Abstract
Heart failure (HF) has become one of the severe public health problems. The detailed role of mitochondrial function in HF was still unclear. Benzoylaconine (BAC) is a traditional Chinese medicine, but its role in HF still needs to be explored. In this study, oxygen-glucose deprivation and reperfusion (OGD/R) was executed to mimic the injury of H9C2 cells in HF. The viability of H9C2 cells was assessed via MTT assay. OGD/R treatment markedly decreased the viability of H9C2 cells, but BAC treatment evidently increased the viability of OGD/R-treated H9C2 cells. The apoptosis of H9C2 was enhanced by OGD/R treatment but suppressed by BAC treatment. The mitochondrial membrane potential was evaluated via JC-1 assay. BAC improved the mitochondrial function and suppressed oxidative stress in OGD/R-treated H9C2 cells. Moreover, Western blot analysis revealed that the protein expression of p-AMPK and PGC-1α were reduced in OGD/R-treated H9C2 cells, which was reversed by BAC. Rescue assays indicated that AMPK attenuation reversed the BAC-mediated protective effect on OGD/R-treated cardiomyocytes. Moreover, BAC alleviated myocardial injury in vivo. In a word, BAC modulated the mitochondrial function in OGD/R-induced cardiomyocyte injury by activation of the AMPK/PGC-1 axis. The findings might provide support for the application of BAC in the treatment of HF.
Myocardial infarction is one of the major parts of cardiovascular diseases, which was associated with an elevated risk of disability and mortality [1]. Heart failure (HF) post-acute myocardial infarction has become one of the severe public health problems which results in increasing hospitalizations and deaths of people [2,3]. Although great progress has been made in the therapeutic strategies of HF, the outcome of these patients was still unfavorable [4]. In addition, the widely applied treatment method, such as cardiac reperfusion following acute myocardial ischemia can also cause cardiomyocyte injury [5]. Recently, the abnormal mitochondrial function was proposed to be implicated in the development of cardiomyocyte injury in HF [6]. Dysfunction of energy metabolism caused by mitochondrial disorders might be closely associated with cardiomyocyte injury in HF [7]. Alleviating the damage of mitochondrial function has become an intriguing therapeutic method for reducing cardiomyocyte injury in HF [8]. Nonetheless, the detailed mechanisms of mitochondrial function regulating HF remain elusive.
As a typical monoester alkaloid, benzoylaconine (BAC) is the primary bioactive compounds in Fuzi [9]. BAC has been widely accepted to have the pharmacological efficacy and a potential anti-inflammatory effect [10]. In former studies, some researchers have found that BAC exerted biological activities and participated in some biological processes. For instance, BAC increased the cell viability and oxygen consumption rate and induced mitochondrial biogenesis in mice through the activation of AMPK signaling [11]. BAC regulated lipopolysaccharide-triggered RAW264.7 cell responses via modulating the toll-like receptor-mediated NF-κB and MAPK signaling pathways [12]. BAC exerted biological activities and pharmacological effect in rats [13]. Nevertheless, the role of BAC in regulating the mitochondrial function of HF still needs to be elucidated.
In the current study, the function of BAC in regulating mitochondrial function in oxygen-glucose deprivation and reperfusion (OGD/R)-treated cardiomyocytes was assessed. The results uncovered that BAC improved the mitochondrial function in OGD/R-induced cardiomyocyte injury through activating the AMPK/PGC-1 signaling pathway. The findings of this study might shed a light on the application of BAC in the treatment of HF in the future.
Sprague–Dawley rats (male, 6–8 weeks, 200–250 g) were acquired from Vital River Biological Co., Ltd, Beijing, China. The animal experiment was approved by the Animal Care and Use Committee of Hebi People’s Hospital (approval no. 2020-158). All rats were kept in the standard cages with free foods and water on a 12 h light-dark cycle. To make the IR rat model, the left anterior descending (LAD) coronary artery was exposed. A 7-0 nylon stitch was maintained at the top edge of the left auricula (near the LAD). The nontraumatic occluder was put above the artery to stimulate myocardial infarction. The IR model was induced by inflating the occluder for 30 min and then performing reperfusion for 3 h. Rats in the Sham group were done by thoracotomy without LAD ligation. Rats (n = 18) were randomly divided into 3 groups: the Sham group (n = 6), the IR group (n = 6) and the IR + 20 mg/kg BAC group (n = 6).
For TTC staining, the heart samples were cut into 1-mm sections. The sections were stained with TTC solution (Sigma-Aldrich, St. Louis, MO, USA) for 15 min. The dyed sections were imaged and analyzed using ImageJ software (National Institutes of Health, Bethesda, MD, USA). The heart infarct size (%) was calculated as following formula: The heart infarct size (%) = the infarction area / the total area × 100%.
The LDH level was examined through a commercial LDH assay kit (ab65393; Abcam, Cambridge, MA, USA). The ROS level was determined by a commercial ROS Detection Cell-Based Assay Kit (Cayman Chemical, Ann Arbor, MI, USA) in line with the manufacturer’s guidelines.
Rat cardiomyocytes (H9C2 cells) were obtained from American Type Culture Collection (ATCC, Manassas, VA, USA) and maintained in Dulbecco’s modified Eagle medium (DMEM; Invitrogen, Carlsbad, CA, USA) with additional GlutaMAX (2 μM; Invitrogen) and fetal bovine serum (FBS, 10%; Invitrogen). H9C2 cells were grown in the incubator with 5% CO2 at 37°C.
OGD/R was executed to mimic the injury of H9C2 cells in HF. OGD was induced through culturing H9C2 cells in glucose-free DMEM including sodium dithionite (Na2S2O4; 5 μM) for 2 h. The complete medium was used in the supernatant for 6 h for reoxygenation.
The viability of H9C2 cells was evaluated via MTT assay. H9C2 cells were planted into 96-well plates. MTT regents (5 mg/ml; Sigma-Aldrich) was supplemented in the culture medium and cells were cultured at 37°C for 4 h in the darkness at 37°C for 4 h. To dissolve the formazan of MTT, dimethyl sulfoxide was then added. The absorbance at 490 nm was read to determine the viability of H9C2 cells.
The dye dihydroethidium (5 μM) (Abcam) was utilized to stain H9C2 cells for 10 min for measuring the ROS production in H9C2 cells. The images were randomly selected from ten regions of interest using a fluorescent microscope and the ImageJ software (National Institutes of Health) were employed for analyzing the fluorescence density of the stained H9C2 cells.
The mitochondrial membrane potential was tested via JC-1 assay. JC-1 dye (Beyotime, Haimen, China) was used to stain H9C2 cells for 20 min at 37°C and rinsed by PBS. The confocal laser scanning microscope (Leica, Heidelberg, Germany) was applied to analyze the images of the green and red fluorescence intensity in each unit. The intensity ratio of red fluorescence to green fluorescence referred to the mitochondrial membrane potential.
An ATP determination kit (Beyotime, Nanjing, China) was employed for determining the content of ATP. A reaction buffer (100 ml) supplemented with dithiothreitol (1 μM), luciferase (12.5 mg/ml), and luciferin (0.5 μM) was added into H9C2 cells. A Varioskan Flash microplate reader was applied for measuring the luminance of the mixtures. The ATP content was defined to be the percentage of the total level in the control group.
Total proteins from H9C2 cells and heart tissues were isolated and separated by 10% sodium dodecyl sulfate-polyacrylamide gel electrophoresis, followed by transferring onto polyvinylidene fluoride membranes (Bio-Rad, Hercules, CA, USA). Being sealed by non-fat milk, the membranes were incubated with the primary antibodies for one night and then washed by Tris-buffered saline with Tween 20. Then a donkey anti-rabbit or anti-mouse IR Dye-conjugated IgG secondary antibody (1:3,000; Abcam) was used for another 1 h incubation. Afterwards, the blots were scanned to capture the images. The labeled protein bands were analyzed via a high-sensitive enhanced chemiluminescent detection kit. The primary antibodies included anti-AMPK (1:1,000, ab32047; Abcam), anti-p-AMPK (1:1,000, ab133448; Abcam), anti-PGC-1α (1 µg/ml, ab191838; Abcam), β-actin (1 µg/ml, ab8226; Abcam).
The concentrations of superoxide dismutase (SOD), glutathione peroxidase (GSH-Px), malondialdehyde (MDA), and catalase (CAT) in H9C2 cells were measured via ELISA. H9C2 cells were planted on a 96-well plate and cultured for 12 h followed by subjecting to respective ELISA kits (Invitrogen). The absorbance at 450 nm was observed.
LDH level was applied for measuring the cell viability. The level of LDH was detected using a commercial assay kit (Roche Applied Science, Mannheim, Germany). Cells were incubated with Triton X-100 (8 µl, 10 %) for 15 min followed by transferring the culture medium (50 µl) onto the 96-well opaque-walled assay plate. Further, cells were incubated with LDH detection reagent (50 µl) for 1 h. The absorbance at 490 nm was evaluated via a spectrophotometer.
The apoptosis of H9C2 cells was evaluated via an Annexin V-FITC/PI double staining kit (Beyotime, Jiangsu, China). After rinsing by PBS, H9C2 cells were cultured with binding buffer (500 µl) and Annexin V (10 µl) for 20 min in the dark followed by incubation with propidium iodide (PI, 5 µl; Sigma). The apoptosis of H9C2 cells was analyzed by flow cytometry.
The data was analyzed using GraphPad Prism 7.0 (GraphPad Software, La Jolla, CA, USA) and displayed as the means ± standard deviation (SD). The differences among multiple groups were compared using one-way or two-way analysis of variance (ANOVA) followed by Tukey’s test while Student’s t-test was applied for comparing the differences between two groups. p < 0.05 was set as statistical significance. All experiments were performed in triplicates
To probe the role of BAC in H9C2 cells, different concentrations of BAC (0 µM, 25 µM, 50 µM, 75µM, 100 µM, 125 µM) were used to treat H9C2 cells. As depicted in Fig. 1A, 25 µM, 50 µM, and 75 µM BAC treatment had no obvious influence on the viability of H9C2 cells, and the H9C2 cell viability was strikingly reduced by 100 µM and 125 µM BAC treatments. Similarly, the secretion of myocardial injury index (LDH) was elevated by the treatment of 100 µM and 125 µM BAC (Fig. 1B). Further, cardiomyocytes injury model was established via OGD/R-treated H9C2 cells. OGD/R treatment markedly decreased the H9C2 cell viability, but 50 µM, and 75 µM BAC treatment evidently increased the OGD/R-treated H9C2 (Fig. 1C). Moreover, the enhanced LDH secretion was reversed by BAC treatment in a dose dependent manner (Fig. 1D). In addition, the apoptosis of H9C2 cells was elevated by OGD/R induction, but suppressed by BAC treatment in a dose dependent manner (Fig. 1E, F). Altogether, BAC relieved OGD/R-induced H9C2 cell injury.
Next, the function of BAC on mitochondrial function in OGD/R-treated H9C2 cells was investigated. The images from JC-1 fluorescence assay revealed that the decreased red/green fluorescence ratio in OGD/R-treated H9C2 cells was elevated by 50 µM, and 75 µM BAC treatment, indicating BAC could improve the mitochondrial function in OGD/R-treated H9C2 cells (Fig. 2A, B). Meanwhile, 50 µM and 75 µM BAC treatment also alleviated OGD/R-triggered enhancement of ROS in H9C2 cells (Fig. 2C). Oppositely, the declined ATP production was counteracted dose-dependently by BAC treatment (Fig. 2D). To sum up, BAC improved mitochondrial function in OGD/R-treated H9C2 cells.
Afterwards, oxidative stress associated biomarkers (SOD, GSH-Px, MDA, and CAT) were tested via ELISA. The data unveiled that the decreased concentration of SOD as a result of OGD/R treatment was attenuated by BAC treatment (25 µM, 50 µM, and 75 µM) in H9C2 cells. Additionally, BAC treatment also inversely changed OGD/R-induced reduction in GSH-Px and CAT concentrations in H9C2 cells dose-dependently. The elevated level of MDA resulted from OGD/R treatment was offset by 50 µM and 75 µM BAC treatment (Fig. 3). All in all, BAC suppressed oxidative stress in OGD/R-treated H9C2 cells.
Subsequently, the mechanism of BAC in OGD/R-treated H9C2 cells was explored. The levels of AMPK/PGC-1 signaling related proteins (p-AMPK and PGC-1α) were assessed. The results uncovered that the p-AMPK and PGC-1α protein levels were decreased in OGD/R-treated H9C2 cells but reversed by BAC treatment dose-dependently (Fig. 4). In a word, BAC activated AMPK/PGC-1 axis in OGD/R-treated H9C2 cells.
Finally, whether BAC modulated OGD/R-treated cardiomyocytes via AMPK/PGC-1 axis was analyzed. BAC treatment restored OGD/R-induced decline in the viability of H9C2 cells, but which was offset by the treatment of AMPK inhibitor (10 µM compound C [CC]) (Fig. 5A). The BAC treatment-improved mitochondrial function in OGD/R-treated H9C2 cells was reversed by 10 µM CC (Fig. 5B). BAC treatment mediated inhibition on ROS level in OGD/R-treated H9C2 cells was reversed by 10 µM CC treatment (Fig. 5C). Besides, 10 µM CC treatment also countervailed the enhanced ATP production resulted from BAC treatment in OGD/R-treated H9C2 cells (Fig. 5D). Taken together, AMPK attenuation reversed the protective effect of BAC on OGD/R-treated cardiomyocytes.
The IR rat model was established to investigate the role of BAC in vivo. Through TTC staining, the heart infarct size was enhanced after IR treatment, but which was rescued by BAC (20 mg/kg) treatment (Fig. 6A). Additionally, the increased LDH and ROS levels mediated by IR treatment could be attenuated by BAC treatment (Fig. 6B, C). Moreover, the down-regulated protein expression of p-AMPK/AMPK and PGC-1α mediated by IR treatment was reversed by BAC treatment (Fig. 6D). These above data revealed that BAC alleviated myocardial injury in vivo.
HF is a chronic health concern with complex pathogenesis [14]. Mitochondria was regarded as the powerhouse of cells via the synthesis ATP through oxidative phosphorylation [15]. The dysfunction of mitochondria has been reported to be implicated in various diseases including HF [11]. Previously, mitochondrial dysfunction has been identified in HF [16]. Moreover, HF is reported to be inhibited by LARP7 via increasing the biogenesis of mitochondria [17]. Multiply researchers indicated that targeting mitochondrial dysfunction might provide an opportunity for HF treatment [18]. Nevertheless, the detailed mechanisms of traditional Chinese medicine regulating mitochondrial dysfunction in HF remain to be explored.
In former studies, traditional Chinese medicine was extensively validated to be involved in cardiomyocytes injury. For instance, the apoptosis and energy metabolism of cardiomyocytes is mediated by Ginsenoside Rb3 through modulating the PPARα pathway [19]. Danqi Pill regulates HIF-1α/PGC-1α-related glucose metabolism pathway to inhibit the development of HF post-acute myocardial infarction [20]. The development of doxorubicin-mediated chronic HF is attenuated by Salsolinol via improving the mitochondrial function in cardiomyocytes [21]. Although BAC has been verified to play a part in the mitochondrial biogenesis [11], RAW264.7 cell responses [12], whether BAC has an effect on HF through modulating the mitochondrial function was unknown. In the current study, different concentrations of BAC were used to treat H9C2 cells, which enhanced H9C2 cell viability. Then, the cardiomyocytes injury model was constructed by OGD/R treatment toH9C2 cells. BAC administration increased the viability and inhibited the apoptosis of H9C2 cells treated by OGD/R. In addition, the attenuated mitochondrial function in OGD/R-treated H9C2 cells was alleviated by BAC treatment. Besides, BAC could suppress oxidative stress in OGD/R-treated H9C2 cells. In summary, BAC could improve the mitochondrial function in OGD/R-treated cardiomyocytes to suppress the development of HF.
Adenosine monophosphate-activated protein kinase (AMPK) is a kind of intracellular energy sensor activated when the cell energy is depleted [22]. Peroxisome proliferator-activated receptor-γ coactivator 1α (PGC-1α) is a member of PGC-1 families, which serves as a transcriptional coactivator that coordinates with physiological adaptation [23]. AMPK/PGC-1α axis has been confirmed to be involved in mitochondrial function and the production of ATP [24]. Besides, AMPK/PGC-1α signaling is found to participate in mitochondrial biogenesis under the regulating of Ursolic acid in C2C12 myotubes [25]. The protective role of Chinese medicine is implicated in the mitochondrial dysfunction in diabetic peripheral neuropathy through modulating the AMPK/PGC-1α signal pathway [26]. AMPK/PGC-1 α axis exerts a role in obese type 2 diabetic rats via mediating the glycolipid metabolism and inflammation [27]. Nonetheless, whether BAC regulated mitochondrial function in HF patients by mediating the AMPK/PGC-1 axis still needs to be explored. Here, the p-AMPK and PGC-1α protein levels were descended in OGD/R-treated H9C2 cells but recovered by BAC treatment. Rescue assays indicated that AMPK attenuation reversed BAC-mediated protective effect on OGD/R-treated cardiomyocytes. Moreover, BAC alleviated myocardial injury in vivo.
In summary, this study firstly corroborated that BAC modulated the mitochondrial function in OGD/R-induced cardiomyocyte injury by activation of the AMPK/PGC-1 axis. The findings of this study might highlight the function of BAC in the treatment of HF.
Notes
REFERENCES
1. Mensah GA, Roth GA, Fuster V. 2019; The global burden of cardiovascular diseases and risk factors: 2020 and beyond. J Am Coll Cardiol. 74:2529–2532. DOI: 10.1016/j.jacc.2019.10.009. PMID: 31727292.
2. Ponikowski P, Voors AA, Anker SD, Bueno H, Cleland JG, Coats AJ, Falk V, González-Juanatey JR, Harjola VP, Jankowska EA, Jessup M, Linde C, Nihoyannopoulos P, Parissis JT, Pieske B, Riley JP, Rosano GM, Ruilope LM, Ruschitzka F, Rutten FH, et al. 2016; 2016 ESC Guidelines for the diagnosis and treatment of acute and chronic heart failure: The Task Force for the diagnosis and treatment of acute and chronic heart failure of the European Society of Cardiology (ESC). Developed with the special contribution of the Heart Failure Association (HFA) of the ESC. Eur J Heart Fail. 18:891–975. DOI: 10.1093/eurheartj/ehw128. PMID: 27206819.


3. Chang IH, Lin LJ, Wu RC. 2022; Acute heart failure from a rapidly progressing large tumor in the right atrium. Signa Vitae. 18:136–138. https://doi.org/10.22514/sv.2021.095. DOI: 10.22514/sv.2021.095.


4. Truby LK, Rogers JG. 2020; Advanced heart failure: epidemiology, diagnosis, and therapeutic approaches. JACC Heart Fail. 8:523–536. DOI: 10.1016/j.jchf.2020.01.014. PMID: 32535126.
5. Jiang H, Xing J, Fang J, Wang L, Wang Y, Zeng L, Li Z, Liu R. 2020; Tilianin protects against ischemia/reperfusion-induced myocardial injury through the inhibition of the Ca2+/calmodulin-dependent protein kinase II-dependent apoptotic and inflammatory signaling pathways. Biomed Res Int. 2020:5939715. DOI: 10.1155/2020/5939715. PMID: 33102583. PMCID: PMC7568786.


6. Geng Y, Hu Y, Wang H, Shi S, Shi J, Qiu Z. 2017; Deficiency of interfibrillar mitochondria in post-acute myocardial infarction heart failure. Pak J Pharm Sci. 30(3(Special)):1089–1094.
7. Niu X, Pu S, Ling C, Xu J, Wang J, Sun S, Yao Y, Zhang Z. 2020; lncRNA Oip5-as1 attenuates myocardial ischaemia/reperfusion injury by sponging miR-29a to activate the SIRT1/AMPK/PGC1α pathway. Cell Prolif. 53:e12818. DOI: 10.1111/cpr.12818. PMID: 32468629. PMCID: PMC7309946.


8. Zhou B, Tian R. 2018; Mitochondrial dysfunction in pathophysiology of heart failure. J Clin Invest. 128:3716–3726. DOI: 10.1172/JCI120849. PMID: 30124471. PMCID: PMC6118589.


9. Tan G, Liao W, Dong X, Yang G, Zhu Z, Li W, Chai Y, Lou Z. 2012; Metabonomic profiles delineate the effect of traditional Chinese medicine sini decoction on myocardial infarction in rats. PLoS One. 7:e34157. DOI: 10.1371/journal.pone.0034157. PMID: 22493681. PMCID: PMC3320902. PMID: 4c6ec630c9434d768502a4722b9bfb21.


10. Liu J, Li Q, Yin Y, Liu R, Xu H, Bi K. 2014; Ultra-fast LC-ESI-MS/MS method for the simultaneous determination of six highly toxic Aconitum alkaloids from Aconiti kusnezoffii radix in rat plasma and its application to a pharmacokinetic study. J Sep Sci. 37:171–178. DOI: 10.1002/jssc.201300775. PMID: 24170571.


11. Deng XH, Liu JJ, Sun XJ, Dong JC, Huang JH. 2019; Benzoylaconine induces mitochondrial biogenesis in mice via activating AMPK signaling cascade. Acta Pharmacol Sin. 40:658–665. DOI: 10.1038/s41401-018-0174-8. PMID: 30315253. PMCID: PMC6786398.


12. Zhou C, Gao J, Ji H, Li W, Xing X, Liu D, Guo Q, Zhou L, Jing F. 2021; Benzoylaconine modulates LPS-induced responses through inhibition of toll-like receptor-mediated NF-κB and MAPK signaling in RAW264.7 cells. Inflammation. 44:2018–2032. DOI: 10.1007/s10753-021-01478-z. PMID: 34272638.


13. Zhang H, Sun S, Zhang W, Xie X, Zhu Z, Chai Y, Zhang G. 2016; Biological activities and pharmacokinetics of aconitine, benzoylaconine, and aconine after oral administration in rats. Drug Test Anal. 8:839–846. DOI: 10.1002/dta.1858. PMID: 26360128.


14. Zhang X, Gao Y, Wu H, Mao Y, Qi Y. 2022; LncRNA HOX transcript antisense RNA mitigates cardiac function injury in chronic heart failure via regulating microRNA-30a-5p to target KDM3A. J Cell Mol Med. 26:1473–1485. DOI: 10.1111/jcmm.17160. PMID: 35083842. PMCID: PMC8899154.


15. van der Bliek AM, Sedensky MM, Morgan PG. 2017; Cell biology of the mitochondrion. Genetics. 207:843–871. Erratum in: Genetics. 2018;208:1673. DOI: 10.1534/genetics.117.300262. PMID: 29097398. PMCID: PMC5676242.


16. Kumar AA, Kelly DP, Chirinos JA. 2019; Mitochondrial dysfunction in heart failure with preserved ejection fraction. Circulation. 139:1435–1450. DOI: 10.1161/CIRCULATIONAHA.118.036259. PMID: 30856000. PMCID: PMC6414077.


17. Yu H, Zhang F, Yan P, Zhang S, Lou Y, Geng Z, Li Z, Zhang Y, Xu Y, Lu Y, Chen C, Wang D, Zhu W, Hu X, Wang J, Zhuang T, Zhang Y, Wu G, Liu J, Zeng C, et al. 2021; LARP7 protects against heart failure by enhancing mitochondrial biogenesis. Circulation. 143:2007–2022. DOI: 10.1161/CIRCULATIONAHA.120.050812. PMID: 33663221.


18. Kiyuna LA, Albuquerque RPE, Chen CH, Mochly-Rosen D, Ferreira JCB. 2018; Targeting mitochondrial dysfunction and oxidative stress in heart failure: challenges and opportunities. Free Radic Biol Med. 129:155–168. DOI: 10.1016/j.freeradbiomed.2018.09.019. PMID: 30227272. PMCID: PMC6309415.


19. Chen X, Wang Q, Shao M, Ma L, Guo D, Wu Y, Gao P, Wang X, Li W, Li C, Wang Y. 2019; Ginsenoside Rb3 regulates energy metabolism and apoptosis in cardiomyocytes via activating PPARα pathway. Biomed Pharmacother. 120:109487. DOI: 10.1016/j.biopha.2019.109487. PMID: 31577975.
20. Zhang Q, Guo D, Wang Y, Wang X, Wang Q, Wu Y, Li C, Wang W, Wang Y. 2020; Danqi pill protects against heart failure post-acute myocardial infarction via HIF-1α/PGC-1α mediated glucose metabolism pathway. Front Pharmacol. 11:458. DOI: 10.3389/fphar.2020.00458. PMID: 32372956. PMCID: PMC7187888. PMID: 6091ca491bf64d798cbc439c4fd89cc0.


21. Wen J, Zhang L, Liu H, Wang J, Li J, Yang Y, Wang Y, Cai H, Li R, Zhao Y. 2019; Salsolinol attenuates doxorubicin-induced chronic heart failure in rats and improves mitochondrial function in H9c2 cardiomyocytes. Front Pharmacol. 10:1135. DOI: 10.3389/fphar.2019.01135. PMID: 31680945. PMCID: PMC6797600.


22. Li Y, Chen Y. 2019; AMPK and autophagy. Adv Exp Med Biol. 1206:85–108. DOI: 10.1007/978-981-15-0602-4_4. PMID: 31776981.


23. Di W, Lv J, Jiang S, Lu C, Yang Z, Ma Z, Hu W, Yang Y, Xu B. 2018; PGC-1: the energetic regulator in cardiac metabolism. Curr Issues Mol Biol. 28:29–46. DOI: 10.21775/cimb.028.029. PMID: 29388552.


24. Cardoso SM, Correia SC, Carvalho C, Moreira PI. 2017; Mitochondria in Alzheimer's disease and diabetes-associated neurodegeneration: license to heal! Handb Exp Pharmacol. 240:281–308. DOI: 10.1007/164_2017_3. PMID: 28251365.


25. Chen J, Wong HS, Leong PK, Leung HY, Chan WM, Ko KM. 2017; Ursolic acid induces mitochondrial biogenesis through the activation of AMPK and PGC-1 in C2C12 myotubes: a possible mechanism underlying its beneficial effect on exercise endurance. Food Funct. 8:2425–2436. DOI: 10.1039/C7FO00127D. PMID: 28675237.


26. Zhang Q, Liang XC. 2019; Effects of mitochondrial dysfunction via AMPK/PGC-1 α signal pathway on pathogenic mechanism of diabetic peripheral neuropathy and the protective effects of Chinese medicine. Chin J Integr Med. 25:386–394. DOI: 10.1007/s11655-018-2579-0. PMID: 30656599.


27. Sun JP, Shi L, Wang F, Qin J, Ke B. 2022; Modified Linggui Zhugan Decoction (加味苓桂术甘汤) ameliorates glycolipid metabolism and inflammation via PI3K-Akt/mTOR-S6K1/AMPK-PGC-1 α signaling pathways in obese type 2 diabetic rats. Chin J Integr Med. 28:52–59. English, Chinese. DOI: 10.1007/s11655-020-3285-2. PMID: 33211278.


Fig. 1
BAC alleviated OGD/R-induced H9C2 cell injury.
(A) MTT assay was performed to evaluate the viability of H9C2 cells. *p < 0.05 indicates the difference compared with vehicle group; **p < 0.01 indicates the difference compared with vehicle group. (B) The LDH level was assessed via the commercial kit. *p < 0.05 indicates the difference compared with vehicle group; **p < 0.01 indicates the difference compared with vehicle group. (C) The viability of H9C2 cells was measured via MTT assay. **p < 0.01 indicates the difference compared with control group; #p < 0.05 indicates the difference compared with OGD (2 h)/R (6 h) + BAC (0 μM) group; ##p < 0.01 indicates the difference compared with OGD (2 h)/R (6 h) + BAC (0 μM) group. (D) The LDH level was detected using the commercial kit. ***p < 0.001 indicates the difference compared with control group; #p < 0.05 indicates the difference compared with OGD (2 h)/R (6 h) + BAC (0 μM) group; ###p < 0.001 indicates the difference compared with OGD (2 h)/R (6 h) + BAC (0 μM) group. (E, F) The apoptosis of H9C2 cells was tested by flow cytometry through Annexin V-FITC/PI double labeling. ***p < 0.001 indicates the difference compared with control group; #p < 0.05 indicates the difference compared with OGD (2 h)/R (6 h) + BAC (0 μM) group; ###p < 0.001 indicates the difference compared with OGD (2 h)/R (6 h) + BAC (0 μM) group. BAC, benzoylaconine; OGD (2 h)/R (6 h), oxygen-glucose deprivation (2 h) and reperfusion (6 h); MTT, 3-(4, 5-dimethylthiazol-2-yl)-2,5-diphenyltetrazolium bromide; LDH, lactate dehydrogenase; PI, propidium iodide.
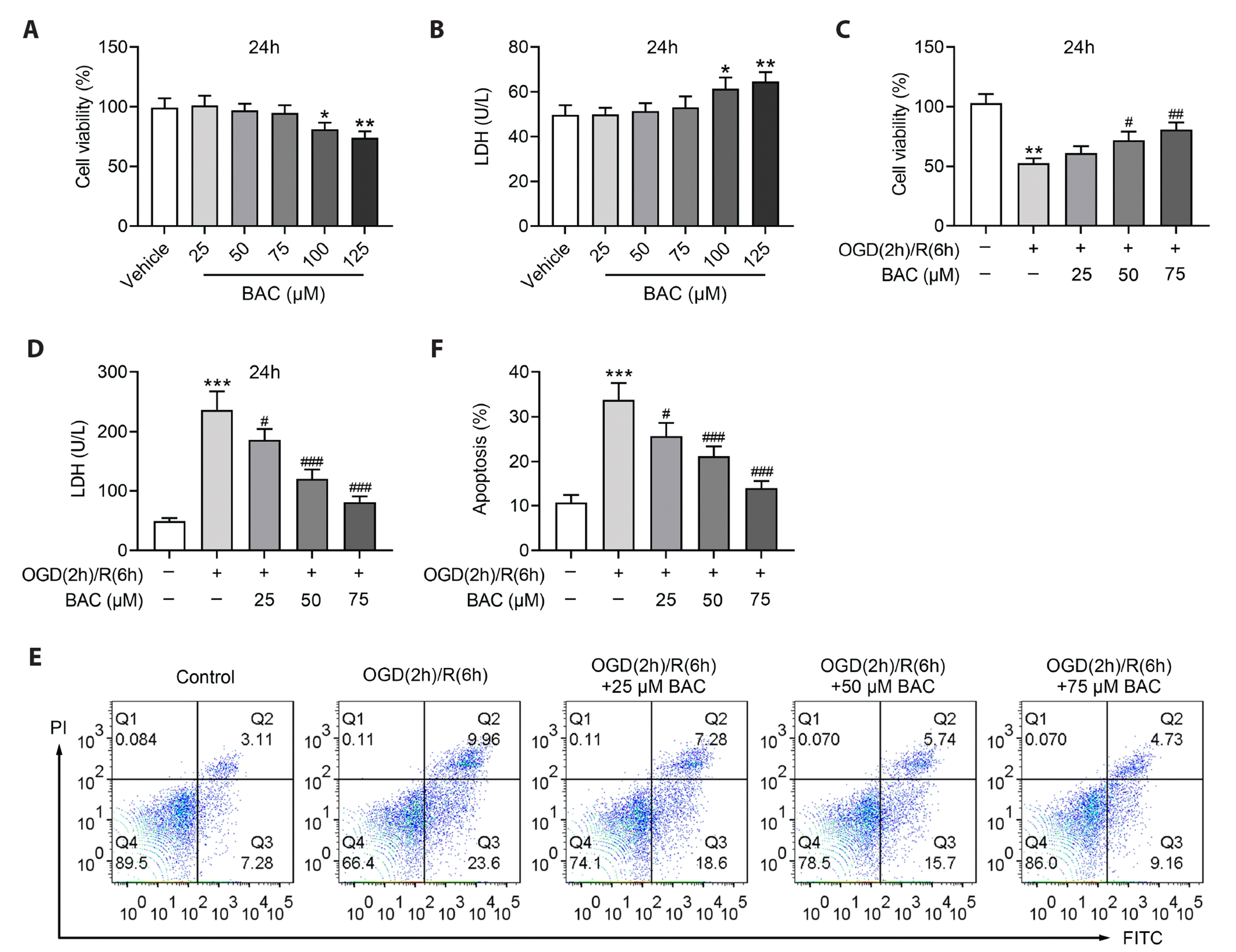
Fig. 2
BAC improved mitochondrial function in OGD/R-treated H9C2 cells.
(A, B) JC-1 fluorescence assay was employed for measuring the mitochondrial membrane potential (scale bar = 50 μm). ***p < 0.001 indicates the difference compared with control group; #p < 0.05 indicates the difference compared with OGD (2 h)/R (6 h) + BAC (0 μM) group; ###p < 0.001 indicates the difference compared with OGD (2 h)/R (6 h) + BAC (0 μM) group. (C, D) The ROS and ATP production were detected through using respective detection kits. ***p < 0.001 indicates the difference compared with control group; ##p < 0.01 indicates the difference compared with OGD (2 h)/R (6 h) + BAC (0 μM) group; ###p < 0.001 indicates the difference compared with OGD (2 h)/R (6 h) + BAC (0 μM) group. BAC, benzoylaconine; OGD (2 h)/R (6 h), oxygen-glucose deprivation (2 h) and reperfusion (6 h); ROS, reactive oxygen species; ATP, adenosine triphosphate; LDH, lactate dehydrogenase.

Fig. 3
BAC suppressed oxidative stress in OGD/R-treated H9C2 cells.
The concentration of SOD, GSH-Px, MDA and CAT was tested via ELISA. ***p < 0.001 indicates the difference compared with control group; #p < 0.05 indicates the difference compared with OGD (2 h)/R (6 h) + BAC (0 μM) group; ##p < 0.01 indicates the difference compared with OGD (2 h)/R (6 h) + BAC (0 μM) group; ###p < 0.001 indicates the difference compared with OGD (2 h)/R (6 h) + BAC (0 μM) group. BAC, benzoylaconine; OGD (2 h)/R (6 h), oxygen-glucose deprivation (2 h) and reperfusion (6 h); SOD, superoxide dismutase; GSH-Px, glutathione peroxidase; MDA, malondialdehyde; CAT, catalase.
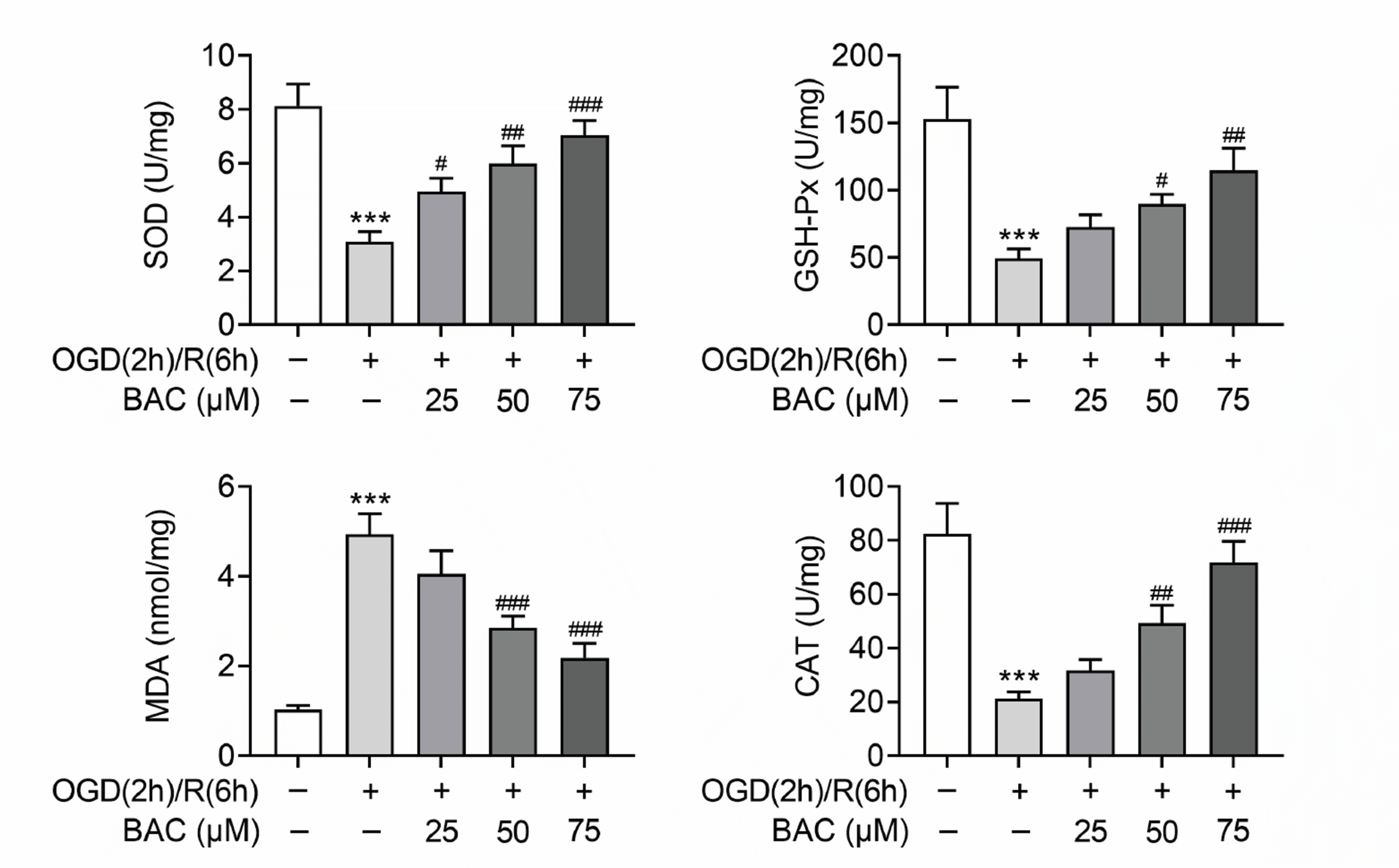
Fig. 4
BAC activated AMPK/PGC-1 axis in OGD/R-treated H9C2 cells.
Western blot analysis was utilized for evaluating the protein levels of AMPK, p-AMPK, and PGC-1α. ***p < 0.001 indicates the difference compared with control group; #p < 0.05 indicates the difference compared with OGD (2 h)/R (6 h) + BAC (0 μM) group; ###p < 0.001 indicates the difference compared with OGD (2 h)/R (6 h) + BAC (0 μM) group. BAC, benzoylaconine; OGD (2 h)/R (6 h), oxygen-glucose deprivation (2 h) and reperfusion (6 h); SOD, superoxide dismutase.
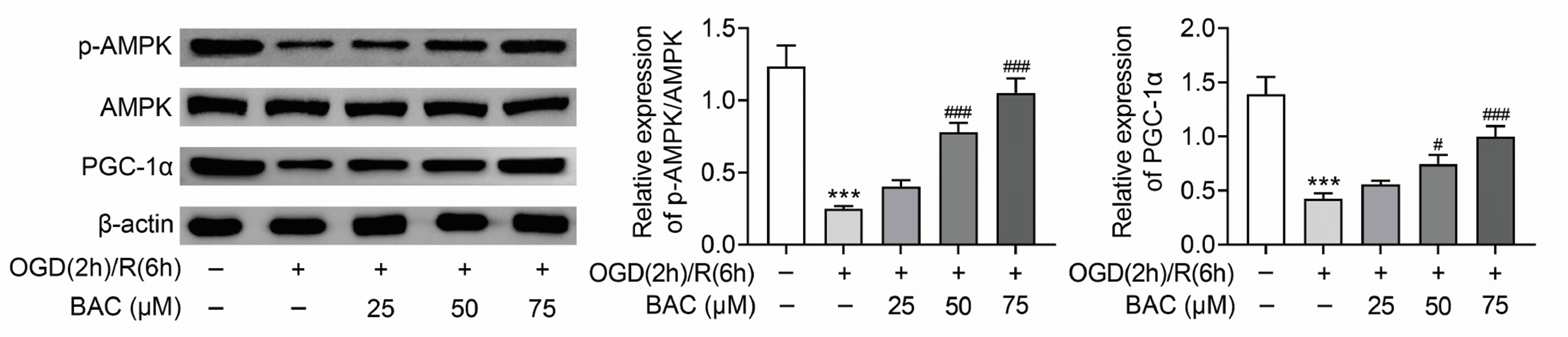
Fig. 5
AMPK attenuation reversed BAC-mediated protective effect on OGD/R-treated cardiomyocytes.
(A) The viability of H9C2 cells was assessed via MTT assay. ***p < 0.001 indicates the difference compared with control group; ##p < 0.01 indicates the difference compared with OGD (2 h)/R (6 h) + BAC (0 μM) group; &&p < 0.01 indicates the difference compared with OGD (2 h)/R (6 h) + BAC (75 μM) group. (B) The mitochondrial membrane potential was detected by JC-1 fluorescence assay (scale bar = 50 μm). ***p < 0.001 indicates the difference compared with control group; ###p < 0.001 indicates the difference compared with OGD (2 h)/R (6 h) + BAC (0 μM) group; &&&p < 0.001 indicates the difference compared with OGD (2 h)/R (6 h) + BAC (75 μM) group. (C, D) The ROS and ATP production were measured using respective detection kits. ***p < 0.001 indicates the difference compared with control group; ###p < 0.001 indicates the difference compared with OGD (2 h)/R (6 h) + BAC (0 μM) group; &&&p < 0.001 indicates the difference compared with OGD (2 h)/R (6 h) + BAC (75 μM) group. BAC, benzoylaconine; OGD (2 h)/R (6 h), oxygen-glucose deprivation (2 h) and reperfusion (6 h); CC, compound C; MTT, 3-(4, 5-dimethylthiazol-2-yl)-2,5-diphenyltetrazolium bromide; ROS, reactive oxygen species; ATP, adenosine triphosphate.
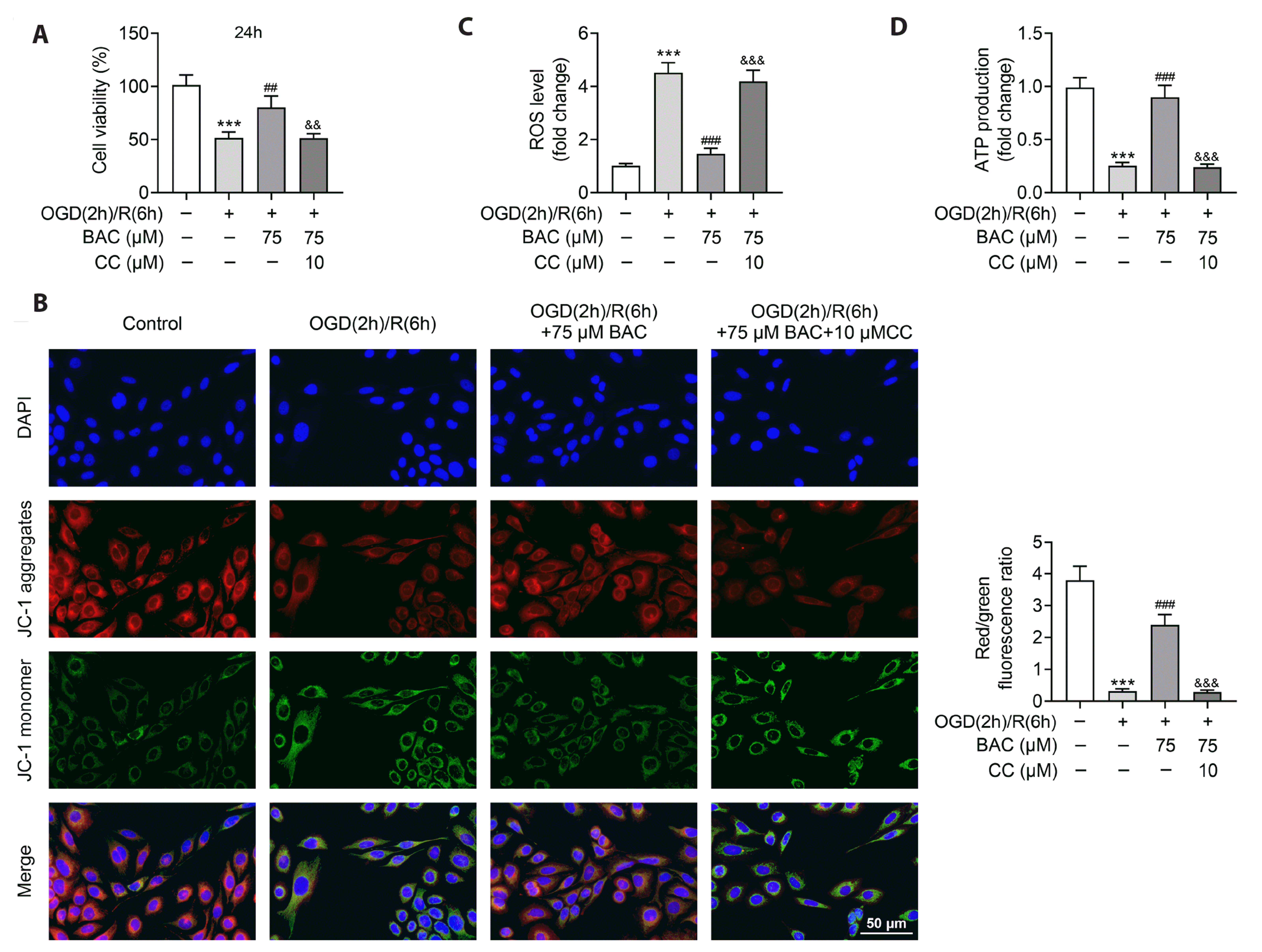
Fig. 6
BAC alleviated myocardial injury in vivo.
(A) The heart infarct size was evaluated by TTC staining. ***p < 0.001 indicates the difference compared with sham group; ###p < 0.001 indicates the difference compared with ischemia reperfusion (IR) group. (B, C) The LDH and ROS levels were measured through the corresponding commercial kits. ***p < 0.001 indicates the difference compared with sham group; ###p < 0.001 indicates the difference compared with IR group. (D) The protein expression of p-AMPK, AMPK, and PGC-1α in heart tissues was assessed through Western blot. ***p < 0.001 indicates the difference compared with sham group; ###p < 0.001 indicates the difference compared with IR group. BAC, benzoylaconine; TTC, 2,3,5-triphenyltetrazolium chloride; LDH, lactate dehydrogenase; ROS, reactive oxygen species.
