, Dubey SK, Taliyan R. 2020; Fibroblast growth factor 21 and autophagy: a complex interplay in Parkinson disease. Biomed Pharmacother. 127:110145. DOI: 10.1016/j.biopha.2020.110145. PMID: 32361164.


3. Lu H, Jia C, Wu D, Jin H, Lin Z, Pan J, Li X, Wang W. 2021; Fibroblast growth factor 21 (FGF21) alleviates senescence, apoptosis, and extracellular matrix degradation in osteoarthritis via the SIRT1-mTOR signaling pathway. Cell Death Dis. 12:865. DOI: 10.1038/s41419-021-04157-x. PMID: 34556628. PMCID: PMC8460788. PMID: 78876a2127d747b0a53e5dd0597c8086.


4. Shi Y, Wang S, Peng H, Lv Y, Li W, Cheng S, Liu J. 2019; Fibroblast growth factor 21 attenuates vascular calcification by alleviating endoplasmic reticulum stress mediated apoptosis in rats. Int J Biol Sci. 15:138–147. DOI: 10.7150/ijbs.28873. PMID: 30662354. PMCID: PMC6329919.


5. Huang WP, Chen CY, Lin TW, Kuo CS, Huang HL, Huang PH, Lin SJ. 2022; Fibroblast growth factor 21 reverses high-fat diet-induced impairment of vascular function via the anti-oxidative pathway in ApoE knockout mice. J Cell Mol Med. 26:2451–2461. DOI: 10.1111/jcmm.17273. PMID: 35307922. PMCID: PMC8995458.


6. Pan X, Shao Y, Wu F, Wang Y, Xiong R, Zheng J, Tian H, Wang B, Wang Y, Zhang Y, Han Z, Qu A, Xu H, Lu A, Yang T, Li X, Xu A, Du J, Lin Z. 2018; FGF21 prevents angiotensin II-induced hypertension and vascular dysfunction by activation of ACE2/angiotensin-(1-7) axis in mice. Cell Metab. 27:1323–1337.e5. DOI: 10.1016/j.cmet.2018.04.002. PMID: 29706566.


7. Xie T, Yin L, Guo D, Zhang Z, Chen Y, Liu B, Wang W, Zheng Y. 2021; The potential role of plasma fibroblast growth factor 21 as a diagnostic biomarker for abdominal aortic aneurysm presence and development. Life Sci. 274:119346. DOI: 10.1016/j.lfs.2021.119346. PMID: 33713667.


8. Ebert SM, Rasmussen BB, Judge AR, Judge SM, Larsson L, Wek RC, Anthony TG, Marcotte GR, Miller MJ, Yorek MA, Vella A, Volpi E, Stern JI, Strub MD, Ryan Z, Talley JJ, Adams CM. 2022; Biology of activating transcription factor 4 (ATF4) and its role in skeletal muscle atrophy. J Nutr. 152:926–938. DOI: 10.1093/jn/nxab440. PMID: 34958390. PMCID: PMC8970988.


9. Kim KH, Jeong YT, Kim SH, Jung HS, Park KS, Lee HY, Lee MS. 2013; Metformin-induced inhibition of the mitochondrial respiratory chain increases FGF21 expression via ATF4 activation. Biochem Biophys Res Commun. 440:76–81. DOI: 10.1016/j.bbrc.2013.09.026. PMID: 24041694.


10. Maruyama R, Shimizu M, Li J, Inoue J, Sato R. 2016; Fibroblast growth factor 21 induction by activating transcription factor 4 is regulated through three amino acid response elements in its promoter region. Biosci Biotechnol Biochem. 80:929–934. DOI: 10.1080/09168451.2015.1135045. PMID: 27010621.


11. Ni XQ, Lu WW, Zhang JS, Zhu Q, Ren JL, Yu YR, Liu XY, Wang XJ, Han M, Jing Q, Du J, Tang CS, Qi YF. 2018; Inhibition of endoplasmic reticulum stress by intermedin1-53 attenuates angiotensin II-induced abdominal aortic aneurysm in ApoE KO Mice. Endocrine. 62:90–106. DOI: 10.1007/s12020-018-1657-6. PMID: 29943223.


12. Zhou Z, Zhou H, Zou X, Wang X. 2022; RUNX3 is up-regulated in abdominal aortic aneurysm and regulates the function of vascular smooth muscle cells by regulating TGF-β1. J Mol Histol. 53:1–11. DOI: 10.1007/s10735-021-10035-9. PMID: 34813022.


13. Liu X, Chen X, Xu C, Lou J, Weng Y, Tang L. 2022; Platelet protects angiotensin II-driven abdominal aortic aneurysm formation through inhibition of inflammation. Exp Gerontol. 159:111703. DOI: 10.1016/j.exger.2022.111703. PMID: 35038567.


14. Gao P, Zhang H, Zhang Q, Fang X, Wu H, Wang M, Lu Z, Wei X, Yang G, Yan Z, Liu D, Zhu Z. 2019; Caloric restriction exacerbates angiotensin II-induced abdominal aortic aneurysm in the absence of p53. Hypertension. 73:547–560. DOI: 10.1161/HYPERTENSIONAHA.118.12086. PMID: 30686087.


15. Ma M, Yang X, Han F, Wang H. 2022; Circ_0092291 attenuates angiotensin II-induced cell damages in human aortic vascular smooth muscle cells via mediating the miR-626/COL4A1 signal axis. J Physiol Biochem. 78:245–256. DOI: 10.1007/s13105-021-00859-0. PMID: 34997455.


16. Chiang MT, Chen IM, Hsu FF, Chen YH, Tsai MS, Hsu YW, Leu HB, Huang PH, Chen JW, Liu FT, Chen YH, Chau LY. 2021; Gal-1 (galectin-1) upregulation contributes to abdominal aortic aneurysm progression by enhancing vascular inflammation. Arterioscler Thromb Vasc Biol. 41:331–345. DOI: 10.1161/ATVBAHA.120.315398. PMID: 33147994.


17. Horimatsu T, Blomkalns AL, Ogbi M, Moses M, Kim D, Patel S, Gilreath N, Reid L, Benson TW, Pye J, Ahmadieh S, Thompson A, Robbins N, Mann A, Edgell A, Benjamin S, Stansfield BK, Huo Y, Fulton DJ, Agarwal G, et al. 2020; Niacin protects against abdominal aortic aneurysm formation via GPR109A independent mechanisms: role of NAD+/nicotinamide. Cardiovasc Res. 116:2226–2238. DOI: 10.1093/cvr/cvz303. PMID: 31710686. PMCID: PMC7695356.


18. Doyle BJ, Bappoo N, Syed MBJ, Forsythe RO, Powell JT, Conlisk N, Hoskins PR, McBride OMB, Shah ASV, Norman PE, Newby DE. 2020; Biomechanical assessment predicts aneurysm related events in patients with abdominal aortic aneurysm. Eur J Vasc Endovasc Surg. 60:365–373. DOI: 10.1016/j.ejvs.2020.02.023. PMID: 32253165.


19. Bernal S, Lopez-Sanz L, Jimenez-Castilla L, Prieto I, Melgar A, La Manna S, Martin-Ventura JL, Blanco-Colio LM, Egido J, Gomez-Guerrero C. 2021; Protective effect of suppressor of cytokine signalling 1-based therapy in experimental abdominal aortic aneurysm. Br J Pharmacol. 178:564–581. DOI: 10.1111/bph.15330. PMID: 33227156.


20. Memon AA, Zarrouk M, Ågren-Witteschus S, Sundquist J, Gottsäter A, Sundquist K. 2020; Identification of novel diagnostic and prognostic biomarkers for abdominal aortic aneurysm. Eur J Prev Cardiol. 27:132–142. DOI: 10.1177/2047487319873062. PMID: 31466471.


21. Riches-Suman K, Hussain A. 2022; Identifying and targeting the molecular signature of smooth muscle cells undergoing early vascular ageing. Biochim Biophys Acta Mol Basis Dis. 1868:166403. DOI: 10.1016/j.bbadis.2022.166403. PMID: 35367337.


22. Hsu CY, Vo TTT, Lee CW, Chen YL, Lin WN, Cheng HC, Vo QC, Lee IT. 2022; Carbon monoxide releasing molecule-2 attenuates angiotensin II-induced IL-6/Jak2/Stat3-associated inflammation by inhibiting NADPH oxidase- and mitochondria-derived ROS in human aortic smooth muscle cells. Biochem Pharmacol. 198:114978. DOI: 10.1016/j.bcp.2022.114978. PMID: 35218740.


23. Jiang B, Wang M, Li X, Ren P, Li G, Wang Y, Wang L, Li X, Yang D, Qin L, Xin S. 2022; Hexarelin attenuates abdominal aortic aneurysm formation by inhibiting SMC phenotype switch and inflammasome activation. Microvasc Res. 140:104280. DOI: 10.1016/j.mvr.2021.104280. PMID: 34856183.


24. Yao D, He Q, Sun J, Cai L, Wei J, Cai G, Liu J, Lin Y, Wang L, Huang X. 2021; FGF21 attenuates hypoxia-induced dysfunction and inflammation in HPAECs via the microRNA-27b-mediated PPARγ pathway. Int J Mol Med. 47:116. DOI: 10.3892/ijmm.2021.4949. PMID: 33907846. PMCID: PMC8083827.


25. Zeng Z, Zheng Q, Chen J, Tan X, Li Q, Ding L, Zhang R, Lin X. 2020; FGF21 mitigates atherosclerosis via inhibition of NLRP3 inflammasome-mediated vascular endothelial cells pyroptosis. Exp Cell Res. 393:112108. DOI: 10.1016/j.yexcr.2020.112108. PMID: 32445748.


26. Yuan S, Liang X, He W, Liang M, Jin J, He Q. 2021; ATF4-dependent heme-oxygenase-1 attenuates diabetic nephropathy by inducing autophagy and inhibiting apoptosis in podocyte. Ren Fail. 43:968–979. DOI: 10.1080/0886022X.2021.1936040. PMID: 34157937. PMCID: PMC8231401.


27. Feng L, Li M, Hu X, Li Y, Zhu L, Chen M, Wei Q, Xu W, Zhou Q, Wang W, Chen D, Wang X, Jin H. 2021; CK1δ stimulates ubiquitination-dependent proteasomal degradation of ATF4 to promote chemoresistance in gastric Cancer. Clin Transl Med. 11:e587. DOI: 10.1002/ctm2.587. PMID: 34709767. PMCID: PMC8516343. PMID: 0e859d4b9c61470e9f235edfc56ecf03.


28. Malabanan KP, Khachigian LM. 2010; Activation transcription factor-4 and the acute vascular response to injury. J Mol Med (Berl). 88:545–552. DOI: 10.1007/s00109-010-0615-4. PMID: 20306012.


29. He L, Yuan J, Xu Q, Chen R, Chen L, Fang M. 2016; miRNA-1283 regulates the PERK/ATF4 pathway in vascular injury by targeting ATF4. PLoS One. 11:e0159171. DOI: 10.1371/journal.pone.0159171. PMID: 27537404. PMCID: PMC4990291.


Fig. 1
Ang-II induced cell proliferation increase and FGF21 upregulation.
(A) CCK-8 assay for assessing the proliferation of HASMCs under treatment of different concentrations of Ang-II (0-100 nM). (B–D) RT-qPCR analysis and western blotting of FGF21 mRNA and protein expression levels in HASMCs treated with different concentrations of Ang-II (0–100 nM), respectively. Ang-II, angiotensin II; FGF21, fibroblast growth factor 21; CCK-8, cell counting kit-8; HASMCs, human aortic vascular smooth muscle cells; RT-qPCR, real time quantitative polymerase chain reaction. *p < 0.05, **p < 0.01.
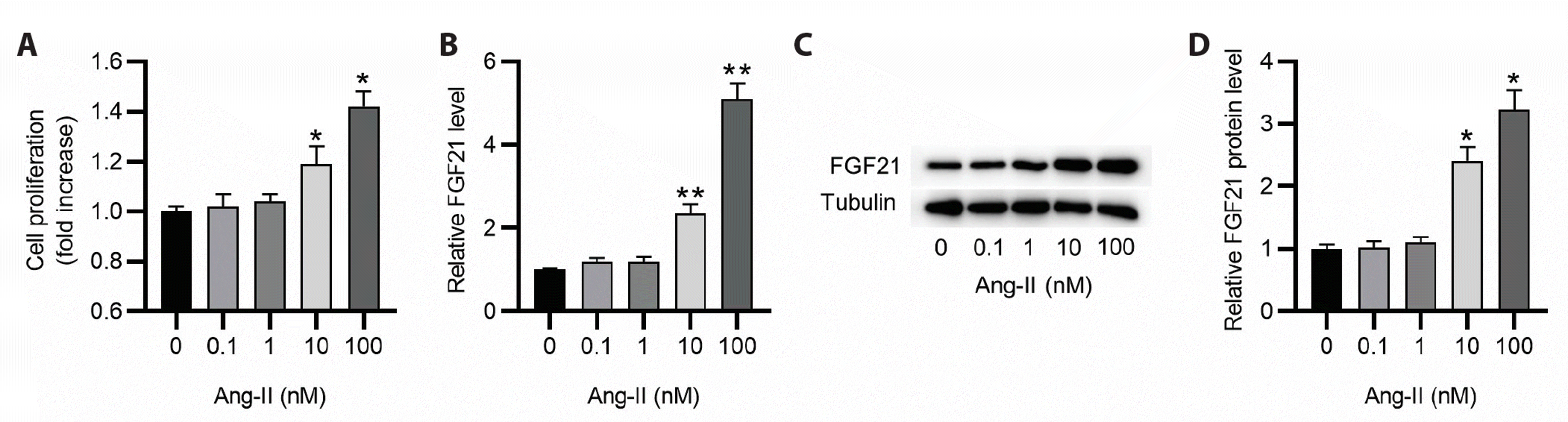
Fig. 2
FGF21 silencing inhibits HASMC proliferation, migration and phenotype switch.
HASMCs were transfected with si-FGF21 or si-NC for 48 h before treatment of 100 nM Ang-II for 24 h. (A–C) RT-qPCR and western blotting for evaluating the efficiency of FGF21 knockdown in HASMCs. (D) CCK-8 assay for assessing cell proliferation in each group. (E–G) Wound healing and Transwell assays for examining cell migratory ability. Magnification for wound healing: ×40; magnification for Transwell: ×200. (H) Western blotting of SM22α and α-SMA protein expression in HASMCs. FGF21, fibroblast growth factor 21; HASMCs, human aortic vascular smooth muscle cells; NC, negative control; Ang-II, angiotensin II; RT-qPCR, real time quantitative polymerase chain reaction; CCK-8, cell counting kit-8. **p < 0.01.

Fig. 3
ATF4 transcriptionally activates FGF21 in HASMCs.
(A–C) RT-qPCR and Western blotting of ATF4 expression in HASMCs treated with different concentrations (0–100 nM) of Ang-II. (D, E) JASPAR website for prediction of ATF4 binding site in FGF21 promoter region. (F) ChIP assay for identifying the binding relation between ATF4 and FGF21 promoter. (G, H) Western blotting of ATF4 and FGF21 protein expression in ATF4-depleted HASMCs. (I) Luciferase reporter assay for identifying the binding relation between ATF4 and FGF21 promoter. ATF4, activating transcription factor 4; FGF21, fibroblast growth factor 21; HASMCs, human aortic vascular smooth muscle cells; RT-qPCR, real time quantitative polymerase chain reaction; Ang-II, angiotensin II; TFBS, transcription factor binding site; TSS, transcription start site; ChIP, chromatin immunoprecipitation. *p < 0.05, **p < 0.01, ***p < 0.001.
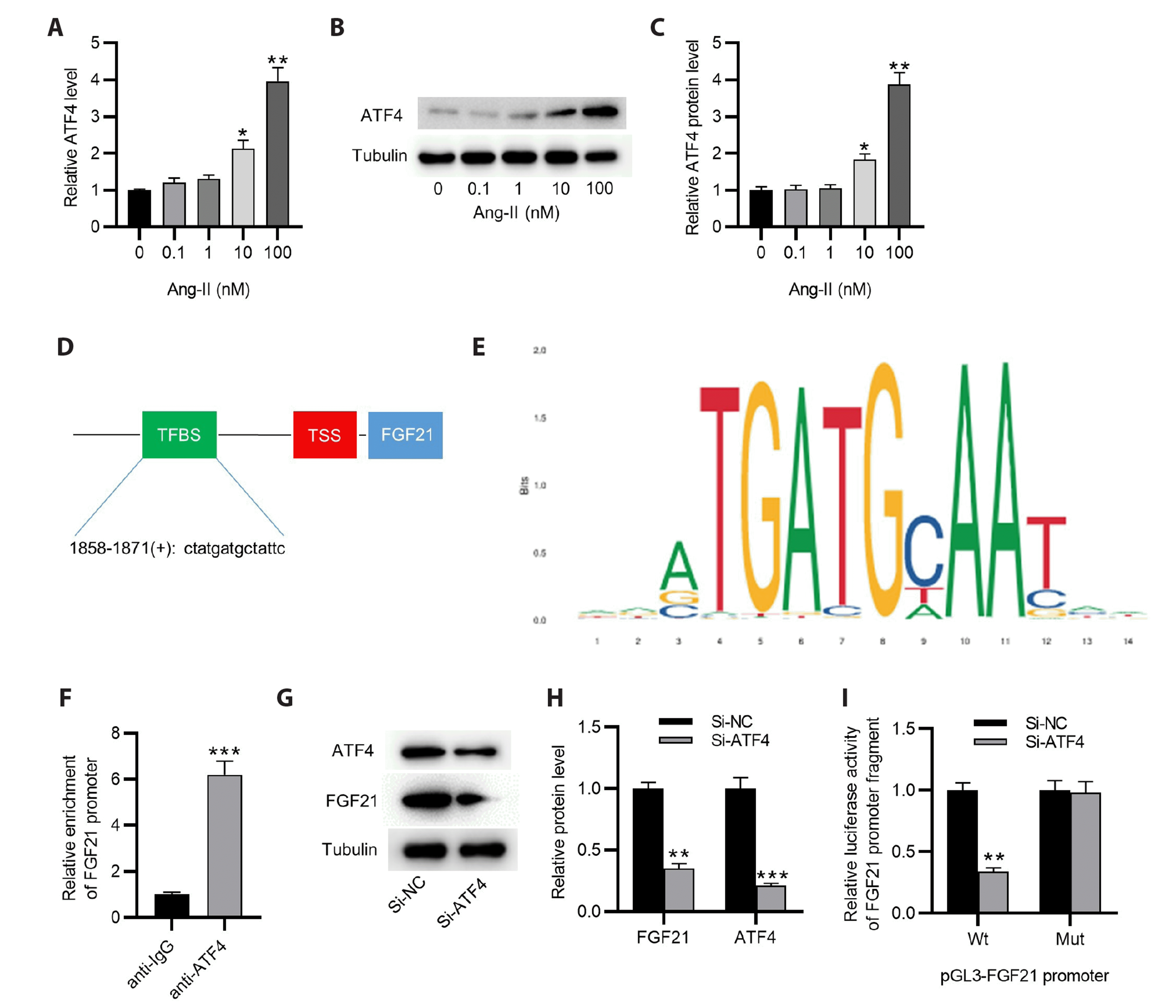
Fig. 4
ATF4 promotes proliferation, migration and phenotype switch of HASMCs by activating FGF21 transcription.
HASMCs were transfected with si-NC, si-ATF4 or si-ATF4 + pcDNA3.1/FGF21 for 48 h before treatment of 100 nM Ang-II for 24 h. (A–C) RT-qPCR analysis and western blotting for assessing the efficiency of FGF21 overexpression in HASMCs. (D) CCK-8 assay for evaluating cell proliferative ability in each group. (E–G) Wound healing and Transwell assays for assessing cell migration in each group. Magnification for wound healing: ×40; magnification for Transwell: × 200. (H) Western blotting of SM22α and α-SMA protein expression in HASMCs of each group. ATF4, activating transcription factor 4; HASMCs, human aortic vascular smooth muscle cells; FGF21, fibroblast growth factor 21; NC, negative control; Ang-II, angiotensin II; RT-qPCR, real time quantitative polymerase chain reaction; CCK-8, cell counting kit-8. *p < 0.05, **p < 0.01, , ***p < 0.001.

Fig. 5
A schematic view of the model investigated in this study.
ATF4 activates FGF21 transcription by binding to its promoter in the nuclei of HASMCs. Increased FGF21 mRNA levels leads to the upregulation of FGF21 protein expression, which promotes proliferation, migration and phenotype switch of HASMCs. ATF4, activating transcription factor 4; HASMCs, human aortic vascular smooth muscle cells; FGF21, fibroblast growth factor 21.
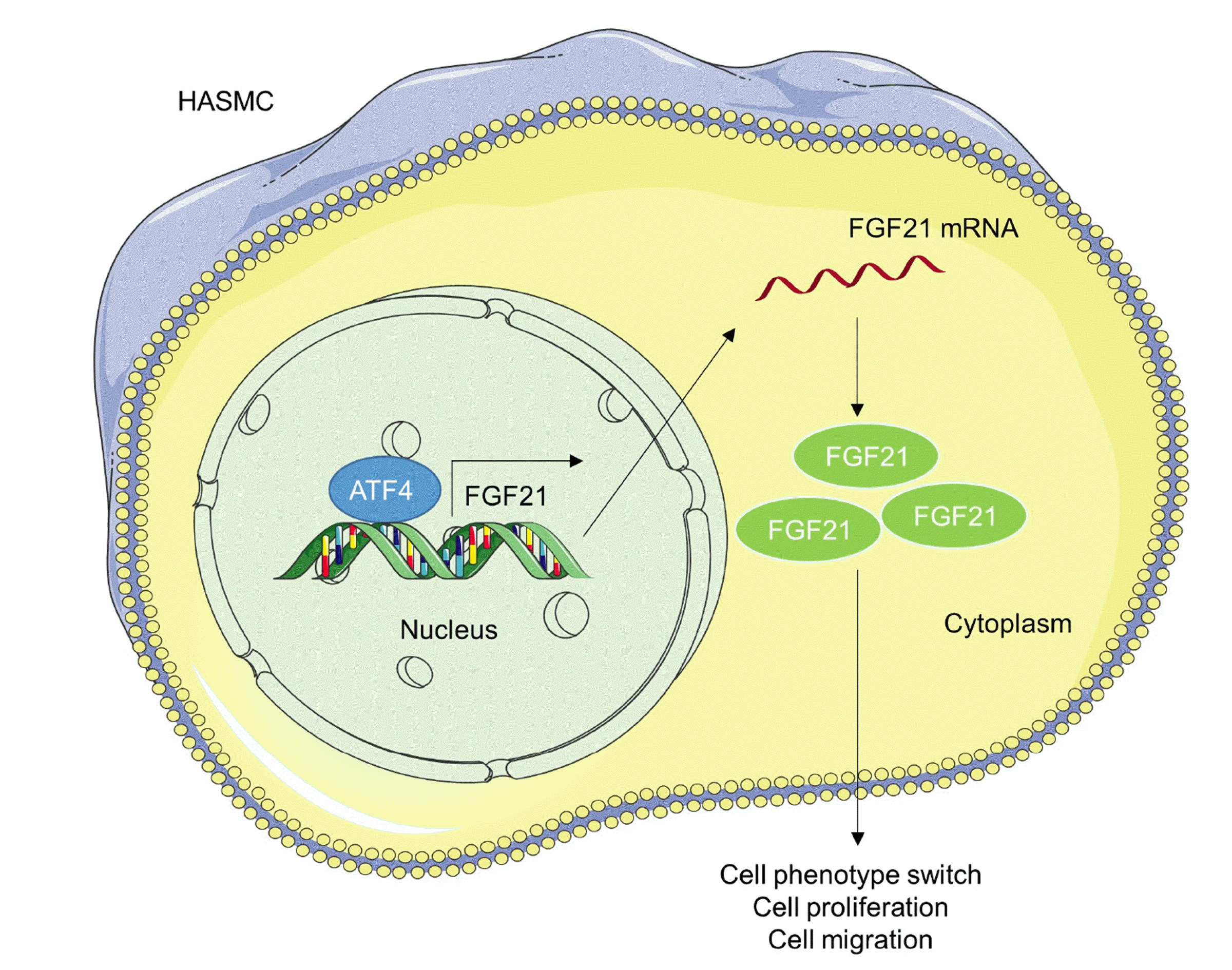