Abstract
The presence of artificial light enables humans to be active 24 h a day. Many people across the globe live in a social culture that encourages staying up late to meet the demands of various activities, such as work and school. Sleep deprivation (SD) is a severe health problem in modern society. Meanwhile, as with cardiometabolic disease, there was an obvious tendency that coronary heart disease (CHD) to become a global epidemic chronic disease. Specifically, SD can significantly increase the morbidity and mortality of CHD. However, the underlying mechanisms responsible for the effects of SD on CHD are multilayered and complex. Inflammatory response, lipid metabolism, oxidative stress, and endothelial function all contribute to cardiovascular lesions. In this review, the effects of SD on CHD development are summarized, and SD-related pathogenesis of coronary artery lesions is discussed. In general, early assessment of SD played a vital role in preventing the harmful consequences of CHD.
Many individuals globally live in a social culture that encourages staying up late to meet the demands of various activities, such as work and school. The causes of poor sleep hygiene can include alcohol use, stimulant intake (including caffeine), and excessive Internet usage, as well as disease states, such as sleep apnea, which prevent individuals from acquiring adequate sleep duration and quality [1-8]. At the same time, shift work (e.g., night shifts) can change workers' sleep patterns, leading to emotional impulsiveness, reduced cognitive ability, diminished processing efficiency, and impaired executive function [9-15]. Further, shift workers have been shown to be at greater risk for the development of metabolic disorders and cardiovascular disease (CVD) [16-23].
Sleep deprivation (SD) has become a severe health problem in modern society. While adults require between seven and nine hours of sleep per night, more than a third sleep less than six [24]. The World Health Organization (WHO) defines sleep deprivation as acute sleep restriction, with complete sleep deprivation defined as no sleep ≥ 24 h and partial sleep deprivation (PSD) when an individual sleeps for four hours or less. Sleep disorders include chronic SD and acute SD, both of which have complex consequences. SD can induce different biological effects, such as changes in autonomic nervous system regulation, increased oxidative stress, changes in inflammatory and coagulation responses, and accelerated atherosclerosis progression [25-31]. These mechanisms link SD with CVD and metabolic disorders [16,26,32-35].
Current epidemiological studies [34-45] have confirmed that SD is associated with increased incidence of CVDs, such as coronary artery disease, hypertension, arrhythmia, diabetes, and obesity. People who sleep less than six hours a day are at increased risk of coronary heart disease (CHD), compared with those who sleep between six and nine hours a day [46]. Therefore, early assessment of SD is associated with preventing harmful consequences of CVDs, such as CHD [47-51].
Short sleep duration is a key risk factor for CHD. In 2018, a cross-sectional study illustrated that the differences between heart age and chronological age were very slight in adults who slept seven hours per night. In contrast, differences significantly increased as sleep duration decreased or increased [52]. In 2018, a study involving 31,830 participants showed that people who slept less than six hours a day were significantly more likely to have non-fatal cardiovascular events than those who slept seven to eight hours [53]. According to epidemiological studies [54-57], abnormal sleep duration is a risk factor for CHD. Accordingly, several cross-sectional studies have shown that sleep duration of fewer than six hours significantly increases the risk of CHD, myocardial infarction (MI), non-fatal cardiovascular events, and cardiovascular death, as shown in Table 1 [53-56,58,59]. Compared with optimal sleep duration, SD is significantly associated with CHD, with a U-shaped relationship between sleep duration and CHD observed [54-57].
SD increases the contracture and necrosis of cardiomyocytes after ischemia-reperfusion injury. In 2015, one study [60] using PSD as a model confirmed that myocardial electrical activity of PSD rats was abnormal, suggesting that PSD may cause myocardial cell damage and that SD increases the risk of CHD. In 2015, a study using complete SD as a model [61] illustrated that, compared with the control group, serum creatine phosphokinase and lactate dehydrogenase in the SD group significantly increased in a time-dependent manner. This study showed that SD leads to myocardial injury, and that the degree of myocardial injury was positively correlated with SD time.
Additionally, SD show a significant impact on MI. From 2016 to 2018, Jeddi et al. [62,63] conducted a model of acute SD for 96 h in rats, and found nitrite + nitrate levels and infarct area in the heart of SD rats were significantly higher than those in the control group. Compared with the control group, inducible nitric oxide synthase and BCL2-Associated X expression in SD rats increased, while Bcl-2 expression decreased. At the same time, basal cardiac function and tolerance to ischemia-reperfusion injury was decreased in SD rats, which may be related to the increase of nitric oxide (NO) production after ischemia-reperfusion. In 2017, Aghajani et al. [64] conducted a model of chronic SD for six days in rats. SD after MI leads to heart enlargement at 21 days, marked by increased oxidative stress and NO production, as well as an imbalance of the ubiquitin-proteasome system. Together, these effects lead to cardiac dysfunction and heart failure.
Thus, both acute and chronic SD can cause myocardial injury, increase CHD risk, and aggravate ischemia-reperfusion injuries following myocardial events. Avoiding SD is of great significance for the prevention of CHD and post-MI treatment.
The mechanisms that are responsible for the effects of SD on CHD are multilayered and complex. Inflammatory response, lipid metabolism, oxidative stress, and endothelial function all contribute to essential factors leading to cardiovascular lesions. However, these changes are interrelated and form a network of risk factors for CHD (Fig. 1).
The interaction between SD and immune disorders is bidirectional, with SD triggering an inflammatory response. In 2015, Carroll et al. [65] conducted a cross-sectional study of 70 volunteers, showed that PSD increased the production of the inflammatory cytokines interleukin-6 (IL-6) and tumor necrosis factor-α (TNF-α). Animal studies [66-68] have also shown that the levels of pro-inflammatory cytokines and markers in SD rats significantly increased. During acute SD, inflammation activated through the complementary C3a-C3aR and C5a-C5aR pathways. Phagocytes in the peripheral circulation, such as monocytes and neutrophils increased, while IL-6 and C-reactive protein also increased. Production of circulating naive T cells and pro-inflammatory cytokines, such as IL-12, peaks at night, while production of cytotoxic effector leukocytes and anti-inflammatory cytokine IL-10 peaks during the day. Therefore, the circadian system and sleep work together to evoke a unique endocrine mechanism that effectively induces changes in white blood cell count and conversion to pro-inflammatory type 1 cytokines during nocturnal sleep [69].
In 2019, Said et al. [70] and De Lorenzo et al. [71], through examination of multiple models, showed that chronic SD may lead to changes in the balance of TH1-related chemokines, resulting in dysfunction in the distribution of circulating white blood cells, affecting neutrophil phagocytosis and nicotinamide adenine dinucleotide phosphate oxidase activity, leading to decreased CD4+ and CD8+ T cells in peripheral blood, and ultimately, resulting in immunosuppression. At the same time, diminished sleep duration leads to a decrease in the production of hypocretin (a neuropeptide that stimulates and promotes arousal) in the lateral hypothalamus. Additionally, SD results in an increase in the production of colony-stimulating factor-1 in hematopoietic cells. Colony-stimulating factor-1 induces the generation of macrophages and Ly-6Chigh monocytes. These inflammatory cells induce atherosclerotic lesions [72]. Therefore, SD induces inflammatory responses and the release of inflammatory mediators [66-68]. Pro-inflammatory mediators and inflammatory cells play an essential role in myocardial injury by inducing atherosclerosis, MI, apoptosis, and reperfusion injury.
SD has a strong effect on lipid metabolism, which leads to atherosclerosis. In 2011, Kong et al. [73] published a cross-sectional study of a sample from Hong Kong, China. The study showed that children with long sleep duration had significantly reduced total cholesterol (TC) and low-density lipoprotein cholesterol (LDL-C) levels compared with children with short sleep duration. Reduced sleep duration was also found to be associated with obesity and atherosclerotic dyslipidemia in young Hong Kong children. In 2019, Ness et al. [74] published a prospective study of 15 volunteers, and found that PSD for five days affected non-esterified fatty acid (NEFA) metabolism. A separate perspective cohort study [75] demonstrated a significant association between serum total NEFA concentration and the incidence of CHD mortality and non-fatal myocardial infarction.
In 2020, animal experiments conducted by Xing et al. [76] showed that after acute sleep deprivation 72 h in rats and mice, nuclear receptor subfamily 1 group D member 1-mediated inhibition of cholesterol 7α-hydroxylase can lead to elevated serum cholesterol level, hepatic cholesterol accumulation. At the same time, this may be related to the fact that sleep deprivation disrupted the secondary biological clock of mice [77]. It broke the rhythm of the inositol-requiring enzyme-1α pathway in the endoplasmic reticulum, and brought about incongruous expression levels of enzymes, which involved in fatty acid and cholesterol metabolism. Ultimately, the above mechanism caused impaired lipid metabolism. Long-term and uncontrolled exposure to hypercholesterolemia can lead to slowly progressive cardiovascular events [78]. In 2019, Wilms et al. [79] enrolled 15 healthy young men. They were assigned to three groups: regular sleep schedule (8 h), sleep restriction (4 h), and sleep deprivation (no sleep at all). The results showed that acute sleep deprivation leaded to profound remodelling of the transcriptomes of white adipose tissue (WAT), resulting in increased carbohydrate turnover and impaired glucose homeostasis. There are robust rhythms throughout WAT as well as subcutaneous adipose stem cells. These factors are connected with circadian rhythmic neuroendocrine hormones, such as growth hormone and glucocorticoids, both of which are known to affect the function and quality of adipose tissue [80]. Sleep deprivation causes hormonal changes, and there are complicated relationship between subtle hormonal changes and lipid metabolism disorders.
Many studies have shown that SD can aggravate myocardial ischemia-reperfusion injury by increasing energy consumption, decreasing antioxidant capacity, increasing oxygen free radical accumulation, and aggravating endoplasmic reticulum stress. Chronic circadian disruption usually activates the adaptive stress response. However, endoplasmic reticulum stress is the dominating catalyst of cell death in atherosclerosis. Figueiro et al. [81] found that GRP78/BiP, an endoplasmic reticulum chaperone for endoplasmic reticulum stress, was approximately 3 times higher in the macrophage-rich region of plaques for sleep-deprived mice than normal group. Arterial foam cells have a circadian rhythm response. Sleep deprivation can destroy the molecular clock of them and increase endoplasmic reticulum stress and apoptosis.
In 2005, Everson et al. [82] found catalase activity and glutathione content in the liver decreased by 23%–36% in rats subjected to five and ten days of SD, which continued or worsened with prolonged SD. Prospective studies involving volunteers by Trivedi et al. [83] and Jówko et al. [84] showed that after 24 h of acute SD, levels of glutathione, adenosine triphosphate (ATP), cysteine, and homocysteine decreased significantly, while activity of glutathione peroxidase and erythrocyte superoxide dismutase decreased, and the residual total antioxidant capacity in plasma increased.
Valvassori et al. [85], Rodrigues et al. [86], and Vosahlikova et al. [87], using acute sleep models, found that SD increased lipid peroxidation and DNA oxidative damage by down-regulating Na+/K+-ATPase activity, and caused changes in antioxidant enzymes in the frontal cortex, hippocampus, and serum, increased reactive oxygen species production, impaired mitochondrial biological ability, and affected mitochondrial activity, antioxidant defense enzyme, and caspase activity, which reduced oxidative phosphorylation and electron transport system respiration. In 2014, Qin and Deng [88], using a mouse model of SD lasting for seven days, found that SD increased inflammation and oxidative stress, and decreased the expression of cryptopigment-1 (CRY1) in vascular endothelial cells. Furthermore, the plaque area of the aortic sinus and the concentrations of TC, triglyceride, and LDL-C were also decreased in atherosclerotic mice by CRY1 overexpression. Overexpression of CYR1 relieves the development of atherosclerosis that may be associated with regulation of the toll-like receptor (TLR)/nuclear factor kappa B (NF-κB) and cyclic adenosine monophosphate/protein kinase A (cAMP/PKA) pathway [88,89]. In 2020, Schilperoort et al. [90] observed increased expression of chemokine C-C motif ligand 2 (CCL2) protein in atherosclerotic lesions of mice, which exposed to alternating light and dark cycles. CCL2 is a chemokine that actively recruited monocytes to the site of endothelial injury. Inflammation and oxidative stress can induce CCL2 expression. CCL2-CCR2 axis plays an essential role in atherosclerosis. Therefore, oxidative stress and inflammation are the fundamental driving factors of endothelial dysfunction.
Vascular homeostasis and maintenance of endothelial function demonstrated functional rhythm oscillations synchronized with the 24-h diurnal cycle. Sleep disorders related to inflammation and sympathetic activation lead to a change in blood vessel architecture, characterized by elastic fiber fracture and disorder, an increase in inflammatory cells, lipid peroxidation, inflammation, and sympathetic activation, which may induce endothelial dysfunction. Endothelial dysfunction is a critical factor in the increased risk of CVD [91-95]. In models of acute and chronic SD in healthy volunteers, Sauvet et al. [96,97], Dettoni et al. [98], measured biomarkers of microvascular reactivity and endothelial activation, elevated plasma E-selectin levels, and significantly increased sympathetic neural activity during SD. Increased serum norepinephrine decreased endothelium-dependent venous dilatation, increased venous endothelial dysfunction, decreased endothelium-dependent vascular dilatation, and decreased local endothelial tolerance. Meanwhile, vascular dysfunction may precede and be independent of sympathetic nerve activity and systolic blood pressure increases. This suggests that endothelial dysfunction is not associated with blood pressure and sympathetic activity, but with inflammation and metabolic pathway responses.
More than 40% of protein-coding genes in human cells are controled by circadian clock gene, which showed tissue-specific circadian oscillations through clock-controlled transcription factors [99]. Many of these genes, which called CLOCK control genes (CCGs), played specific roles in regulating atherosclerosis of endothelial cells. Transcription factors brain-muscle-ARNT-like protein-2 (BMAL1) and CLOCK heterodimers combined with the promoter region of the period (PER1/2) and cryptochrome (CRY1/2) genes [100,101]. CLOCK/BMAL2 heterodimers integrated promoters of endothelial CCGs in the e-box enhancer region, such as plasminogen activator inhibitor-1 (PAI-1) and thrombomodulin (TM) [102,103]. Both PAI-1 and TM are tightly associated with endothelial activation and the development of atherosclerotic plaques. Short sleep duration may exacerbate the instability of atherosclerosis and plaque by regulating CCGs expression in endothelial cells.
NO-mediated impairment of vasodilation contributes to atherosclerotic vascular disease and acute cardiovascular events. Prospective studies by Bain et al. [104], Sauvet et al. [105], and Stockelman et al. [106] showed that short sleep duration at night was associated with endothelium-dependent vasodilation dysfunction. This may be partly due to reduced bioavailability of NO, which leads to impaired vasodilation. At the same time, acetylcholine induces a decrease in vasodilation by increasing the concentration of pro-inflammatory cytokine TNF-α. endothelin-1 mediated increased vasoconstriction, resulting in increased pulse wave velocity, decreased vascular elasticity, and ultimately increased cardiovascular risk. In 2017, Jiang et al. [107] showed that the concentration of NO and cyclic guanosine phosphate (cGMP) and the phosphorylation level of endothelial NO synthase (eNOS) in the aorta decreased in rats subjected to five days of rapid movement SD. This suggests that SD can cause endothelial dysfunction and hypertension in middle-aged rats through the eNOS/NO/cGMP pathway.
Recent studies [108] have shown that chronic SD is associated with significantly reduced circulating levels of miRNA-125a, miRNA-126, and miRNA-146a. Dysregulation of these miRNAs may lead to increased inflammatory burden and endothelial dysfunction. miRNA-26a-5p induces the apoptosis of endothelial cells in CHD by inhibiting phosphatidyl inositol-3 kinase/protein kinase B pathway [109]. Acute SD alters methylation levels in healthy individuals [110]. These epigenomic changes may be used as biomarkers for sleep loss or as therapeutic targets for sleep-related diseases [111-114].
CHD, like CVD, is a critical problem threatening human health. SD is significantly associated with increased morbidity and mortality of CHD. Mate analysis showed a U-shaped relationship between sleep duration and the risk of CHD morbidity and mortality; sleep duration < 6.5 h/d was a risk factor of CHD, and increased the risk of CHD morbidity and mortality. However, its pathogenesis is complex and is the focus of current research. The experimental data reviewed in this paper show that inflammatory response, lipid metabolism, oxidative stress, and endothelial function are essential factors leading to vascular lesions, as shown in Fig. 1. In general, ensuring adequate sleep time to prevent CHD and MI after treatment is significant. Specifically, early intervention and treatment of SD can reduce cardiovascular morbidity and mortality among night shift workers.
REFERENCES
1. Riemann D. 2018; Sleep hygiene, insomnia and mental health. J Sleep Res. 27:3. DOI: 10.1111/jsr.12713. PMID: 29878665.


2. Kansagra S. 2020; Sleep disorders in adolescents. Pediatrics. 145(Suppl 2):S204–S209. DOI: 10.1542/peds.2019-2056I. PMID: 32358212.


3. Yaremchuk K. 2018; Sleep disorders in the elderly. Clin Geriatr Med. 34:205–216. DOI: 10.1016/j.cger.2018.01.008. PMID: 29661333.


4. Shriane AE, Ferguson SA, Jay SM, Vincent GE. 2020; Sleep hygiene in shift workers: a systematic literature review. Sleep Med Rev. 53:101336. DOI: 10.1016/j.smrv.2020.101336. PMID: 32593135.


5. Jung SY, Kim HS, Min JY, Hwang KJ, Kim SW. 2019; Sleep hygiene-related conditions in patients with mild to moderate obstructive sleep apnea. Auris Nasus Larynx. 46:95–100. DOI: 10.1016/j.anl.2018.06.003. PMID: 29934236.


6. van der Heijden KB, Vermeulen MCM, Donjacour CEHM, Gordijn MCM, Hamburger HL, Meijer AM, van Rijn KJ, Vlak M, Weysen T. 2018; Chronic sleep reduction is associated with academic achievement and study concentration in higher education students. J Sleep Res. 27:165–174. DOI: 10.1111/jsr.12596. PMID: 28880425.


7. Booker LA, Barnes M, Alvaro P, Collins A, Chai-Coetzer CL, McMahon M, Lockley SW, Rajaratnam SMW, Howard ME, Sletten TL. 2020; The role of sleep hygiene in the risk of Shift Work Disorder in nurses. Sleep. 43:zsz228. DOI: 10.1093/sleep/zsz228. PMID: 31637435.


8. Snyder CK. 2020; Biopsychosocial outcomes of poor sleep in adolescents. J Pediatr Nurs. 54:114–115. DOI: 10.1016/j.pedn.2020.06.006. PMID: 32616454.


9. Choshen-Hillel S, Ishqer A, Mahameed F, Reiter J, Gozal D, Gileles-Hillel A, Berger I. 2021; Acute and chronic sleep deprivation in residents: cognition and stress biomarkers. Med Educ. 55:174–184. DOI: 10.1111/medu.14296. PMID: 32697336. PMCID: PMC7854866.


10. Barnes CM, Watson NF. 2019; Why healthy sleep is good for business. Sleep Med Rev. 47:112–118. DOI: 10.1016/j.smrv.2019.07.005. PMID: 31450119.


11. Garbarino S, Tripepi G, Magnavita N. 2020; Sleep health promotion in the workplace. Int J Environ Res Public Health. 17:7952. DOI: 10.3390/ijerph17217952. PMID: 33138203. PMCID: PMC7663389. PMID: 22b8ca34c6274bd79ef6a4766355d732.


12. Chaput JP, Dutil C, Featherstone R, Ross R, Giangregorio L, Saunders TJ, Janssen I, Poitras VJ, Kho ME, Ross-White A, Zankar S, Carrier J. 2020; Sleep timing, sleep consistency, and health in adults: a systematic review. Appl Physiol Nutr Metab. 45(10 Suppl 2):S232–S247. DOI: 10.1139/apnm-2020-0032. PMID: 33054339.


13. Kang J, Noh W, Lee Y. 2020; Sleep quality among shift-work nurses: a systematic review and meta-analysis. Appl Nurs Res. 52:151227. DOI: 10.1016/j.apnr.2019.151227. PMID: 31902652.


14. Khan S, Duan P, Yao L, Hou H. 2018; Shiftwork-mediated disruptions of circadian rhythms and sleep homeostasis cause serious health problems. Int J Genomics. 2018:8576890. DOI: 10.1155/2018/8576890. PMID: 29607311. PMCID: PMC5828540.


15. Heath G, Dorrian J, Coates A. 2019; Associations between shift type, sleep, mood, and diet in a group of shift working nurses. Scand J Work Environ Health. 45:402–412. DOI: 10.5271/sjweh.3803. PMID: 30806474. PMID: d6a10adf85b54a0ca30ab107c7298db3.


16. Lunde LK, Skare Ø, Mamen A, Sirnes PA, Aass HCD, Øvstebø R, Goffeng E, Matre D, Nielsen P, Heglum HSA, Hammer SE, Skogstad M. 2020; Cardiovascular health effects of shift work with long working hours and night shifts: study protocol for a three-year prospective follow-up study on industrial workers. Int J Environ Res Public Health. 17:589. DOI: 10.3390/ijerph17020589. PMID: 31963313. PMCID: PMC7014249.


17. Kuetting DLR, Feisst A, Sprinkart AM, Homsi R, Luetkens J, Thomas D, Schild HH, Dabir D. 2019; Effects of a 24-hr-shift-related short-term sleep deprivation on cardiac function: a cardiac magnetic resonance-based study. J Sleep Res. 28:e12665. DOI: 10.1111/jsr.12665. PMID: 29411477.


18. Gohari A, Wiebe D, Ayas N. 2021; Shift working and cardiovascular health. Chronobiol Int. doi: 10.1080/07420528.2021.1933000. [Epub ahead of print]. DOI: 10.1080/07420528.2021.1933000. PMID: 34100313.


19. Chellappa SL, Vujovic N, Williams JS, Scheer FAJL. 2019; Impact of circadian disruption on cardiovascular function and disease. Trends Endocrinol Metab. 30:767–779. DOI: 10.1016/j.tem.2019.07.008. PMID: 31427142. PMCID: PMC6779516.


20. McHill AW, Velasco J, Bodner T, Shea SA, Olson R. 2022; Rapid changes in overnight blood pressure after transitioning to early-morning shiftwork. Sleep. 45:zsab203. DOI: 10.1093/sleep/zsab203. PMID: 34369575. PMCID: PMC8919200.


21. Choi WS, Lee JW, Lee JY, Kim KY, Myong JP, Lee WC. 2019; The effect of special medical examination for night shift workers and follow-up management against hypertension. Int J Environ Res Public Health. 16:719. DOI: 10.3390/ijerph16050719. PMID: 30823384. PMCID: PMC6427592.


22. Gamboa Madeira S, Reis C, Paiva T, Moreira CS, Nogueira P, Roenneberg T. 2021; Social jetlag, a novel predictor for high cardiovascular risk in blue-collar workers following permanent atypical work schedules. J Sleep Res. 30:e13380. DOI: 10.1111/jsr.13380. PMID: 33942925. PMCID: PMC9286443.


23. Zhu B, Shi C, Park CG, Zhao X, Reutrakul S. 2019; Effects of sleep restriction on metabolism-related parameters in healthy adults: a comprehensive review and meta-analysis of randomized controlled trials. Sleep Med Rev. 45:18–30. DOI: 10.1016/j.smrv.2019.02.002. PMID: 30870662.


24. Lee S. 2022; Naturally occurring consecutive sleep loss and day-to-day trajectories of affective and physical well-being. Ann Behav Med. 56:393–404. DOI: 10.1093/abm/kaab055. PMID: 34223608. PMCID: PMC8976290.


25. Tobaldini E, Costantino G, Solbiati M, Cogliati C, Kara T, Nobili L, Montano N. 2017; Sleep, sleep deprivation, autonomic nervous system and cardiovascular diseases. Neurosci Biobehav Rev. 74(Pt B):321–329. DOI: 10.1016/j.neubiorev.2016.07.004. PMID: 27397854.


26. Besedovsky L, Lange T, Haack M. 2019; The sleep-immune crosstalk in health and disease. Physiol Rev. 99:1325–1380. DOI: 10.1152/physrev.00010.2018. PMID: 30920354. PMCID: PMC6689741.


27. Domínguez F, Fuster V, Fernández-Alvira JM, Fernández-Friera L, López-Melgar B, Blanco-Rojo R, Fernández-Ortiz A, García-Pavía P, Sanz J, Mendiguren JM, Ibañez B, Bueno H, Lara-Pezzi E, Ordovás JM. 2019; Association of sleep duration and quality with subclinical atherosclerosis. J Am Coll Cardiol. 73:134–144. DOI: 10.1016/j.jacc.2018.10.060. PMID: 30654884.


28. Lechner K, von Schacky C, McKenzie AL, Worm N, Nixdorff U, Lechner B, Kränkel N, Halle M, Krauss RM, Scherr J. 2020; Lifestyle factors and high-risk atherosclerosis: pathways and mechanisms beyond traditional risk factors. Eur J Prev Cardiol. 27:394–406. DOI: 10.1177/2047487319869400. PMID: 31408370. PMCID: PMC7065445.


29. Vallat R, Shah VD, Redline S, Attia P, Walker MP. 2020; Broken sleep predicts hardened blood vessels. PLoS Biol. 18:e3000726. DOI: 10.1371/journal.pbio.3000726. PMID: 32497046. PMCID: PMC7271997. PMID: 90cf0c61615445aeb840f74579f87ba1.


30. Chen S, Yang Y, Cheng GL, Jia J, Fan FF, Li JP, Huo Y, Zhang Y, Chen DF. 2018; Association between short sleep duration and carotid atherosclerosis modified by age in a Chinese community population. J Epidemiol Community Health. 72:539–544. DOI: 10.1136/jech-2017-209464. PMID: 29449352.


31. Carroll JE, Irwin MR, Seeman TE, Diez-Roux AV, Prather AA, Olmstead R, Epel E, Lin J, Redline S. 2019; Obstructive sleep apnea, nighttime arousals, and leukocyte telomere length: the Multi-Ethnic Study of Atherosclerosis. Sleep. 42:zsz089. DOI: 10.1093/sleep/zsz089. PMID: 30994174. PMCID: PMC6612669.
32. Huang T, Mariani S, Redline S. 2020; Sleep irregularity and risk of cardiovascular events: the Multi-Ethnic Study of Atherosclerosis. J Am Coll Cardiol. 75:991–999. DOI: 10.1016/j.jacc.2019.12.054. PMID: 32138974. PMCID: PMC7237955.
33. Liew SC, Aung T. 2021; Sleep deprivation and its association with diseases- a review. Sleep Med. 77:192–204. DOI: 10.1016/j.sleep.2020.07.048. PMID: 32951993.


34. Makarem N, Shechter A, Carnethon MR, Mullington JM, Hall MH, Abdalla M. 2019; Sleep duration and blood pressure: recent advances and future directions. Curr Hypertens Rep. 21:33. DOI: 10.1007/s11906-019-0938-7. PMID: 30953237.
35. Muscente F, De Caterina R. 2020; Challenges in ischaemic heart disease: not sleeping enough, not brushing your teeth, and skipping breakfast-three ways of increasing your risk of myocardial infarction? Eur Heart J Suppl. 22(Suppl L):L57–L60. DOI: 10.1093/eurheartj/suaa136. PMID: 33654468. PMCID: PMC7904058.
36. Beaman A, Bhide MC, McHill AW, Thosar SS. 2021; Biological pathways underlying the association between habitual long-sleep and elevated cardiovascular risk in adults. Sleep Med. 78:135–140. DOI: 10.1016/j.sleep.2020.12.011. PMID: 33429289. PMCID: PMC7887071.


37. Feng X, Liu Q, Li Y, Zhao F, Chang H, Lyu J. 2019; Longitudinal study of the relationship between sleep duration and hypertension in Chinese adult residents (CHNS 2004-2011). Sleep Med. 58:88–92. DOI: 10.1016/j.sleep.2019.01.006. PMID: 31132577.


38. Merikanto I, Lahti T, Puolijoki H, Vanhala M, Peltonen M, Laatikainen T, Vartiainen E, Salomaa V, Kronholm E, Partonen T. 2013; Associations of chronotype and sleep with cardiovascular diseases and type 2 diabetes. Chronobiol Int. 30:470–477. DOI: 10.3109/07420528.2012.741171. PMID: 23281716.


39. Fang J, Wheaton AG, Keenan NL, Greenlund KJ, Perry GS, Croft JB. 2012; Association of sleep duration and hypertension among US adults varies by age and sex. Am J Hypertens. 25:335–341. DOI: 10.1038/ajh.2011.201. PMID: 22052075.


40. Gangwisch JE, Feskanich D, Malaspina D, Shen S, Forman JP. 2013; Sleep duration and risk for hypertension in women: results from the nurses' health study. Am J Hypertens. 26:903–911. DOI: 10.1093/ajh/hpt044. PMID: 23564028. PMCID: PMC3731821.


41. Matassini MV, Brambatti M, Guerra F, Scappini L, Capucci A. 2015; Sleep-disordered breathing and atrial fibrillation: review of the evidence. Cardiol Rev. 23:79–86. DOI: 10.1097/CRD.0000000000000027. PMID: 25098199.
42. Marti-Almor J, Marques P, Jesel L, Garcia R, Di Girolamo E, Locati F, Defaye P, Venables P, Dompnier A, Barcelo A, Nägele H, Burri H. 2020; Incidence of sleep apnea and association with atrial fibrillation in an unselected pacemaker population: results of the observational RESPIRE study. Heart Rhythm. 17:195–202. DOI: 10.1016/j.hrthm.2019.09.001. PMID: 31493591.


43. Borel AL. 2019; Sleep apnea and sleep habits: relationships with metabolic syndrome. Nutrients. 11:2628. DOI: 10.3390/nu11112628. PMID: 31684029. PMCID: PMC6893600.


44. Oliveira RF, Daniele TMDC, Façanha CFS, Forti ACE, Bruin PFC, Bruin VMS. 2018; Adiponectin levels and sleep deprivation in patients with endocrine metabolic disorders. Rev Assoc Med Bras (1992). 64:1122–1128. DOI: 10.1590/1806-9282.64.12.1122. PMID: 30569989.


45. Simon SL, Higgins J, Melanson E, Wright KP Jr, Nadeau KJ. 2021; A model of adolescent sleep health and risk for type 2 diabetes. Curr Diab Rep. 21:4. DOI: 10.1007/s11892-020-01373-1. PMID: 33449241. PMCID: PMC7810106.


46. Liu H, Chen A. 2019; Roles of sleep deprivation in cardiovascular dysfunctions. Life Sci. 219:231–237. DOI: 10.1016/j.lfs.2019.01.006. PMID: 30630005.


47. Fan M, Sun D, Zhou T, Heianza Y, Lv J, Li L, Qi L. 2020; Sleep patterns, genetic susceptibility, and incident cardiovascular disease: a prospective study of 385 292 UK biobank participants. Eur Heart J. 41:1182–1189. DOI: 10.1093/eurheartj/ehz849. PMID: 31848595. PMCID: PMC7071844.


48. Madsen MT, Huang C, Zangger G, Zwisler ADO, Gögenur I. 2019; Sleep disturbances in patients with coronary heart disease: a systematic review. J Clin Sleep Med. 15:489–504. DOI: 10.5664/jcsm.7684. PMID: 30853047. PMCID: PMC6411183.


49. Choi Y, Choi JW. 2020; Association of sleep disturbance with risk of cardiovascular disease and all-cause mortality in patients with new-onset type 2 diabetes: data from the Korean NHIS-HEALS. Cardiovasc Diabetol. 19:61. DOI: 10.1186/s12933-020-01032-5. PMID: 32404104. PMCID: PMC7222449. PMID: 08b506aa7f7f42618549892f05b0fdd4.


50. Huang T, Zeleznik OA, Poole EM, Clish CB, Deik AA, Scott JM, Vetter C, Schernhammer ES, Brunner R, Hale L, Manson JE, Hu FB, Redline S, Tworoger SS, Rexrode KM. 2019; Habitual sleep quality, plasma metabolites and risk of coronary heart disease in post-menopausal women. Int J Epidemiol. 48:1262–1274. DOI: 10.1093/ije/dyy234. PMID: 30371783. PMCID: PMC6693883.


51. Svensson AK, Svensson T, Kitlinski M, Almgren P, Engström G, Nilsson PM, Melander O. 2018; Incident diabetes mellitus may explain the association between sleep duration and incident coronary heart disease. Diabetologia. 61:331–341. DOI: 10.1007/s00125-017-4464-3. PMID: 29103105. PMCID: PMC6448951.


52. Yang Q, Durmer JL, Wheaton AG, Jackson SL, Zhang Z. 2018; Sleep duration and excess heart age among US adults. Sleep Health. 4:448–455. DOI: 10.1016/j.sleh.2018.07.001. PMID: 30241660.


53. Kobayashi D, Kuriyama N, Osugi Y, Arioka H, Takahashi O. 2018; Longitudinal relationships between cardiovascular events, risk factors, and time-dependent sleep duration. Cardiol J. 25:229–235. DOI: 10.5603/cj.a2017.0088. PMID: 28714521.


54. Lao XQ, Liu X, Deng HB, Chan TC, Ho KF, Wang F, Vermeulen R, Tam T, Wong MCS, Tse LA, Chang LY, Yeoh EK. 2018; Sleep quality, sleep duration, and the risk of coronary heart disease: a prospective cohort study with 60,586 adults. J Clin Sleep Med. 14:109–117. DOI: 10.5664/jcsm.6894. PMID: 29198294. PMCID: PMC5734879.
55. Bertisch SM, Pollock BD, Mittleman MA, Buysse DJ, Bazzano LA, Gottlieb DJ, Redline S. 2018; Insomnia with objective short sleep duration and risk of incident cardiovascular disease and all-cause mortality: Sleep Heart Health Study. Sleep. 41:zsy047. DOI: 10.1093/sleep/zsy047. PMID: 29522193. PMCID: PMC5995202.


56. Yazdanpanah MH, Homayounfar R, Khademi A, Zarei F, Shahidi A, Farjam M. 2020; Short sleep is associated with higher prevalence and increased predicted risk of cardiovascular diseases in an Iranian population: Fasa PERSIAN Cohort Study. Sci Rep. 10:4608. DOI: 10.1038/s41598-020-61506-0. PMID: 32165672. PMCID: PMC7067883.


57. Liu Y, Wheaton AG, Chapman DP, Croft JB. 2013; Sleep duration and chronic diseases among U.S. adults age 45 years and older: evidence from the 2010 Behavioral Risk Factor Surveillance System. Sleep. 36:1421–1427. DOI: 10.5665/sleep.3028. PMID: 24082301. PMCID: PMC3773191.


58. Sabanayagam C, Shankar A. 2010; Sleep duration and cardiovascular disease: results from the National Health Interview Survey. Sleep. 33:1037–1042. DOI: 10.1093/sleep/33.8.1037. PMID: 20815184. PMCID: PMC2910533.


59. Ai S, Zhang J, Zhao G, Wang N, Li G, So HC, Liu Y, Chau SW, Chen J, Tan X, Jia F, Tang X, Shi J, Lu L, Wing YK. 2021; Causal associations of short and long sleep durations with 12 cardiovascular diseases: linear and nonlinear Mendelian randomization analyses in UK Biobank. Eur Heart J. 42:3349–3357. DOI: 10.1093/eurheartj/ehab170. PMID: 33822910.


60. Ibarra-Coronado EG, Pantaleón-Martínez AM, Velazquéz-Moctezuma J, Prospéro-García O, Méndez-Díaz M, Pérez-Tapia M, Pavón L, Morales-Montor J. 2015; The bidirectional relationship between sleep and immunity against infections. J Immunol Res. 2015:678164. DOI: 10.1155/2015/678164. PMID: 26417606. PMCID: PMC4568388.


61. Periasamy S, Hsu DZ, Fu YH, Liu MY. 2015; Sleep deprivation-induced multi-organ injury: role of oxidative stress and inflammation. EXCLI J. 14:672–683. DOI: 10.17179/excli2015-245. PMID: 26648820. PMCID: PMC4669910.
62. Jeddi S, Ghasemi A, Asgari A, Nezami-Asl A. 2018; Role of inducible nitric oxide synthase in myocardial ischemia-reperfusion injury in sleep-deprived rats. Sleep Breath. 22:353–359. DOI: 10.1007/s11325-017-1573-7. PMID: 28942508.


63. Jeddi S, Asl AN, Asgari A, Ghasemi A. 2016; The effect of sleep deprivation on cardiac function and tolerance to ischemia-reperfusion injury in male rats. Arq Bras Cardiol. 106:41–48. DOI: 10.5935/abc.20150137. PMID: 26559853. PMCID: PMC4728594.


64. Aghajani M, Faghihi M, Imani A, Vaez Mahdavi MR, Shakoori A, Rastegar T, Parsa H, Mehrabi S, Moradi F, Kazemi Moghaddam E. 2017; Post-infarct sleep disruption and its relation to cardiac remodeling in a rat model of myocardial infarction. Chronobiol Int. 34:587–600. DOI: 10.1080/07420528.2017.1281823. PMID: 28156163.


65. Carroll JE, Carrillo C, Olmstead R, Witarama T, Breen EC, Yokomizo M, Seeman T, Irwin MR. 2015; Sleep deprivation and divergent toll-like receptor-4 activation of cellular inflammation in aging. Sleep. 38:205–211. DOI: 10.5665/sleep.4398. PMID: 25325509. PMCID: PMC4288601.


66. Wadhwa M, Prabhakar A, Anand JP, Ray K, Prasad D, Kumar B, Panjwani U. 2019; Complement activation sustains neuroinflammation and deteriorates adult neurogenesis and spatial memory impairment in rat hippocampus following sleep deprivation. Brain Behav Immun. 82:129–144. DOI: 10.1016/j.bbi.2019.08.004. PMID: 31408672.


67. Korin B, Avraham S, Azulay-Debby H, Farfara D, Hakim F, Rolls A. 2020; Short-term sleep deprivation in mice induces B cell migration to the brain compartment. Sleep. 43:zsz222. DOI: 10.1093/sleep/zsz222. PMID: 31553459.


68. Tuan LH, Lee LJ. 2019; Microglia-mediated synaptic pruning is impaired in sleep-deprived adolescent mice. Neurobiol Dis. 130:104517. DOI: 10.1016/j.nbd.2019.104517. PMID: 31229687.


69. Lange T, Dimitrov S, Born J. 2010; Effects of sleep and circadian rhythm on the human immune system. Ann N Y Acad Sci. 1193:48–59. DOI: 10.1111/j.1749-6632.2009.05300.x. PMID: 20398008.


70. Said EA, Al-Abri MA, Al-Saidi I, Al-Balushi MS, Al-Busaidi JZ, Al-Reesi I, Koh CY, Idris MA, Al-Jabri AA, Habbal O. 2019; Sleep deprivation alters neutrophil functions and levels of Th1-related chemokines and CD4+ T cells in the blood. Sleep Breath. 23:1331–1339. DOI: 10.1007/s11325-019-01851-1. PMID: 31041780.


71. De Lorenzo BHP, Novaes EBRR, Paslar Leal T, Piqueira Garcia N, Martins Dos Santos RM, Alvares-Saraiva AM, Perez Hurtado EC, Braga Dos Reis TC, Duarte Palma B. 2018; Chronic sleep restriction impairs the antitumor immune response in mice. Neuroimmunomodulation. 25:59–67. DOI: 10.1159/000490352. PMID: 30007965.


72. McAlpine CS, Kiss MG, Rattik S, He S, Vassalli A, Valet C, Anzai A, Chan CT, Mindur JE, Kahles F, Poller WC, Frodermann V, Fenn AM, Gregory AF, Halle L, Iwamoto Y, Hoyer FF, Binder CJ, Libby P, Tafti M, et al. 2019; Sleep modulates haematopoiesis and protects against atherosclerosis. Nature. 566:383–387. DOI: 10.1038/s41586-019-0948-2. PMID: 30760925. PMCID: PMC6442744.


73. Kong AP, Wing YK, Choi KC, Li AM, Ko GT, Ma RC, Tong PC, Ho CS, Chan MH, Ng MH, Lau J, Chan JC. 2011; Associations of sleep duration with obesity and serum lipid profile in children and adolescents. Sleep Med. 12:659–665. DOI: 10.1016/j.sleep.2010.12.015. PMID: 21689984.


74. Ness KM, Strayer SM, Nahmod NG, Chang AM, Buxton OM, Shearer GC. 2019; Two nights of recovery sleep restores the dynamic lipemic response, but not the reduction of insulin sensitivity, induced by five nights of sleep restriction. Am J Physiol Regul Integr Comp Physiol. 316:R697–R703. DOI: 10.1152/ajpregu.00336.2018. PMID: 30892916. PMCID: PMC6620653.


75. Huang NK, Bůžková P, Matthan NR, Djoussé L, Hirsch CH, Kizer JR, Longstreth WT Jr, Mukamal KJ, Lichtenstein AH. 2021; Associations of serum nonesterified fatty acids with coronary heart disease mortality and nonfatal myocardial infarction: The CHS (Cardiovascular Health Study) Cohort. J Am Heart Assoc. 10:e019135. DOI: 10.1161/JAHA.120.019135. PMID: 33682438. PMCID: PMC8174223. PMID: 89305513c4f4404eaad2cf0c28c2d05d.


76. Xing C, Huang X, Zhang Y, Zhang C, Wang W, Wu L, Ding M, Zhang M, Song L. 2020; Sleep disturbance induces increased cholesterol level by NR1D1 mediated CYP7A1 inhibition. Front Genet. 11:610496. DOI: 10.3389/fgene.2020.610496. PMID: 33424933. PMCID: PMC7793681. PMID: 6d65576eee5a448d90f7001079a8a8e3.


77. Cretenet G, Le Clech M, Gachon F. 2010; Circadian clock-coordinated 12 Hr period rhythmic activation of the IRE1alpha pathway controls lipid metabolism in mouse liver. Cell Metab. 11:47–57. DOI: 10.1016/j.cmet.2009.11.002. PMID: 20074527.


78. Jeong SM, Choi S, Kim K, Kim SM, Lee G, Park SY, Kim YY, Son JS, Yun JM, Park SM. 2018; Effect of change in total cholesterol levels on cardiovascular disease among young adults. J Am Heart Assoc. 7:e008819. DOI: 10.1161/JAHA.118.008819. PMID: 29899019. PMCID: PMC6220545. PMID: 88ba2d4809b942458b281cac44b33bf3.


79. Wilms B, Leineweber EM, Mölle M, Chamorro R, Pommerenke C, Salinas-Riester G, Sina C, Lehnert H, Oster H, Schmid SM. 2019; Sleep loss disrupts morning-to-evening differences in human white adipose tissue transcriptome. J Clin Endocrinol Metab. 104:1687–1696. DOI: 10.1210/jc.2018-01663. PMID: 30535338.


80. Ribas-Latre A, Santos RB, Fekry B, Tamim YM, Shivshankar S, Mohamed AMT, Baumgartner C, Kwok C, Gebhardt C, Rivera A, Gao Z, Sun K, Heiker JT, Snyder BE, Kolonin MG, Eckel-Mahan KL. 2021; Publisher correction: cellular and physiological circadian mechanisms drive diurnal cell proliferation and expansion of white adipose tissue. Nat Commun. 12:4528. Erratum for: Nat Commun. 2021;12:3482. DOI: 10.1038/s41467-021-24864-5. PMID: 34285241. PMCID: PMC8292399. PMID: 35948522b3234d209efccb45c80c9f81.


81. Figueiro MG, Goo YH, Hogan R, Plitnick B, Lee JK, Jahangir K, Moulik M, Yechoor VK, Paul A. 2021; Light-dark patterns mirroring shift work accelerate atherosclerosis and promote vulnerable lesion phenotypes. J Am Heart Assoc. 10:e018151. DOI: 10.1161/JAHA.120.018151. PMID: 33401929. PMCID: PMC7955296. PMID: d0a4ad155eb8425c8c276b0c296eb5d0.


82. Everson CA, Laatsch CD, Hogg N. 2005; Antioxidant defense responses to sleep loss and sleep recovery. Am J Physiol Regul Integr Comp Physiol. 288:R374–R383. DOI: 10.1152/ajpregu.00565.2004. PMID: 15472007.


83. Trivedi MS, Holger D, Bui AT, Craddock TJA, Tartar JL. 2017; Short-term sleep deprivation leads to decreased systemic redox metabolites and altered epigenetic status. PLoS One. 12:e0181978. DOI: 10.1371/journal.pone.0181978. PMID: 28738082. PMCID: PMC5524320.


84. Jówko E, Różański P, Tomczak A. 2018; Effects of a 36-h survival training with sleep deprivation on oxidative stress and muscle damage biomarkers in young healthy men. Int J Environ Res Public Health. 15:2066. DOI: 10.3390/ijerph15102066. PMID: 30241324. PMCID: PMC6211103.


85. Valvassori SS, Resende WR, Dal-Pont G, Sangaletti-Pereira H, Gava FF, Peterle BR, Carvalho AF, Varela RB, Dal-Pizzol F, Quevedo J. 2017; Lithium ameliorates sleep deprivation-induced mania-like behavior, hypothalamic-pituitary-adrenal (HPA) axis alterations, oxidative stress and elevations of cytokine concentrations in the brain and serum of mice. Bipolar Disord. 19:246–258. DOI: 10.1111/bdi.12503. PMID: 28612976.


86. Rodrigues NR, Macedo GE, Martins IK, Gomes KK, de Carvalho NR, Posser T, Franco JL. 2018; Short-term sleep deprivation with exposure to nocturnal light alters mitochondrial bioenergetics in Drosophila. Free Radic Biol Med. 120:395–406. DOI: 10.1016/j.freeradbiomed.2018.04.549. PMID: 29655867.
87. Vosahlikova M, Roubalova L, Cechova K, Kaufman J, Musil S, Miksik I, Alda M, Svoboda P. 2020; Na+/K+-ATPase and lipid peroxidation in forebrain cortex and hippocampus of sleep-deprived rats treated with therapeutic lithium concentration for different periods of time. Prog Neuropsychopharmacol Biol Psychiatry. 102:109953. DOI: 10.1016/j.pnpbp.2020.109953. PMID: 32360816.
88. Qin B, Deng Y. 2015; Overexpression of circadian clock protein cryptochrome (CRY) 1 alleviates sleep deprivation-induced vascular inflammation in a mouse model. Immunol Lett. 163:76–83. DOI: 10.1016/j.imlet.2014.11.014. PMID: 25435215.
89. Yang L, Chu Y, Wang L, Wang Y, Zhao X, He W, Zhang P, Yang X, Liu X, Tian L, Li B, Dong S, Gao C. 2015; Overexpression of CRY1 protects against the development of atherosclerosis via the TLR/NF-κB pathway. Int Immunopharmacol. 28:525–530. DOI: 10.1016/j.intimp.2015.07.001. PMID: 26218278.


90. Schilperoort M, van den Berg R, Bosmans LA, van Os BW, Dollé MET, Smits NAM, Guichelaar T, van Baarle D, Koemans L, Berbée JFP, Deboer T, Meijer JH, de Vries MR, Vreeken D, van Gils JM, Willems van Dijk K, van Kerkhof LWM, Lutgens E, Biermasz NR, Rensen PCN, et al. 2020; Disruption of circadian rhythm by alternating light-dark cycles aggravates atherosclerosis development in APOE*3-Leiden.CETP mice. J Pineal Res. 68:e12614. DOI: 10.1111/jpi.12614. PMID: 31599473. PMCID: PMC6916424.


91. Carreras A, Zhang SX, Peris E, Qiao Z, Gileles-Hillel A, Li RC, Wang Y, Gozal D. 2014; Chronic sleep fragmentation induces endothelial dysfunction and structural vascular changes in mice. Sleep. 37:1817–1824. DOI: 10.5665/sleep.4178. PMID: 25364077. PMCID: PMC4196065.


92. Cherubini JM, Cheng JL, Williams JS, MacDonald MJ. 2021; Sleep deprivation and endothelial function: reconciling seminal evidence with recent perspectives. Am J Physiol Heart Circ Physiol. 320:H29–H35. DOI: 10.1152/ajpheart.00607.2020. PMID: 33064569.


93. Holmer BJ, Lapierre SS, Jake-Schoffman DE, Christou DD. 2021; Effects of sleep deprivation on endothelial function in adult humans: a systematic review. Geroscience. 43:137–158. DOI: 10.1007/s11357-020-00312-y. PMID: 33558966. PMCID: PMC8050211.


94. Papadakis Z, Forsse JS, Peterson MN. 2020; Acute partial sleep deprivation and high-intensity interval exercise effects on postprandial endothelial function. Eur J Appl Physiol. 120:2431–2444. DOI: 10.1007/s00421-020-04468-5. PMID: 32803383.


95. Nawi A, Eu KL, Faris ANA, Wan Ahmad WAN, Noordin L. 2020; Lipid peroxidation in the descending thoracic aorta of rats deprived of REM sleep using the inverted flowerpot technique. Exp Physiol. 105:1223–1231. DOI: 10.1113/EP088667. PMID: 32539237.


96. Sauvet F, Leftheriotis G, Gomez-Merino D, Langrume C, Drogou C, Van Beers P, Bourrilhon C, Florence G, Chennaoui M. 2010; Effect of acute sleep deprivation on vascular function in healthy subjects. J Appl Physiol (1985). 108:68–75. DOI: 10.1152/japplphysiol.00851.2009. PMID: 19910332.


97. Sauvet F, Drogou C, Bougard C, Arnal PJ, Dispersyn G, Bourrilhon C, Rabat A, Van Beers P, Gomez-Merino D, Faraut B, Leger D, Chennaoui M. 2015; Vascular response to 1 week of sleep restriction in healthy subjects. A metabolic response? Int J Cardiol. 190:246–255. DOI: 10.1016/j.ijcard.2015.04.119. PMID: 25932797.


98. Dettoni JL, Consolim-Colombo FM, Drager LF, Rubira MC, Souza SB, Irigoyen MC, Mostarda C, Borile S, Krieger EM, Moreno H Jr, Lorenzi-Filho G. 2012; Cardiovascular effects of partial sleep deprivation in healthy volunteers. J Appl Physiol (1985). 113:232–236. DOI: 10.1152/japplphysiol.01604.2011. PMID: 22539169.


99. Gutierrez-Monreal MA, Harmsen JF, Schrauwen P, Esser KA. 2020; Ticking for metabolic health: the skeletal-muscle clocks. Obesity (Silver Spring). 28(Suppl 1):S46–S54. DOI: 10.1002/oby.22826. PMID: 32468732. PMCID: PMC7381376.
100. Jagannath A, Taylor L, Wakaf Z, Vasudevan SR, Foster RG. 2017; The genetics of circadian rhythms, sleep and health. Hum Mol Genet. 26(R2):R128–R138. DOI: 10.1093/hmg/ddx240. PMID: 28977444. PMCID: PMC5886477.


101. Xie Y, Tang Q, Chen G, Xie M, Yu S, Zhao J, Chen L. 2019; New insights into the circadian rhythm and its related diseases. Front Physiol. 10:682. DOI: 10.3389/fphys.2019.00682. PMID: 31293431. PMCID: PMC6603140.


102. Jiang Q, Liu H, Wang S, Wang J, Tang Y, He Z, Wu F, Huang Z, Cong X, Ding R, Liang C. 2018; Circadian locomotor output cycles kaput accelerates atherosclerotic plaque formation by upregulating plasminogen activator inhibitor-1 expression. Acta Biochim Biophys Sin (Shanghai). 50:869–879. DOI: 10.1093/abbs/gmy087. PMID: 30124738.


103. Takeda N, Maemura K, Horie S, Oishi K, Imai Y, Harada T, Saito T, Shiga T, Amiya E, Manabe I, Ishida N, Nagai R. 2007; Thrombomodulin is a clock-controlled gene in vascular endothelial cells. J Biol Chem. 282:32561–32567. DOI: 10.1074/jbc.M705692200. PMID: 17848551.


104. Bain AR, Weil BR, Diehl KJ, Greiner JJ, Stauffer BL, DeSouza CA. 2017; Insufficient sleep is associated with impaired nitric oxide-mediated endothelium-dependent vasodilation. Atherosclerosis. 265:41–46. DOI: 10.1016/j.atherosclerosis.2017.08.001. PMID: 28846879.


105. Sauvet F, Arnal PJ, Tardo-Dino PE, Drogou C, Van Beers P, Bougard C, Rabat A, Dispersyn G, Malgoyre A, Leger D, Gomez-Merino D, Chennaoui M. 2017; Protective effects of exercise training on endothelial dysfunction induced by total sleep deprivation in healthy subjects. Int J Cardiol. 232:76–85. DOI: 10.1016/j.ijcard.2017.01.049. PMID: 28089456.


106. Stockelman KA, Bain AR, Dow CA, Diehl KJ, Greiner JJ, Stauffer BL, DeSouza CA. 2021; Regular aerobic exercise counteracts endothelial vasomotor dysfunction associated with insufficient sleep. Am J Physiol Heart Circ Physiol. 320:H1080–H1088. DOI: 10.1152/ajpheart.00615.2020. PMID: 33416458. PMCID: PMC7988760.


107. Jiang J, Gan Z, Li Y, Zhao W, Li H, Zheng JP, Ke Y. 2017; REM sleep deprivation induces endothelial dysfunction and hypertension in middle-aged rats: roles of the eNOS/NO/cGMP pathway and supplementation with L-arginine. PLoS One. 12:e0182746. DOI: 10.1371/journal.pone.0182746. PMID: 28809932. PMCID: PMC5557538.


108. Hijmans JG, Levy M, Garcia V, Lincenberg GM, Diehl KJ, Greiner JJ, Stauffer BL, DeSouza CA. 2019; Insufficient sleep is associated with a pro-atherogenic circulating microRNA signature. Exp Physiol. 104:975–982. DOI: 10.1113/EP087469. PMID: 31016755. PMCID: PMC6544492.


109. Jing R, Zhong QQ, Long TY, Pan W, Qian ZX. 2019; Downregulated miRNA-26a-5p induces the apoptosis of endothelial cells in coronary heart disease by inhibiting PI3K/AKT pathway. Eur Rev Med Pharmacol Sci. 23:4940–4947. DOI: 10.26355/eurrev_201906_18084. PMID: 31210329.
110. Nilsson EK, Boström AE, Mwinyi J, Schiöth HB. 2016; Epigenomics of total acute sleep deprivation in relation to genome-wide DNA methylation profiles and RNA expression. OMICS. 20:334–342. DOI: 10.1089/omi.2016.0041. PMID: 27310475. PMCID: PMC4926204.


111. Gaine ME, Chatterjee S, Abel T. 2018; Sleep deprivation and the epigenome. Front Neural Circuits. 12:14. DOI: 10.3389/fncir.2018.00014. PMID: 29535611. PMCID: PMC5835037.


112. Hor CN, Yeung J, Jan M, Emmenegger Y, Hubbard J, Xenarios I, Naef F, Franken P. 2019; Sleep-wake-driven and circadian contributions to daily rhythms in gene expression and chromatin accessibility in the murine cortex. Proc Natl Acad Sci U S A. 116:25773–25783. DOI: 10.1073/pnas.1910590116. PMID: 31776259. PMCID: PMC6925978.


113. Fuller PM, Eikermann M. 2019; Genomic consequences of sleep restriction: the devil is in the details. Anaesthesia. 74:417–419. DOI: 10.1111/anae.14479. PMID: 30383307.


114. Cortese R, Khalyfa A, Bao R, Andrade J, Gozal D. 2015; Epigenomic profiling in visceral white adipose tissue of offspring of mice exposed to late gestational sleep fragmentation. Int J Obes (Lond). 39:1135–1142. Erratum in: Int J Obes (Lond). 2015;39:1432. DOI: 10.1038/ijo.2015.38. PMID: 25801690. PMCID: PMC4496299.


Fig. 1
Sleep deprivation and the pathogenesis of coronary heart disease.
NR1D1, nuclear receptor subfamily 1 group D member 1; CYP7A1, cholesterol 7α-hydroxylase; IRE1α ,Inositol-requiring enzyme-1α; CCL2, chemokine C-C motif ligand 2; CCR2, chemokine C-C-motif receptor 2; CCGs, CLOCK control genes; BMAL2, brain-muscle-ARNT-like protein-2; PAI-1, plasminogen activator inhibitor-1; TM, thrombomodulin; PI3K, phosphatidyl inositol-3 kinase; Akt, protein kinase B; eNOS, endothelial nitric oxide synthase; NO, nitric oxide; cGMP, cyclic guanosine monophosphate; cAMP, cyclic adenosine monophosphate; PKA, protein kinase A; TLR, toll-like receptor; NF-κB, nuclear factor kappa B.
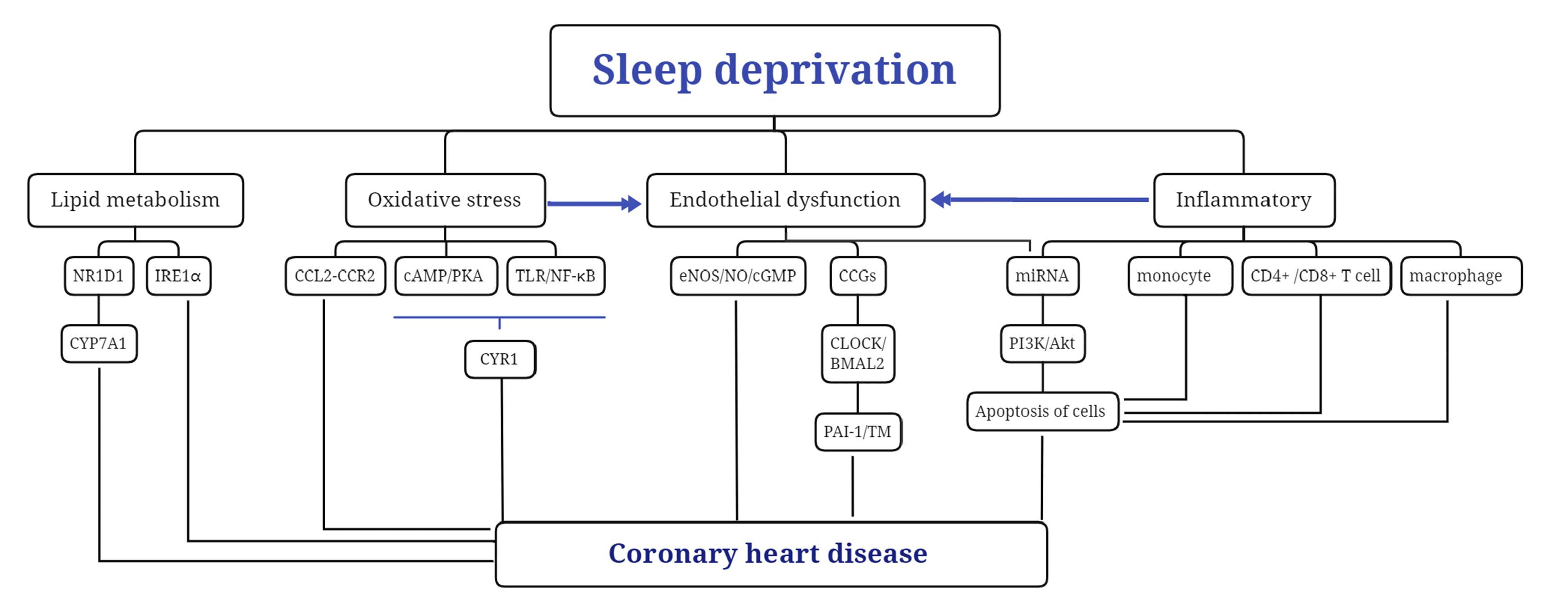
Table 1
Risk stratification and relative risk associated with sleep deprivation for coronary heart disease
Outcome | Groups | Sample size (n) | OR | 95% CI | p-value |
---|---|---|---|---|---|
Angina [58] | = 6 h | 6,369 | 1.32 | 1.04–1.67 | < 0.005 |
7 h | Ref | Ref | Ref | ||
CHD [59] | < 6 h | 15,594 | 1.24 | 1.12–1.37 | 4.09E-05 |
6–9 h | Ref | Ref | Ref | ||
MI [59] | < 6 h | 14,871 | 1.21 | 1.09–1.34 | 3.81E-04 |
6–9 h | Ref | Ref | Ref |