Abstract
Background and Objectives
Many preclinical studies have been conducted using animal disease models to determine the effectiveness of human mesenchymal stem cells (hMSCs) for treating immune and inflammatory diseases based on the belief that hMSCs are not immunogenic across species. However, several researchers have suggested xenogeneic immune responses to hMSCs in animals, still without detailed features. This study aimed to investigate a xenogeneic humoral immune response to hMSCs in mice in detail.
Methods and Results
Balb/c mice were intraperitoneally injected with adipose tissue-derived or Wharton’s jelly-derived hMSCs. Sera from these mice were titrated for each isotype. To confirm specificity of the antibodies, hMSCs were stained with the sera and subjected to a flow cytometic analysis. Spleens were immunostained for proliferating cell nuclear antigen to verify the germinal center formation. Additionally, splenocytes were subjected to a flow cytometric analysis for surface markers including GL-7, B220, CD4, CD8, CD44, and CD62L. Similar experiments were repeated in C57BL/6 mice. The results showed increased IgG1 and IgG2a titers in the sera from Balb/c mice injected with hMSCs, and the titers were much higher in the secondary sera than in the primary sera. These antibodies were specifically stained the hMSCs. Germinal centers were observed in the spleen, and flow cytometric analysis of the splenocytes showed higher frequencies of centroblasts (B220+ GL7+) and memory T cells (CD62L+ CD44+) both in CD4+ and CD8+ subsets. Similar results were obtained for C57BL/6 mice.
As multipotent, self-renewing adult stem cells, mesenchymal stem cells (MSCs) can differentiate into a variety of specialized tissue cells such as chondrocytes (1), osteocytes (2), hepatocytes (3), and neuronal cells (4), which warrants the application of these cells in regenerative medicine. Besides, MSCs also have been considered to be used in the treatment of chronic inflammatory or immune diseases such as graft-versus-host disease (5), rheumatoid arthritis (6), chronic colitis (7), and so on, because they exert anti-inflammatory and immunosuppressive activities (8).
In addition to the above-mentioned diseases, many experiments have been performed using animal disease models for many other disorders, including stroke, diabetes mellitus, septic shock, and kidney diseases. Human MSCs (hMSCs), which are xenogeneic to experimental animals, have frequently been used in many experiments to elucidate the role of hMSCs in disease, based on the belief that MSCs are not immunogenic across species; successful immunosuppressive and anti-inflammatory effects were reported (9).
However, the possibility that the exogenous MSCs, either allogeneic or xenogeneic, could yield host immune responses has been suggested (10), and some investigators have reported xenogeneic immune response to hMSCs. Specifically, when peripheral blood mononuclear cells were isolated from horses injected intra-articularly with hMSCs and co-cultured with hMSCs, cell-mediated immunity occurred with increases in the CD4+ T cells number and IL-6 concentration in the supernatant (11). Similar results were reported in ischemic heart model rats (12), both of which implied the provocation of a cellular immune response against hMSCs. Recently, Hwang et al. (13) observed that intra-cerebral injection of hMSCs induced high infiltration of CD45-positive leukocytes and argued that xenogeneic MSCs were “not poorly immunogenic” as previously noted. Concerning the humoral immune response, the sera obtained from female NZB/NZW F1 mice, a representative animal model for lupus, which were repeatedly injected with hMSCs stained positive for hMSCs based on flow cytometric analysis (14), indicating the occurrence of a humoral immune response. However, no further details have been reported.
In the present study, we clearly identified the presence of a humoral immune response against hMSCs in mice, a representative human disease model, and further characterized it in detail.
Male Balb/c and C57BL/6 (B6) mice 6∼7 weeks of age were purchased from OrientBio (Seongnam, Republic of Korea). The animals were kept at the animal facility of Seoul National University College of Medicine under specific-pathogen-free conditions. All animal experiments were approved by the IACUC of Seoul National University (SNU-170612-2).
Two types of hMSCs were used in the present study. Adipose tissue-derived MSCs (Ad-MSCs) were prepared and characterized as described previously (15). Briefly, 450 ml MSC basal medium (Lonza, Walkerville, MD, USA) was supplemented with 50 ml mesenchymal cell growth supplement (Lonza), 10 ml L-glutamine (Lonza), and 0.5 ml gentamicin sulfate (30 mg/ml) and amphotericin-B (15 μg/ml) mixture (GA-1000, Lonza), and used for Ad-MSC culture. Wharton’s jelly-derived MSCs (UC-MSCs) were gifted by professor Jo et al. (16) and maintained in low-glucose DMEM (Hyclone, South Logan, UT, USA) supplemented with 10% fetal bovine serum (Hyclone), 100 U/ml penicillin, 100 μg/ml streptomycin, and 0.25 μg/ml amphotericin (Welgene Inc., Gyeongsan, South Korea).
Characterization of the MSCs were performed previously for the expression of surface markers including CD29, CD34, CD44, CD45, CD90, CD105, and CD117 and for the lineage differentiation capacity to adipocytes, chondrocyes, and osteoblasts in our previous experiments using the same stalk of MSCs to this experiment (15, 17, 18 for Ad-MSCs and 18 for UC-MSCs), the results of which indicated that the cells were mesenchymal stem cells. For this experiment, frozen stalks of these cells from passage 5∼6 were thawed, plated at a cell density of 1×106 cells/T75 flask, and cultured until sub-confluency when the cells were harvested with trypsinization and prepared for injection.
Mice were injected intraperitoneally on days 1 and 31 with 1×106 naïve or activated Ad-MSCs or UC-MSCs from passage 7∼8 in 100 μl PBS. Sera were obtained on days 0, 10, and 38 from the orbital plexus under anesthesia, and the mice were sacrificed on day 38. The number of mice in each experimental group was eight. Another eight mice of the same age were used as the control group, which were injected only with PBS. For activation, hMSCs were treated with 10 ng/ml human IFN-γ (PeproTech, Rocky Hill, NJ, USA) for 72 h. The study protocol was approved by the Institutional Review Board of Seoul National University (SNU-J-1511-005-715).
The antibody titer in the serum was measured using ELISA. The 96-well ELISA plate was coated with goat anti-mouse total immunoglobulin (Ig; M6149, Sigma, St. Louis, MO, USA) at 3.75 μg/100 μl PBS per well and then blocked in 1% skim milk. Serum samples were duplicated, serially diluted four-fold, and incubated for 2 h at room temperature (RT). Then, the plate was incubated with alkaline phosphatase-conjugated secondary antibodies at a 1:1,000 dilution (0.5∼1 μg/100 μl PBS per well) for 1 h at RT, followed by a color reaction with 1 mg/ml p-nitrophenyl phosphate substrate (Sigma). The absorbance was measured at 405 nm using a spectrophotometer (Molecular Devices Co., Sunnyvale, CA, USA). The antibody titers in the serum were calculated relative to the values in a standard serum sample drawn from mice secondarily immunized with Keywhole limpet hemocyanin (KLH) in a previous study. In the B6 sera, IgG2c instead of IgG2a titers were measured (19). For the measurement of absolute concentrations of IgG1 and IgG2a, standards of known concentration were used: mouse IgG1, kappa (MOPC 21, Sigma, M-9269; 1 mg/ml) and anti-p-ERK (E-4) mouse monoclonal IgG2a (Santa Cruz, SC-7383; 200 μg/ml). The secondary antibodies used were as follows: goat anti-mouse Ig (H+L) (1010-04), IgG1 (1070-04), IgG2a 1080-04), IgG2b (1090-04), IgG2c (1079-04), IgG3 (1100-04), and IgM (1020-04) (all from Southern Biotech, Birmingham, AL, USA).
Half of the spleen obtained at the time of sacrifice was fixed in methacarn solution (methanol:chloroform:acetic acid=6:3:1) for 24 h, processed, and embedded in paraffin. Samples were sliced into 4-μm thick sections, deparaffinized, and treated with 3% H2O2 (in methanol) for 15 min to block endogenous peroxidases. Sections were incubated with biotinylated anti-mouse PCNA antibody (BioLegend, San Diego, CA, USA; 1:100 dilution) overnight at 4℃ and then avidin-biotinylated HRP complex (Elite/Vectastain ABC kit, PK6100, Vector Laboratories, Burlingame, CA, USA; 1:50 dilution) for 1 h at RT. The color reaction was performed using 3,3’-diaminobenzidine (D8001, Sigma) for 5 min at RT.
The other half of the spleen was minced using ground glasses. The cells were treated with RBC lysis buffer (Sigma) and washed twice with PBS to obtain the sple-nocytes. Cells were stained with 1:200 dilutions of Alexa647-anti-GL7 (561529), Bv786-anti-B220 (563894), Bv650-anti-CD4 (563232), APC-cy7-anti-CD8 (561967), PE-CD44 (553134) and/or Bv421-anti-CD62L (562910) antibodies (all from BD Biosciences, San Jose, CA, USA) for 30 min at 4℃ and subjected to flow cytometric analysis using LSRFortessa (BD Biosciences).
To confirm the presence of antibodies specific to hMSCs, naïve or activated UC-MSCs or Ad-MSCs were incubated with sera from each experimental group at a dilution of 1:100 or 1:1,000 for 1 h, at 4℃, and then with Alexa488-goat anti-mouse IgG antibody (Invitrogen, Camarillo, CA, USA; 1:200 dilution). The stained cells were analyzed on the BD LSRFortessaTM (BD Biosciences) instrument using FACSDivaTM software, and the data were analyzed using FlowJoⓇ software (TreeStar, Ashland, OR, USA).
In sera of Balb/c mice obtained 10 days after the first injection of hMSCs (i.e., primary serum), the total Ig titer was increased in all experimental groups (Fig. 1), even though only the naive Ad-MSC-injected group secured statistical significance compared to the control group. This increase was due mainly to increased IgG1 and IgG2a titers. IgG2b and IgG3 titers in the experimental groups did not change compared with the control. The IgM titer was decreased compared with the control but not significantly.
After the second injection, the serum (i.e., secondary serum) total Ig titer was increased (Fig. 2A). Similar to the primary serum, this was due to increases in the IgG1 and IgG2a titers, and the other isotype antibodies did not show any significant changes (data not shown). The absolute concentrations of the IgG2a and IgG1 titers were increased approximately 6- and 54-fold, respectively, compared with the control (Fig. 2B, Table 1).
Even though the titers of total Ig, IgG1, and IgG2a were increased in all experimental groups compared to the control group, some lacked statistical significance. Thus, we need to verify whether specific antibodies to the injected hMSCs were developed in the mice. For this purpose, we stained hMSCs with the secondary sera from each experimental group and analyzed using flow cytometry. The sera from all experimental groups were positive for both UC-MSCs and Ad-MSCs, irrespective of naïve or activated (Fig. 3). Meanwhile, the control sera minimally stained the cells (Supplementary Fig. S1), which indicated the specificity of the staining by the experimental sera. Notably, although sera from mice injected with naïve hMSCs showed similar staining intensities between naïve and activated hMSCs, sera from mice injected with activated hMSCs showed more intense staining in the activated than naïve cells (Table 2). This tendency was more prominent in the UC-MSC-injected groups (143.2% in UC-MSCs, p=0.046; 120.4% in Ad-MSCs, p=0.208).
In addition to the increase of serum antibody titers, immunohistochemical staining of PCNA, which is present in dividing cells, in the spleen showed germinal centers in hMSC-injected Balb/c mice (Fig. 4). However, no difference in the frequency of germinal centers between the Ad-MSC- and UC-MSC-injected groups was observed. In addition, flow cytometric analysis of splenocytes showed an increased frequency of GL7+ germinal center B cells at the time of sacrifice (Supplementary Fig. S2). In both CD4+ and CD8+ T cells, the frequencies of effector (CD62L− CD44+) and memory cells (CD62L+CD44+) were increased (Supplementary Fig. S3 and S4). However, no difference was observed between the Ad-MSC- and UC-MSC-injected groups.
In addition to Balb/c mice, similar experiments were performed in B6 mice; however, only secondary sera were analyzed. ELISA showed increased titers of total Ig, IgG1, and IgG2c in all groups compared with the control, similar to the Balb/c mice. The titers of both isotypes were increased similarly compared with the control (Table 3). In contrast to the Blab/c mice, the IgM titer was also increased in all experimental groups (Fig. 5). The sera stained hMSCs similar to the sera from Balb/c mice.
In the present study, it was investigated whether hMSCs elicit xenogeneic humoral immune responses when injected into mice. In Balb/c mice, injection of hMSCs increased the IgG1 and IgG2a titers, which were higher in the secondary sera than in the primary sera. The sera stained hMSCs, as revealed by flow cytometric analysis, indicating that the increased antibodies were specific to hMSCs. Additionally, the frequency of GL7+ centroblasts and PCNA immunostaining showed germinal center formation in the spleen. When the same experiments were performed in B6 mice, similar results were obtained.
Both in Balb/c and B6 mice, IgG1 and IgG2a (IgG2c instead of IgG2a in B6 mice) were increased among the Ig isotypes evaluated. However, a difference in the profiles was observed between the two strains. In the secondary sera of Balb/c mice, the IgG1 titer increased significantly more, from 54- to 250-fold relative to the control group, than did the IgG2a titer (approximately 6-fold) (Table 1). However, the fold increases in the two isotypes were similar in B6 mice (Table 3). It is well known that IFN-γ, a Th1 type cytokine, and IL-4, a Th2 type cytokine, reciprocally drive the production of IgG2a and IgG1; thus the isotypes represent Th1 and Th2 humoral immune responses, respectively (20). Also known is that Balb/c and B6 mice are Th2 and Th1 prone, respectively (21). Thus, the humoral immune response in mice against hMSCs followed the Th1/Th2 paradigm. These results, together with the exaggerated secondary response and the germinal center formation reflect the characteristics of T-cell-dependent responses.
At least, several molecules expressed on the hMSC surface likely acted as xenogeneic antigens. The results that the sera surface-stained hMSCs (Fig. 3) support this assumption. Furthermore, sera from mice injected with naïve hMSC showed similar staining between naïve and activated hMSCs, whereas sera from mice injected with activated hMSCs showed more intense staining in activated than in the naïve hMSCs (Fig. 3, Supplementary Fig. S2, Table 2), which can be interpreted as that new proteins had expressed on the cell surface of hMSCs following activation and acted as additional antigens. In fact, IFN-γ, used for hMSC activation in the present study, promotes the expression of several surface molecules such as HLA-ABC and HLA-DR and secretory molecules in Ad-MSCs (18), B7-H1 in UC-MSCs (22), and ICAM-1 and VCAM-1 in Ad- and UC-MSCs (23).
For the production of antibodies to T-cell-dependent antigens, dendritic cells (DCs) engulf the antigens and present them to antigen-specific T cells, which then activate and help antigen-specific B cells proliferate and differentiate into plasma cells to secrete antigen-specific antibodies. MSCs across the species suppressively affect all of the players in this process, including DCs, T cells, and B cells. For example, MSCs inhibited the differentiation of DCs from their CD34+ progenitor cells or monocytes (24). The inhibited DCs expressed decreased levels of surface molecules such as CD86, CD83, CD80, HLA-DR, and CD40. MSCs administered in vivo downregulated expression of CCR7 and CD49dβ1 in DCs, thus inhibiting the homing of these cells to lymph nodes (25). hMSCs inhibited murine T-cell proliferation by secreting cyclooxygenase-2 (15). Regarding B cells, MSCs arrested the cell cycle in these cells (26) and inhibited differentiation of the cells into plasma cells (27). Thus, it would be natural to assume that a humoral immune response against hMSCs would not occur. However, the results of the present study are contradictory to this assumption. In fact, this “contradiction” has continuously been suggested by previous reports; cellular immune responses against intra-articularly injected hMSCs in horses (11) and intra-myocardially injected hMSCs in rats (12), local leukocyte infiltration at the injection site of hMSC in the putamen of mice (13). Repeated intravenous injection of hMSCs in female NZB/NZW F1 mice elicited antibody formation specific for the xenogeneic MSCs, as well as beneficial effects of delaying the onset of the disease (14). These contradictory results need to be explained, yet no explanation has been suggested in the previous reports. Unfortunately, we also have no experimental data to explain this apparent discrepancy.
Presumably, the activation status of the hMSCs could matter. MSCs exert immunomodulatory effects only when they are activated by pro-inflammatory cytokines such as IFN-γ (28), TNF-α (29), and IL-1β (30) in an inflammatory environment, and the naïve hMSCs injected into healthy mice might fail to exert immunomodulatory effects because they cannot be activated in the absence of inflammation. However, in the present study, even the ex-vivo IFN-γ-activated hMSCs did not show an immunosuppressive effect, similar to the naïve MSCs. Thus, activation status of the injected hMSCs was apparently not relevant.
Another concern is the incompatibility of cytokines or other factors that are needed for immunosuppression between humans and mice. For example, mouse IFN-γ, which is a principal cytokine for MSC priming, is not compatible with humans (15). Meanwhile, hMSC express indoleamine 2,3-dioxygenase (IDO) (31) and cyclooxyge-nase-2 (COX-2) (32), and these enzymes are important for immune modulation such as inhibition of T cell proli-feration. IDO metabolizes tryptophan and its metabolites such as kynurenine, 3-hydroxykynurenine, and 3-hydroxyanthranilic acid is known to inhibit the proliferation of T cells (33). COX-2 exhibits its action by producing PGE2 (32). Both of these enzymes have been reported to be produced also in mouse MSCs and used for immune modulation (34). Altogether, human IL-10 and TGF-β, the major effector cytokines responsible for the immunosupp-ressive function of MSCs, act on mouse cells (35, 36). Thus, cytokine incompatibility between humans and mice does not appear to be a major cause of the failed suppression of the humoral immune response.
The number of live hMSCs in mouse lymphoid organs can be another possible explanation. In vitro inhibition of T-cell proliferation by hMSCs is apparent at a 1:10 ratio of MSCs to T cells; however, the effect is dose-dependent and becomes negligible at a 1:400 ratio (15, 20). Additionally, activated MSCs need proximity to affect B cells (37). Thus, a significant number of MSCs should be concentrated in lymphoid organs to exert their immunosuppressive effects. Although the number of hMSCs that reached the spleen and regional lymph nodes is unknown, we can surmise that the number is not substantial based on previous reports; hMSCs injected into normal mice or rats may or may not reach the spleen depending on the administration route, and even MSCs have reached the spleen, the frequency is low or very low (38). Another concern is the viability of hMSCs injected into mice. Even though some investigators reported long-term survival of hMSCs in mice, also reported are that majority of hMSCs disappear within 1∼2 weeks after injection into mice, regardless of the administration route (39, 40). Therefore, it is reasonable to assume that many of the administered hMSCs die early instead they accumulate in lymphoid organs. Thus, it is anticipated that the number of live hMSCs in organs such as the spleen and lymph nodes are not enough to suppress the humoral immunes response.
The limitation of this study is that experiments have not been performed in disease animal models with vivid inflammation, in which the immune milieu could be different from those in healthy animals and thus the processes of humoral immune response might differ. It should be further elucidated whether the phenomena observed in this study would be recapitulated when hMSCs are administered in these animals.
In conclusion, although many preclinical studies in disease animal models have shown that hMSCs suppressed the immune and inflammatory responses of host animals, the results of the present study showed that hMSCs administered in healthy mice induced a strong humoral immune response characterized by typical T cell-dependent immunity. These results should be considered when designing preclinical experiments using hMSCs and interpreting the results from them, especially with repeated injections of hMSCs.
Supplementary data including four figures can be found with this article online at https://doi.org/10.15283/ijsc21116.
Acknowledgments
This research was supported exclusively by the Bio & Medical Technology Development Program of the National Research Foundation (NRF) funded by the Ministry of Science, ICT & Future Planning (NRF-2015M3A9E6028677). No any other financial sources support this study.
References
1. To K, Zhang B, Romain K, Mak C, Khan W. 2019; Synovium-derived mesenchymal stem cell transplantation in cartilage regeneration: a PRISMA review of in vivo studies. Front Bioeng Biotechnol. 7:314. DOI: 10.3389/fbioe.2019.00314. PMID: 31803726. PMCID: PMC6873960. PMID: https://www.scopus.com/inward/record.uri?partnerID=HzOxMe3b&scp=85076040986&origin=inward.
2. Ansari AS, Yazid MD, Sainik NQAV, Razali RA, Saim AB, Idrus RBH. 2018; Osteogenic induction of Wharton's jelly-de-rived mesenchymal stem cell for bone regeneration: a systematic review. Stem Cells Int. 2018:2406462. DOI: 10.1155/2018/2406462. PMID: 30534156. PMCID: PMC6252214. PMID: https://www.scopus.com/inward/record.uri?partnerID=HzOxMe3b&scp=85062864268&origin=inward.
3. Hu C, Zhao L, Li L. 2019; Current understanding of adipose-derived mesenchymal stem cell-based therapies in liver diseases. Stem Cell Res Ther. 10:199. DOI: 10.1186/s13287-019-1310-1. PMID: 31287024. PMCID: PMC6613269. PMID: b0bb20371bbc401398e771d293aea5f9. PMID: https://www.scopus.com/inward/record.uri?partnerID=HzOxMe3b&scp=85068928532&origin=inward.
4. Cofano F, Boido M, Monticelli M, Zenga F, Ducati A, Vercelli A, Garbossa D. 2019; Mesenchymal stem cells for spinal cord injury: current options, limitations, and future of cell therapy. Int J Mol Sci. 20:2698. DOI: 10.3390/ijms20112698. PMID: 31159345. PMCID: PMC6600381. PMID: https://www.scopus.com/inward/record.uri?partnerID=HzOxMe3b&scp=85067174633&origin=inward.
5. Le Blanc K, Rasmusson I, Sundberg B, Götherström C, Hassan M, Uzunel M, Ringdén O. 2004; Treatment of severe acute graft-versus-host disease with third party haploiden-tical mesenchymal stem cells. Lancet. 363:1439–1441. DOI: 10.1016/S0140-6736(04)16104-7. PMID: 15121408. PMID: https://www.scopus.com/inward/record.uri?partnerID=HzOxMe3b&scp=2342482526&origin=inward.
6. Álvaro-Gracia JM, Jover JA, García-Vicuña R, Carreño L, Alonso A, Marsal S, Blanco F, Martínez-Taboada VM, Taylor P, Martín-Martín C, DelaRosa O, Tagarro I, Díaz-González F. 2017; Intravenous administration of expanded allogeneic adipose-derived mesenchymal stem cells in refractory rheumatoid arthritis (Cx611): results of a multicentre, dose escalation, randomised, single-blind, placebo-controlled phase Ib/IIa clinical trial. Ann Rheum Dis. 76:196–202. DOI: 10.1136/annrheumdis-2015-208918. PMID: 27269294. PMID: https://www.scopus.com/inward/record.uri?partnerID=HzOxMe3b&scp=84973304693&origin=inward.
7. Zhang J, Lv S, Liu X, Song B, Shi L. 2018; Umbilical cord mesenchymal stem cell treatment for Crohn's disease: a randomized controlled clinical trial. Gut Liver. 12:73–78. DOI: 10.5009/gnl17035. PMID: 28873511. PMCID: PMC5753687. PMID: https://www.scopus.com/inward/record.uri?partnerID=HzOxMe3b&scp=85040510160&origin=inward.
8. Schepers K, Fibbe WE. 2016; Unraveling mechanisms of mesenchymal stromal cell-mediated immunomodulation through patient monitoring and product characterization. Ann N Y Acad Sci. 1370:15–23. DOI: 10.1111/nyas.12984. PMID: 26713608. PMID: https://www.scopus.com/inward/record.uri?partnerID=HzOxMe3b&scp=84976617562&origin=inward.
9. Prockop DJ, Oh JY, Lee RH. 2017; Data against a common assumption: xenogeneic mouse models can be used to assay suppression of immunity by human MSCs. Mol Ther. 25:1748–1756. DOI: 10.1016/j.ymthe.2017.06.004. PMID: 28647464. PMCID: PMC5542802. PMID: https://www.scopus.com/inward/record.uri?partnerID=HzOxMe3b&scp=85021175505&origin=inward.
10. Buja LM, Vela D. 2010; Immunologic and inflammatory reactions to exogenous stem cells implications for experimen-tal studies and clinical trials for myocardial repair. J Am Coll Cardiol. 56:1693–1700. DOI: 10.1016/j.jacc.2010.06.041. PMID: 21070919. PMID: https://www.scopus.com/inward/record.uri?partnerID=HzOxMe3b&scp=78349245568&origin=inward.
11. Pigott JH, Ishihara A, Wellman ML, Russell DS, Bertone AL. 2013; Investigation of the immune response to autologous, allogeneic, and xenogeneic mesenchymal stem cells after intra-articular injection in horses. Vet Immunol Immuno-pathol. 156:99–106. DOI: 10.1016/j.vetimm.2013.09.003. PMID: 24094688. PMID: https://www.scopus.com/inward/record.uri?partnerID=HzOxMe3b&scp=84887050176&origin=inward.
12. Grinnemo KH, Månsson A, Dellgren G, Klingberg D, Wardell E, Drvota V, Tammik C, Holgersson J, Ringdén O, Sylvén C, Le Blanc K. 2004; Xenoreactivity and engraftment of human mesenchymal stem cells transplanted into infarcted rat myocardium. J Thorac Cardiovasc Surg. 127:1293–1300. DOI: 10.1016/j.jtcvs.2003.07.037. PMID: 15115985. PMID: https://www.scopus.com/inward/record.uri?partnerID=HzOxMe3b&scp=2342427573&origin=inward.
13. Hwang JW, Lee NK, Yang JH, Son HJ, Bang SI, Chang JW, Na DL. 2020; A comparison of immune responses exerted following syngeneic, allogeneic, and xenogeneic transplantation of mesenchymal stem cells into the mouse brain. Int J Mol Sci. 21:3052. DOI: 10.3390/ijms21093052. PMID: 32357509. PMCID: PMC7246520. PMID: dffeb7a1acc34da481e4611252b44e91. PMID: https://www.scopus.com/inward/record.uri?partnerID=HzOxMe3b&scp=85084062470&origin=inward.
14. Choi EW, Lee HW, Shin IS, Park JH, Yun TW, Youn HY, Kim SJ. 2016; Comparative efficacies of long-term serial transplantation of syngeneic, allogeneic, xenogeneic, or CTLA4Ig-overproducing xenogeneic adipose tissue-derived mesenchymal stem cells on murine systemic lupus erythematosus. Cell Transplant. 25:1193–1206. DOI: 10.3727/096368915X689442. PMID: 26377835. PMID: https://www.scopus.com/inward/record.uri?partnerID=HzOxMe3b&scp=84973168502&origin=inward.
15. Kim JH, Lee YT, Hong JM, Hwang YI. 2013; Suppression of in vitro murine T cell proliferation by human adipose tissue-derived mesenchymal stem cells is dependent mainly on cyclooxygenase-2 expression. Anat Cell Biol. 46:262–271. DOI: 10.5115/acb.2013.46.4.262. PMID: 24386599. PMCID: PMC3875844.
16. Jo CH, Kim OS, Park EY, Kim BJ, Lee JH, Kang SB, Lee JH, Han HS, Rhee SH, Yoon KS. 2008; Fetal mesenchymal stem cells derived from human umbilical cord sustain primitive characteristics during extensive expansion. Cell Tissue Res. 334:423–433. DOI: 10.1007/s00441-008-0696-3. PMID: 18941782. PMID: https://www.scopus.com/inward/record.uri?partnerID=HzOxMe3b&scp=59449088430&origin=inward.
17. Kim JH, Lee YT, Oh K, Cho J, Lee DS, Hwang YI. 2014; Paradoxical effects of human adipose tissue-derived mesenchymal stem cells on progression of experimental arthritis in SKG mice. Cell Immunol. 292:94–101. DOI: 10.1016/j.cellimm.2014.10.005. PMID: 25460084. PMID: https://www.scopus.com/inward/record.uri?partnerID=HzOxMe3b&scp=84911395004&origin=inward.
18. Kim JH, Jo CH, Kim HR, Hwang YI. 2018; Comparison of immunological characteristics of mesenchymal stem cells from the periodontal ligament, umbilical cord, and adipose tissue. Stem Cells Int. 2018:8429042. DOI: 10.1155/2018/8429042. PMID: 29760736. PMCID: PMC5901833. PMID: https://www.scopus.com/inward/record.uri?partnerID=HzOxMe3b&scp=85056141943&origin=inward.
19. Martin RM, Brady JL, Lew AM. 1998; The need for IgG2c specific antiserum when isotyping antibodies from C57BL/6 and NOD mice. J Immunol Methods. 212:187–192. DOI: 10.1016/S0022-1759(98)00015-5. PMID: 9672206. PMID: https://www.scopus.com/inward/record.uri?partnerID=HzOxMe3b&scp=0032520727&origin=inward.
20. Stevens TL, Bossie A, Sanders VM, Fernandez-Botran R, Coffman RL, Mosmann TR, Vitetta ES. 1988; Regulation of antibody isotype secretion by subsets of antigen-specific helper T cells. Nature. 334:255–258. DOI: 10.1038/334255a0. PMID: 2456466. PMID: https://www.scopus.com/inward/record.uri?partnerID=HzOxMe3b&scp=0023733838&origin=inward.
21. Mills CD, Kincaid K, Alt JM, Heilman MJ, Hill AM. 2000; M-1/M-2 macrophages and the Th1/Th2 paradigm. J Immunol. 164:6166–6173. DOI: 10.4049/jimmunol.164.12.6166. PMID: 10843666. PMID: https://www.scopus.com/inward/record.uri?partnerID=HzOxMe3b&scp=0034659784&origin=inward.
22. Jang IK, Jung HJ, Noh OK, Lee DH, Lee KC, Park JE. 2018; B7‑H1‑mediated immunosuppressive properties in human mesenchymal stem cells are mediated by STAT‑1 and not PI3K/Akt signaling. Mol Med Rep. 18:1842–1848. DOI: 10.3892/mmr.2018.9102. PMID: 29901104. PMID: https://www.scopus.com/inward/record.uri?partnerID=HzOxMe3b&scp=85049589022&origin=inward.
23. Wang Q, Yang Q, Wang Z, Tong H, Ma L, Zhang Y, Shan F, Meng Y, Yuan Z. 2016; Comparative analysis of human mesenchymal stem cells from fetal-bone marrow, adipose tissue, and Warton's jelly as sources of cell immunomo-dulatory therapy. Hum Vaccin Immunother. 12:85–96. DOI: 10.1080/21645515.2015.1030549. PMID: 26186552. PMCID: PMC4962749. PMID: https://www.scopus.com/inward/record.uri?partnerID=HzOxMe3b&scp=84961827503&origin=inward.
24. Nauta AJ, Kruisselbrink AB, Lurvink E, Willemze R, Fibbe WE. 2006; Mesenchymal stem cells inhibit generation and function of both CD34+-derived and monocyte-derived dendritic cells. J Immunol. 177:2080–2087. DOI: 10.4049/jimmunol.177.4.2080. PMID: 16887966. PMID: https://www.scopus.com/inward/record.uri?partnerID=HzOxMe3b&scp=33746898242&origin=inward.
25. Chiesa S, Morbelli S, Morando S, Massollo M, Marini C, Bertoni A, Frassoni F, Bartolomé ST, Sambuceti G, Traggiai E, Uccelli A. 2011; Mesenchymal stem cells impair in vivo T-cell priming by dendritic cells. Proc Natl Acad Sci U S A. 108:17384–17389. DOI: 10.1073/pnas.1103650108. PMID: 21960443. PMCID: PMC3198360. PMID: https://www.scopus.com/inward/record.uri?partnerID=HzOxMe3b&scp=80054809361&origin=inward.
26. Corcione A, Benvenuto F, Ferretti E, Giunti D, Cappiello V, Cazzanti F, Risso M, Gualandi F, Mancardi GL, Pistoia V, Uccelli A. 2006; Human mesenchymal stem cells modulate B-cell functions. Blood. 107:367–372. DOI: 10.1182/blood-2005-07-2657. PMID: 16141348. PMID: https://www.scopus.com/inward/record.uri?partnerID=HzOxMe3b&scp=30144440925&origin=inward.
27. Franquesa M, Mensah FK, Huizinga R, Strini T, Boon L, Lombardo E, DelaRosa O, Laman JD, Grinyó JM, Weimar W, Betjes MG, Baan CC, Hoogduijn MJ. 2015; Human adipose tissue-derived mesenchymal stem cells abrogate plasmablast formation and induce regulatory B cells indepen-dently of T helper cells. Stem Cells. 33:880–891. DOI: 10.1002/stem.1881. PMID: 25376628. PMID: https://www.scopus.com/inward/record.uri?partnerID=HzOxMe3b&scp=84923217704&origin=inward.
28. Kim DS, Jang IK, Lee MW, Ko YJ, Lee DH, Lee JW, Sung KW, Koo HH, Yoo KH. 2018; Enhanced immunosuppressive properties of human mesenchymal stem cells primed by interferon-γ. EBioMedicine. 28:261–273. DOI: 10.1016/j.ebiom.2018.01.002. PMID: 29366627. PMCID: PMC5898027. PMID: https://www.scopus.com/inward/record.uri?partnerID=HzOxMe3b&scp=85040649839&origin=inward.
29. Putra A, Ridwan FB, Putridewi AI, Kustiyah AR, Wirastuti K, Sadyah NAC, Rosdiana I, Munir D. 2018; The role of TNF-α induced MSCs on suppressive inflammation by increasing TGF-β and IL-10. Open Access Maced J Med Sci. 6:1779–1783. DOI: 10.3889/oamjms.2018.404. PMID: 30455748. PMCID: PMC6236029. PMID: https://www.scopus.com/inward/record.uri?partnerID=HzOxMe3b&scp=85058173547&origin=inward.
30. Wu TY, Liang YH, Wu JC, Wang HS. 2019; Interleukin-1β enhances umbilical cord mesenchymal stem cell adhesion ability on human umbilical vein endothelial cells via LFA-1/ICAM-1 interaction. Stem Cells Int. 2019:7267142. DOI: 10.1155/2019/7267142. PMID: 31949440. PMCID: PMC6948307. PMID: 8df63d2d28d34ace8d0deb3bd318de94. PMID: https://www.scopus.com/inward/record.uri?partnerID=HzOxMe3b&scp=85077839153&origin=inward.
31. Meisel R, Zibert A, Laryea M, Göbel U, Däubener W, Dilloo D. 2004; Human bone marrow stromal cells inhibit allogeneic T-cell responses by indoleamine 2,3-dioxygenase-mediated tryptophan degradation. Blood. 103:4619–4621. DOI: 10.1182/blood-2003-11-3909. PMID: 15001472. PMID: https://www.scopus.com/inward/record.uri?partnerID=HzOxMe3b&scp=2942595706&origin=inward.
32. Aggarwal S, Pittenger MF. 2005; Human mesenchymal stem cells modulate allogeneic immune cell responses. Blood. 105:1815–1822. DOI: 10.1182/blood-2004-04-1559. PMID: 15494428. PMID: https://www.scopus.com/inward/record.uri?partnerID=HzOxMe3b&scp=13544249606&origin=inward.
33. Bauer TM, Jiga LP, Chuang JJ, Randazzo M, Opelz G, Terness P. 2005; Studying the immunosuppressive role of indoleamine 2,3-dioxygenase: tryptophan metabolites suppress rat allogeneic T-cell responses in vitro and in vivo. Transpl Int. 18:95–100. DOI: 10.1111/j.1432-2277.2004.00031.x. PMID: 15612990. PMID: https://www.scopus.com/inward/record.uri?partnerID=HzOxMe3b&scp=21244487758&origin=inward.
34. English K, Barry FP, Field-Corbett CP, Mahon BP. 2007; IFN-gamma and TNF-alpha differentially regulate immunomodulation by murine mesenchymal stem cells. Immunol Lett. 110:91–100. DOI: 10.1016/j.imlet.2007.04.001. PMID: 17507101. PMID: https://www.scopus.com/inward/record.uri?partnerID=HzOxMe3b&scp=34249662219&origin=inward.
35. Massagué J, Like B. 1985; Cellular receptors for type beta transforming growth factor. Ligand binding and affinity labeling in human and rodent cell lines. J Biol Chem. 260:2636–2645. DOI: 10.1016/S0021-9258(18)89408-X. PMID: 2982829.
36. Tan JC, Indelicato SR, Narula SK, Zavodny PJ, Chou CC. 1993; Characterization of interleukin-10 receptors on human and mouse cells. J Biol Chem. 268:21053–21059. DOI: 10.1016/S0021-9258(19)36892-9. PMID: 8407942.
37. Luk F, Carreras-Planella L, Korevaar SS, de Witte SFH, Borràs FE, Betjes MGH, Baan CC, Hoogduijn MJ, Franquesa M. 2017; Inflammatory conditions dictate the effect of mesenchymal stem or stromal cells on B cell function. Front Immunol. 8:1042. DOI: 10.3389/fimmu.2017.01042. PMID: 28894451. PMCID: PMC5581385. PMID: https://www.scopus.com/inward/record.uri?partnerID=HzOxMe3b&scp=85028442641&origin=inward.
38. Leibacher J, Henschler R. 2016; Biodistribution, migration and homing of systemically applied mesenchymal stem/stromal cells. Stem Cell Res Ther. 7:7. DOI: 10.1186/s13287-015-0271-2. PMID: 26753925. PMCID: PMC4709937. PMID: https://www.scopus.com/inward/record.uri?partnerID=HzOxMe3b&scp=84954101786&origin=inward.
39. Braid LR, Wood CA, Wiese DM, Ford BN. 2018; Intramuscular administration potentiates extended dwell time of mesenchymal stromal cells compared to other routes. Cytotherapy. 20:232–244. DOI: 10.1016/j.jcyt.2017.09.013. PMID: 29167063. PMID: https://www.scopus.com/inward/record.uri?partnerID=HzOxMe3b&scp=85034578828&origin=inward.
40. Toupet K, Maumus M, Peyrafitte JA, Bourin P, van Lent PL, Ferreira R, Orsetti B, Pirot N, Casteilla L, Jorgensen C, Noël D. 2013; Long-term detection of human adipose-derived mesenchymal stem cells after intraarticular injection in SCID mice. Arthritis Rheum. 65:1786–1794. DOI: 10.1002/art.37960. PMID: 23553439.
Fig. 1
Elevated serum immunoglobulin titers after primary injection of human MSCs. Mouse blood was drawn 10 days after intraperitoneal injection of human MSCs and sera were obtained. Titration was done by ELISA using a standard serum which had been drawn from secondarily immunized mice with KLH in previous studies. *p<0.05 and **p<0.01 vs. the control.
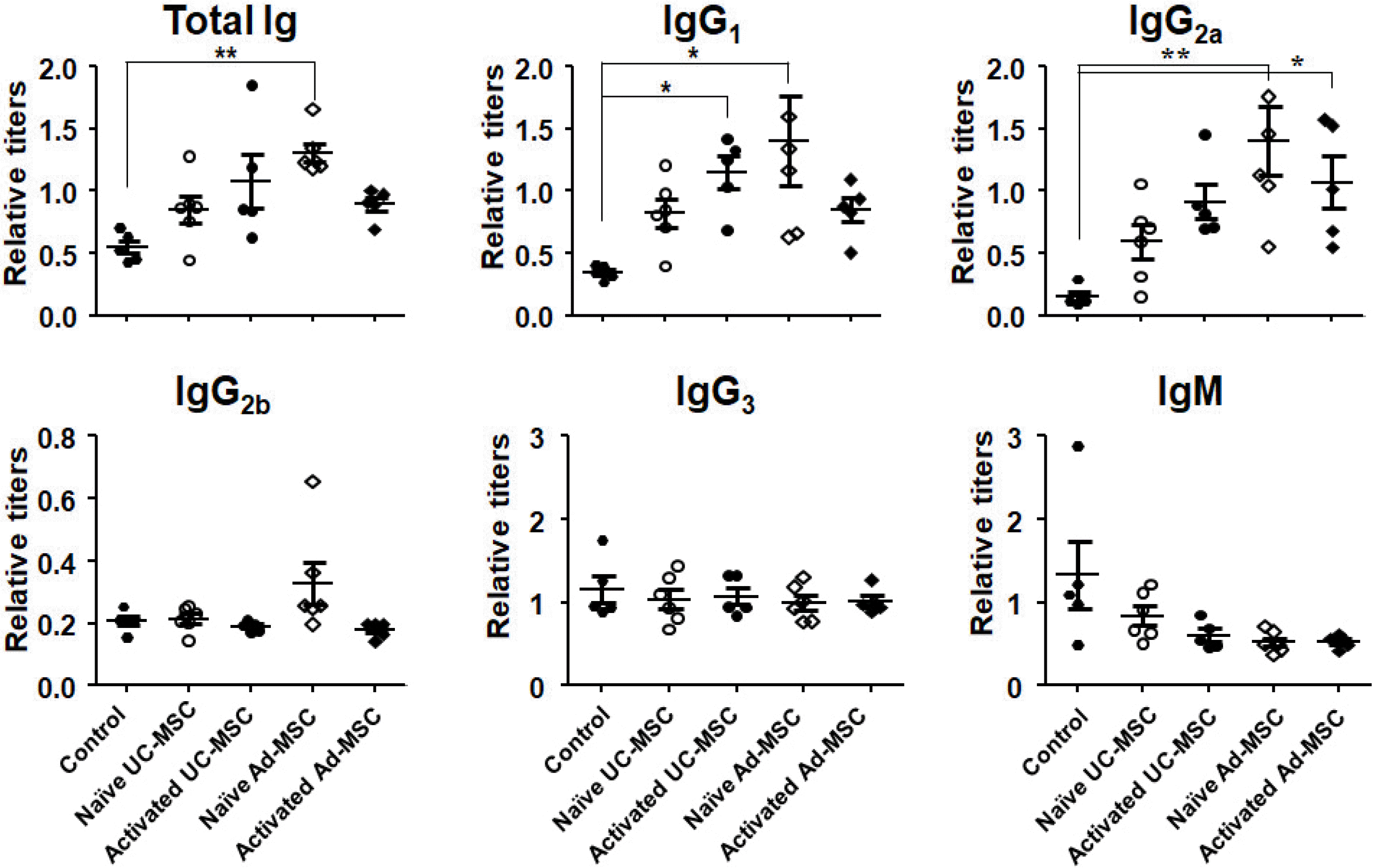
Fig. 2
Elevated serum immunoglobulin titers after secondary injection of human MSCs. Mice were injected with human MSCs on days 1 and 31, and blood samples were obtained seven days after the second injection. ELISA was performed with each sample duplicated using (A) KLH-immunized serum or (B) purified mouse IgG1 or IgG2a antibodies of known concentration as a standard. *p<0.05, **p<0.01, and ***p<0.001 vs. the control.
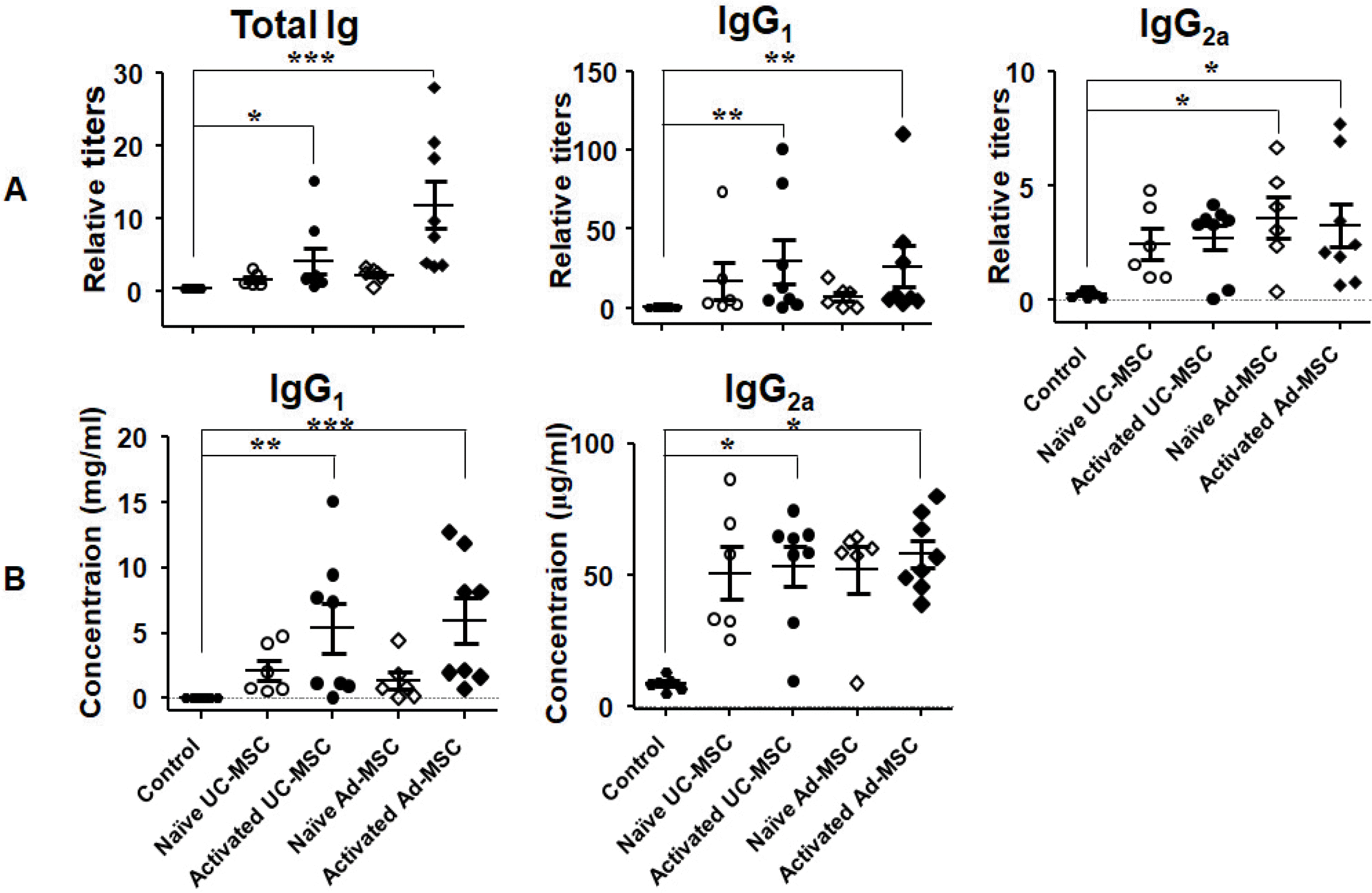
Fig. 3
Surface staining of human MSCs using mice sera. Human UC-MSCs, either naïve or activated, were stained with secondary sera from mice injected with naïve or activated hUC-MSCs (A). Likewise, hAd-MSCs, either naïve or activated, were stained with secondary sera from mice injected with naïve or activated hAd-MSCs (B). Stained cells were analyzed by flow cytometry. Colored graphs represent those stained with each serum, and the black one represents that stained only with a secondary serum. The mean median fluorescent intensity (MFI) values are summarized in Table 2.
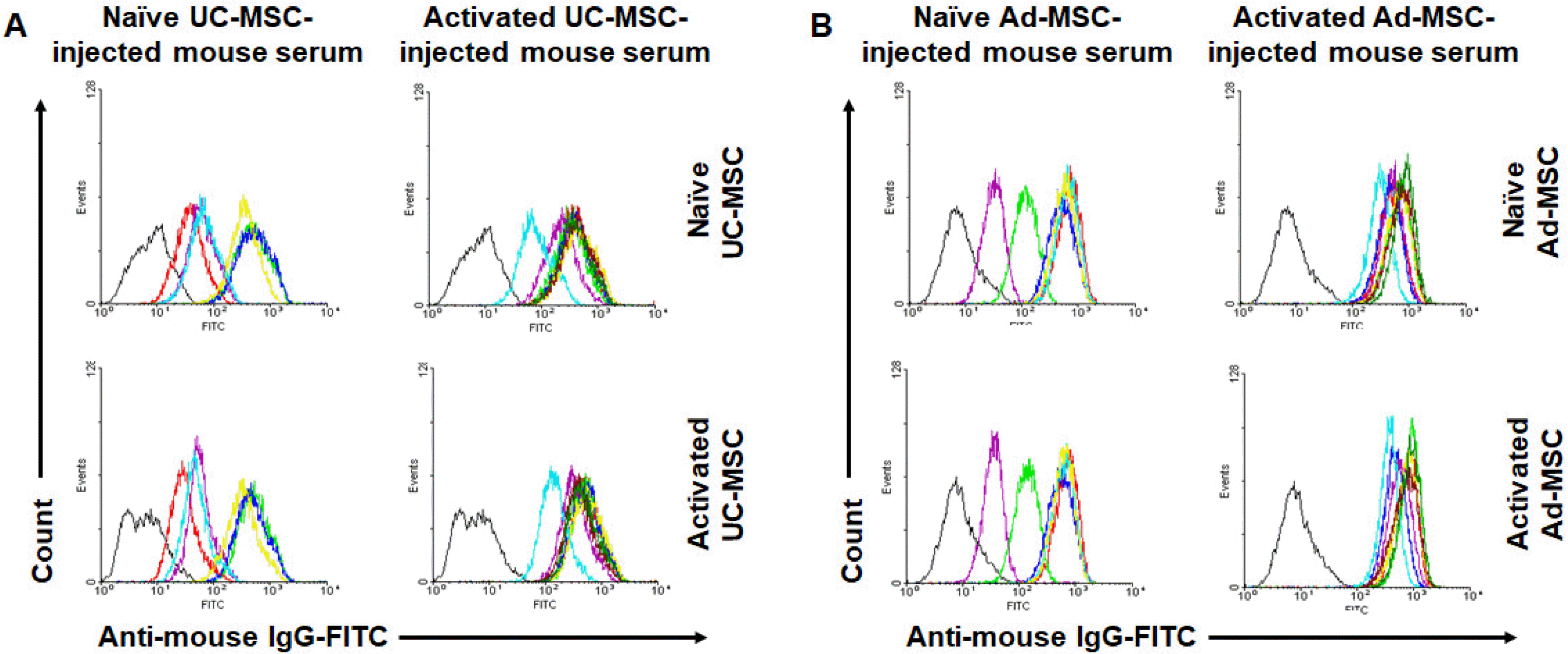
Fig. 4
Germinal center formation in the mouse spleen. Spleens from each group of second injection experiment were immuno-stained for proliferating cell nuclear antigen, which stained proliferating B cells in the germinal centers. All the spleens show germinal centers (arrows), but no differences in the frequency were observed among groups. Magnification; ×100.
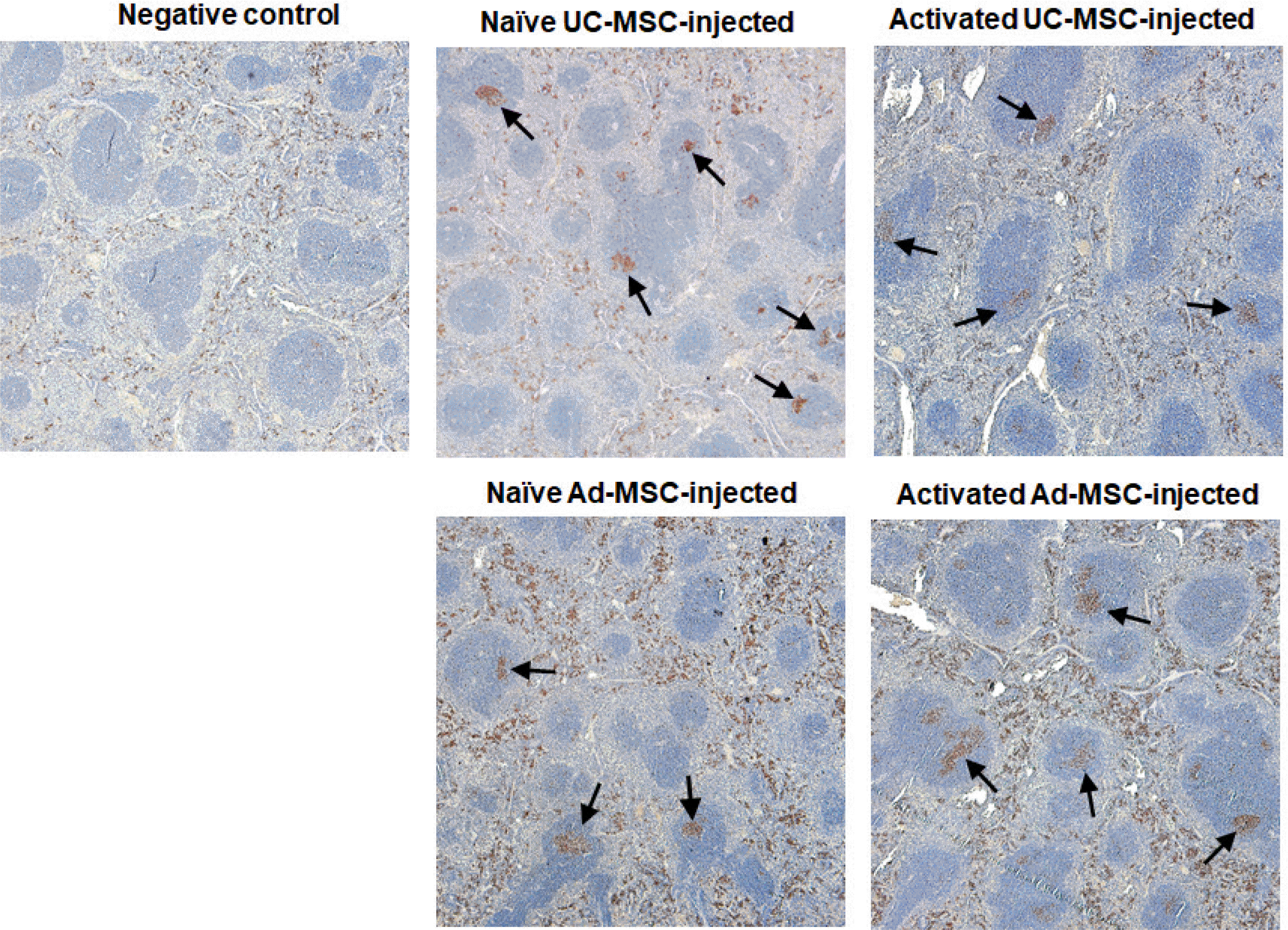
Fig. 5
Humoral immune responses against injected human MSCs in C57BL/6 mice. Mice were injected twice with naïve hUC-MSCs or hAd-MSCs on days 1 and 31, and blood samples were drawn on day 38. (A) Sera were obtained and subjected to ELISA for total Ig and each Ig isotype. (B) hUC-MSCs or hAd-MSCs were stained with the sera from mice injected with hUC-MSCs or hAd-MSCs, respectively, and subjected to flow cytometric analysis. *p<0.05 and **p<0.01 vs. the control.
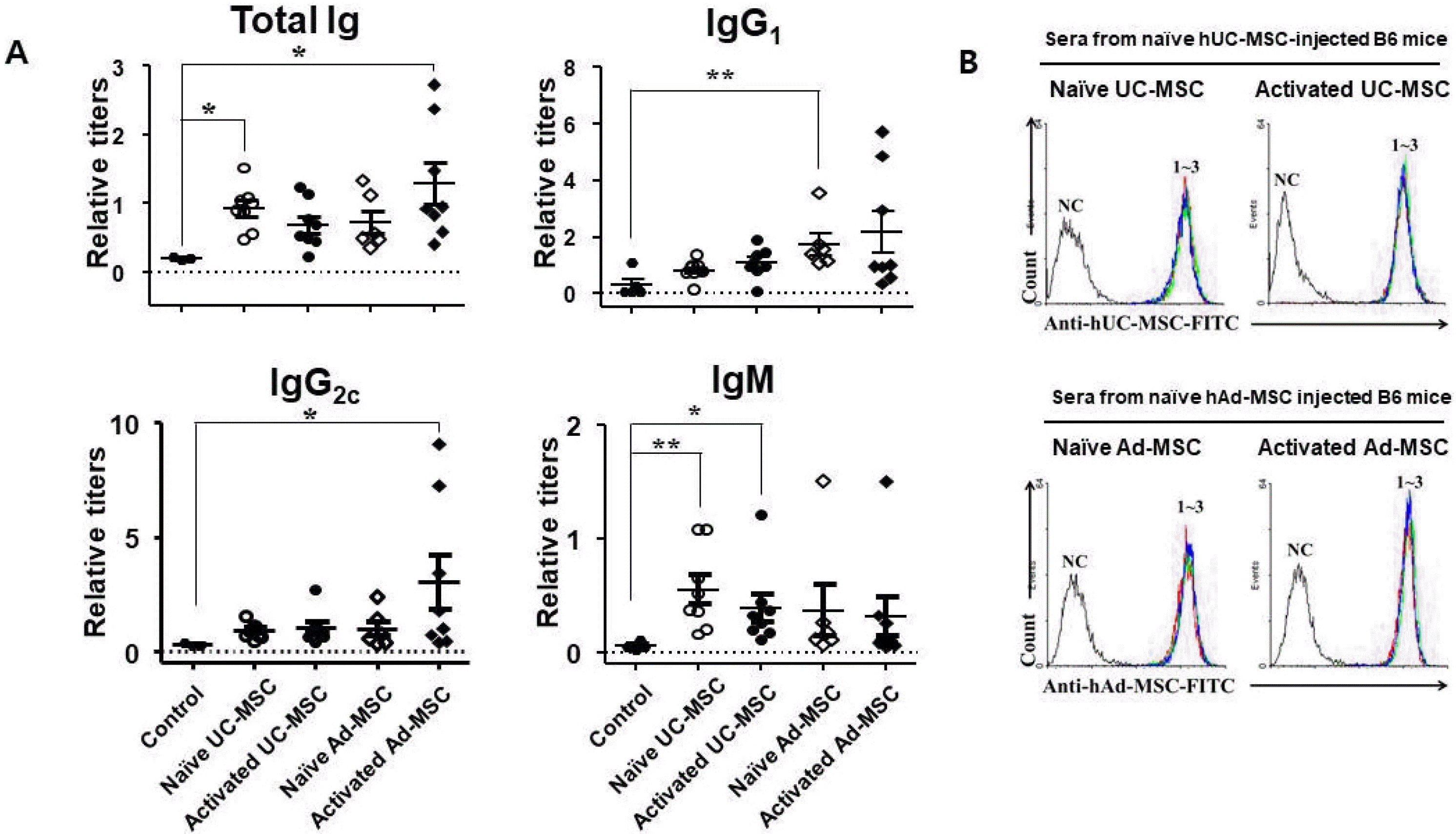
Table 1
Absolute concentrations of Ig isotypes in the secondary sera of Balb/c mice
Table 2
Median fluorescent intensity (MFI) values