Abstract
Background and Objectives
Although human-induced pluripotent stem cells (hiPSC) can be efficiently differentiated into cardiomyocytes (CMs), the heterogeneity of the hiPSC-CMs hampers their applications in research and regenerative medicine. Retinoic acid (RA)-mediated signaling pathway has been proved indispensable in cardiac development and differentiation of hiPSC toward atrial CMs. This study was aimed to test whether RA signaling pathway can be manipulated to direct the differentiation into sinoatrial node (SAN) CMs.
Methods and Results
Using the well-characterized GiWi protocol that cardiomyocytes are generated from hiPSC via temporal modulation of Wnt signaling pathway by small molecules, RA signaling pathway was manipulated during the differentiation of hiPSC-CMs on day 5 post-differentiation, a crucial time point equivalent to the transition from cardiac mesoderm to cardiac progenitor cells in cardiac development. The resultant CMs were characterized at mRNA, protein and electrophysiology levels by a combination of qPCR, immunofluorescence, flow cytometry, and whole-cell patch clamp. The results showed that activation of the RA signaling pathway biased the differentiation of atrial CMs, whereas inhibition of the signaling pathway biased the differentiation of sinoatrial node-like cells (SANLCs).
Human induced pluripotent stem cells (hiPSC), similar to embryonic stem cells (ESC) possessing pluripotency and self-renewal capacity, and importantly facing far few medical ethical challenges, have been used extensively in disease modeling, mechanistical studies, cell replacement therapy, and drug screening, among others (1-4). Through manipulating signaling pathways by small molecular drugs, hiPSC can now be very efficiently differentiated to cardiomyocytes (CMs) (5). However, these hiPSC-CMs are a heterogeneous population consisting of ventricular-, atrial- and sinoatrial-CMs and some no-differentiated hiPSC, which significantly limit their clinical applications and cannot be used to model specific cardiovascular diseases such as atrial fibrillation (AF) (6). In addition, accumulating evidence have shown that the contamination of the sinoatrial node-like cells (SANLCs) is one of the most important factors leading to the development of cardiac arrhythmia post hiPSC-CMs transplantation (7, 8). Therefore, it is of great importance to optimize the differentiation protocols to enrich specific CMs subtypes.
RA (retinoic acid) is the active form of vitamin A that through its receptor participates in both cardiac development and maturation (9). Recently, manipulation of RA signaling pathway has emerged as a critical switch in directing cardiomyocyte differentiation (10). Activation of RA signaling pathway biased the differentiation of hiPSC to atrial-like CMs, whereas inhibition of the signaling pathway enriched the ventricular-like CMs (11, 12). However, the timing and dosage of the RA signaling in the differentiation toward SANLC remain to be studied. Embryonic heart development starts from cardiac crescent derived from primitive streak, which then fuses to form primitive heart tube. As development progresses, the primitive heart tube forms anterior and posterior poles. Precursor cells on the anterior pole develop into ventricles, whereas cells on the posterior pole mainly contribute to the formation of atria and sinus venousus, and the latter happens to be where the sinoatrial node cells locate (13, 14). In adult heart, the sinus node is located at the border region between superior vena cava and right atrium. These discoveries suggest that the spatial distribution of the precursor cells of atrial and sinoatrial node CMs is correlated to their different responses to signaling pathway.
In this study, based on the hiPSC-CMs differentiation protocol adopted and optimized (15) from which RA signaling pathway was manipulated during the transition period from cardiac mesoderm to myocardial precursor cells, we found that activation of RA signaling biased the differentiation to atrial-CMs, whereas inhibition of the signaling pathway enriched SANLCs. These discoveries not only improve our understanding of the roles of RA in CMs differentiation but provide an efficient protocol to enrich SANLCs.
hiPSC (HNF-P30-P11), human embryonic stem cell (hESC) line (HN4) and BioCISO medium was obtained from OSINGLAY BIO (Guangzhou, CHINA). Human fibroblast (hF) cells (BJ) were purchased from ATCC. Anti-Ki67 (ab15580), -TBX18 (ab115262) and -TBX3 (ab154828), -SHOX2 (ab55740) were purchased from Abcam. Anti-NANOG (#3580), -OCT4 (#2750), and -TRA-1-60 (#4746) were all from Cell Signaling Techno-logy. Anti-cTNT (MS-295-P1) was from Thermo-Fisher Scientific. Anti-α-actinin (A7811) was from Sigma-Aldrich. Anti-COUPTFII (PP-H7147-00) was from R&D. Anti-NKX2.5 (MAB2444) was from NOVUS. All other chemi-cals, unless stated otherwise, were products of Sigma -Aldrich.
hiPSC was cultured in BioCISO medium that was refreshed every day, which was then either propagated or differentiated upon reaching around 80% confluence. hiPSC was characterized by proliferation marker Ki67, hiPSC-specific transcription factors, NANOG and OCT4, and iPS-specific membrane protein, TRA-1-60 using both flow cytometry and immunofluorescence (IF).
Cardiomyocyte differentiation was performed by small molecule based temporal modulation of Wnt signaling using GSK inhibitor (Gi) and Wnt inhibitor (Wi) (named as GiWi protocol) (16). Briefly, hiPSC was harvested at 80% confluence using 0.5 mM EDTA and resuspended with hiPSC-maintaining medium to 0.5×105 cells per milliliter (ml). Two ml of the cell suspension was added per well in a 12-well Matrigel-coated plate, and this is designated as day minus 4. At day 0, the cells were refreshed with RPMI/B27 medium without insulin (RPMI/B27−) containing 10 μM GSK3 inhibitor, CHIR99021 (S1263, Sigma, USA), and continued to incubate for 24 h. On day 1, the cells were refreshed with RPMI/B27− and continued to incubate for 48 h. On day 3, the cells were refreshed with RPMI/B27− containing 5 μM Wnt inhibitor, IWP2 (3533, Tocris Bioscience, UK) without insulin and continued to incubate for 48 h. On day 5, the cells were refreshed with RPMI/B27− and continued to incubate for 48 h. From day 7, the cells were refreshed with RPMI/B27 medium containing insulin (RPMI/B27+) every 3 days till day 35. The beating cardiomyocytes can be seen as early as on day 8. The differentiated cardiomyocytes were then characterized by immunofluore-scence for the expression of cTNT (MS-295-P1, Thermo Fisher Scientific, USA) and α-actinin (A7811, Sigma, USA), and by flow cytometry for cTNT expression.
At the differentiation day 5∼7, equivalent to the cardiac mesodermal stage in vivo, different concentrations of GiWi signaling pathway agonist, RA (0, 0.5, 1, 2, and 5 μM) and antagonist, BMS (0, 1, 2, 5, and 10 μM) were added to the differentiating cardiomyocytes till day 21, and the expression of atrial markers, NPPA, MYL7, COUPTFII, KCNJ5, and CX40 were analyzed by qPCR to determine the optimum concentrations of RA and BMS that regulate the maximum expression of atrial markers. The optimum concentrations of RA and BMS were then added to the differentiating cardiomyocytes on day 5∼7, and hiPSC was continued to differentiate to day 21 for differentiation efficiency evaluation and to day 60 for electrophysiological analysis by patch-clamp, respectively. qPCR was used for the expression of the Sinus node markers, SHOX2, TBX18, TBX3, HCN4, ISL1, CX30.2, CACNB1, CACNA1A, KCNN4, KCNK2, KCND2, and SCN3B; IF was used for atrial marker (COUPTFII) and the sinus node markers (TBX18 and TBX3); and flow cytometry was used to analyze atrial marker (COUPTFII) and markers for identifying sinus node cell, i.e., cTNT+/NKX2.5− and cTNT+/SHOX2+. Whole cell patch-clamp was used to record the action potential and analyze their electrophysiological characteristics of atrial-, pacemaker-, and ventricular-like cardiomyocytes.
Total RNA was isolated using TRIzol (15596026, Invitrogen, USA). One μg total RNA was reversely transcribed in a total volume of 10 μl with ReverTra Ace qPCR RT Master Mix kit (FSQ-201, TOYOBO, Japan) following the vendor’s instructions. The cDNA was diluted 3 times, and 1 μl was used for real-time PCR in a 20-μl reaction using SYBR Green Real Time PCR Mix (204143, Qiagen, Germany). The PCR conditions were 95℃ for 2 min, followed by 40 cycles of 95℃ for 20" and 60℃ for 15". All primers are listed in Supplementary Table S1. The expression of target gene was normalized to that of GAPDH and calculated using the 2−ΔΔCt method.
Single hiPSC cell suspension and induced cardiomyo-cytes were seeded in a μ-Slide 8 well (80827, ibidi) pre-coated with Matrigel at the density of 2×104 cells per well for 48 h. Cells were fixed with 4% (w/v) paraformaldehyde (PFA) for 15 min at room temperature (RT), permeabilized, blocked in 5% (w/v) BSA in PBS for 30 min, and then incubated with primary antibodies as instructed by vendors at 4℃ for overnight. Then, the plates were washed with PBS on a decolorizing shaker for 3 times at 5 minutes each, followed by incubation with species-specific fluorescence-conjugated secondary antibodies at RT for 1 h. The secondary antibodies were: Alexa fluor 488 labeled goat anti-rabbit IgG (A11008, Invitrogen, USA), alexa fluor 594 labeled goat anti-rabbit IgG (R37177, Invitrogen, USA), and alexa fluor 594 labeled goat anti-mouse IgG (A-11005, Invitrogen, USA). The cells were washed 3 times with PBS and then counterstained using 0.5 μg/ml of DAPI (4083, Cell Signaling Technology, USA) for 15 min at RT. The chambers were mounted and visualized under fluorescence microscopy (IX83, Olympus, Japan). The corresponding antibody isotype controls were: mouse IgG (ab205719, abcam, USA), mouse IgG1 (#5415, Cell Signaling Technology, USA), rabbit IgG (ab205718, abcam, USA), and rabbit IgM (ab37424, abcam, USA).
The induced cardiomyocytes were digested with 0.25% trypsin/0.5 mM EDTA into single cell suspension, spun down and washed with PBS. Cells were fixed with 4% formaldehyde for 10 min at RT and chilled on ice for 1 min. Permeabilization was performed by adding one tenth of ice-cold 100% methanol slowly to the pre-chilled cells and cells were continued to incubate on ice for 30 min. Cells were then blocked with blocking buffer (0.5% BSA in PBS) for 10 min; incubated with the primary antibody as indicated in the corresponding figures. for 1 h at RT, washed with PBS, and incubated with the corresponding species-specific fluorescence-conjugated secondary antibodies for 30 min at room temperature. The secondary antibodies used were, Alexa fluor 488 labeled goat anti-mouse IgG (A-11029, Invitrogen, USA), and Alexa fluor 647 labeled goat anti-rabbit IgG (A-32733, Invitrogen, USA). Cells were analyzed using a flow cytometer (651155, BD FACS Verse, BD Bioscience, USA) according to the manufacturer’s protocol. The corresponding antibody isotype controls were: mouse IgG (ab205719, abcam, USA), mouse IgG1 (#5415, Cell Signaling Technology, USA), mouse IgG2a (ab18415, abcam, USA), and rabbit IgG (ab205718, abcam, USA).
AP recording was performed following El-Battrawy et al.’s protocol with some modifications (17). Briefly, on day 60 after differentiation, induced cardiomyocytes were dissociated into single cell suspension by incubation with type I collagenase (2 mg/ml) for 30 min, followed by 0.25% trypsin without EDTA for 3 min. 1×104 cells were seeded into a 3.5-cm dish containing a lysine-treated glass coverslip and incubated for 3 days. AP was recorded using the whole cell patch-clamp method. Briefly, adherent cells on the coverslip were placed in the recording chamber and perfused with bath solution containing 140 mM NaCl, 1 mM MgCl2, 5 mM KCl, 1.8 mM CaCl2, 5 mM 4-(2-hydro-xyethyl)-1-piperazineethanesulfonic acid (HEPES), and 10 mM glucose (the pH was adjusted to 7.4, and the osmolality to 301±3 mOsm, respectively). The patch pipettes were pulled from borosilicate glass capillaries (7-000-0650-LHC, Drum-mond, USA) by a horizontal puller (PC100, NARI SHIGE, Japan) and had resistances of 1.5∼3 MΩ. Pipette solution consists of 110 mM K-gluconate, 20 mM KCl, 1 mM CaCl2, 1 mM MgCl2, 10 mM HEPES, 5 mM ethylene glycol tetra-acetic acid potassium chloride (EGTA-KOH), 5 mM ATP-Mg2+, and 5 mM Na-phosphocreatine. The pH was adjusted to 7.2 by KOH, and the osmolality to 290±3 mOsm. A Multiclamp 700B amplifier was used to record APs, and data were analyzed using a custom software.
To validate the authenticity of the hiPSC, the expression of the signature molecules of hiPSC (OCT4, NANOG, SOX2 and TRA-1-60) and proliferation marker (Ki67) were evaluated by both IF (A∼D), qPCR (E∼H) and flow cytometry (I∼L). As shown in Fig. 1, the hiPSC highly expressed pluripotent stem cell-specific markers OCT4 (A, E, I), NANOG (B, F, J), SOX2 (G) and TRA-1-60 (C, K), and proliferation marker Ki67 (D, H, L). These results demonstrate that the hiPSC possesses their pluripotency and self-renewal capability.
Cardiomyocytes were induced following the GiWi protocol with some modifications (18). On day 35 after the differentiation, the induced cardiomyocytes displayed relatively mature discernable striae and sacromeric structure and were positive for cardiomyocyte-specific markers, α-actinin by IF (Fig. 2A) and cardiac sarcomere proteins, cTNT by both IF (Fig. 2B) and flow cytometry showing that 83.5% of the induced cardiomyocytes were positive for cTNT (Fig. 2C). These results demonstrated the high efficiency of our modified GiWi differentiation protocol.
The differentiation of hiPSC to CMs is a spatiotemporally coordinated process. Our previous study showed that day 5 of the GiWi differentiation process was a critical time point for the transition from cardiac mesoderm to cardiac precursor cells (18). RA signaling pathway agonist, RA was introduced into the differentiation on day 5, and atrial cardiomyocyte markers was analyzed on day 21 by qPCR. The results showed that RA at 2 μM significantly induced the expression of natriuretic peptide A (NPPA), myosin light chain 7 (MYL7), COUP transcription factor 2 (COUPTFII), potassium inwardly rectifying channel subfamily J member 5 (KCNJ5), and connexin 40 (CX40) (Fig. 3A). Therefore, 2 μM RA was used to further study the effect of RA on the differentiation of hiPSC to the Sinus node-like cells. When gene expression was analyzed on day 21 (Fig. 3B), RA significantly decreased the expression of sinus node cells-specific transcription factors, SHOX2, TBX18, TBX3 and ISL1, ion channels, HCN4, SCN3B, CACNA1A and KCNN4, and gap junction CX30.2, but no significant effect was observed on the expression of CACNB1, KCNK2 and KCND2.
To further investigate the effect of RA signaling pathway on the differentiation of CM subtypes, a RA signaling pathway inhibitor, BMS was introduced on day 5 of the GiWi protocol. When gene expression of atrial cells markers was analyzed on day 21 after the differentiation, BMS dose-dependently inhibited the expression of NPPA, COUPTFII and KCNJ5, and affected the expression of MYL7 and CX40 in a biphasic manner, with 5 and 2 μM inhibited the most on MYL7 and CX40 respectively (Fig. 4A). Therefore, 5 μM was used as the optimum concentration of BMS in the following study. When gene expression was analyzed on day 21, differentiated cardio-myocytes treated with BMS expressed higher levels of sinoatrial node-specific transcription factors, SHOX2, TBX18, TBX3 and ISL1, ion channels, HCN4, CACNB1, CACNA1A, KCNN4, KCNK2, and KCND2, and gap junction CX30.2 (Fig. 4B).
To validate the above findings that RA and BMS promoted the differentiation of atrial and Sinoatrial-like cells respectively, the resultant cardiomyocytes on day 21 were analyzed by IF and flow cytometry, and whole cell patch-clamp was performed on day 60. Compared to RA, BMS decreased the percentage of atrial-specific transcription factor, COUPTFII-positive cells (Fig. 5A, B), whereas increased the percentage of SANLCs-specific transcription factors, TBX18− (Fig. 5C and 5D) and TBX3-po-sitive cells (Fig. 5E and 5F). These results were further confirmed by flow cytometry showing that BMS decreased the percentage of RA-induced COUPTFII positive cells (BMS vs. RA, 10.4±4.2% vs. 46.9±7.6%) (Fig. 6A), and simultaneously increased the percentage of cells expressing SANLC-specific markers, cTNT+/SHOX2+ (BMS vs. RA, 30.9±6.5% vs. 11.4±3.2%) (Fig. 6B), and cTNT+/NKX2.5− MS vs. RA, 38.9±9.8% vs. 14.4±5.8%) (Fig. 6C).
To further characterize the differentiated atrial-like cells and SANLCs, the beating frequency was recorded and action potential (AP) was analyzed by whole cell patch-clamp technique. Compared to RA, BMS significantly increased the beating frequency (times/min) of differentiated cardiomyocytes (RA vs. BMS, 71.57±7.68 vs. 110.43±12.20) (Fig. 7A), and sensitized cardiomyocytes to pacing-related ion channel inhibitor, HCNi (Fig. 7B). AP analysis further showed that the ratio of cardiomyocytes displaying atrial-like AP was significantly higher in RA (10/15) than BMS (5/15) groups, whereas the ratio of cardiomyocytes displaying pacemaker-like AP was significantly higher in BMS (4/15) than RA (2/15) groups (Fig. 7C and 7D).
Mounting evidence has shown that RA is one of the most critical signaling molecules orchestrating embryonic development and regulating many crucial steps of the heart morphogenesis, such as differentiation of atria- and ventricular-CMs, and CMs maturation (9, 11). However, how the molecular mechanisms governing the differentiation of sinoatrial-like CMs remains largely unknown, which significantly limits the enrichment of the sinoatrial-like CMs for basic research and regenerative medicine. In this report, the GiWi protocol was optimized to efficiently differentiate hiPSC to pan-CMs, from which the RA signaling pathway was inhibited on day 5 post-differentiation, equivalent to the transition from cardiac mesoderm to the CM precursor cells in vivo (18), and the resultant CMs were analyzed at different time points. We found that after RA signaling pathway inhibition, the expression of atrial-CMs markers at both mRNA and protein levels were significantly suppressed, while that of sinoatrial node-like cells markers were significantly activated on day 21 post-differentiation. When CMs were analyzed on day 60 post-differentiation, the percentage of CMs showing action potential (AP) characteristic of sinoatrial-like CMs were significantly increased, whereas that of atrial CMs were significantly decreased.
Cardiac subtype specification is a precisely and spatiotemporally regulated process and atrial cardiomyocytes could be derived from cardiac mesoderm population via RA activation (11, 15). Consistent with previous reports, we observed that activation of RA during the period from cardiac mesoderm to cardiac progenitor significantly enriched the ratio of atrial-like cells from hiPSC using our previously optimized GiWi protocol (18). In addition to the enrichment effect of atrial CM subtype from human pluripotent stem cells, RA has also been reported to exert inhibitory effect on the specification of the ventricular subtype (11). However, whose effect on the generation sinoatrial node-like cells remains unclear. It was observed in our current study that activating the RA signaling pathway with RA inhibited the expression of sinoatrial CM-specific markers and then the yield of sinoatrial cells. this was further supported by inactivating RA pathway using BMS. As far as our knowledge, it is the first finding that in addition to the pro-atrial and anti-ventricular effect, RA activation also has inhibitory effect on the sinoatrial node direction although the related mechanism remains to be elucidated.
It was evidenced by Lee, et al’s study (11) that human ventricular and atrial cardiomyocytes develop from distinct mesoderm populations that are specified with different concentrations of BMP4 and Activin A and can be identified based on the expression of CD235a (Glycophorin A) and RALDH2, respectively. the RALDH2+, but not the CD235a+, mesoderm population responds to retinol to generate atrial cardiomyocytes. In consistence, our study showed that the cardiac mesoderm cells responding to the RA activator could differentiated into atrial subtype CMs in high-efficiency and vice versa. Moreover, it was also found that the activation and inhibition of RA pathway could also antagonize and promote the generation of sinoatrial node-like cells, respectively. It is therefore speculated that in addition to the ventricular and atrial ancestor sub-population, there also exists the sub-population of sinoatrial node ancestor during the cardiac mesoderm stage whose conversion into sinoatrial node cells is at least depend on the RA modulation. It will be an interesting study in our future work to verify this possible sinoatrial node mesoderm subpopulation and elucidate the involved signaling pathways modulating its differentiation of hiPSC into sinoatrial node CMs.
Currently, there are mainly two strategies to generate sinoatrial CMs. One is directing hiPSC into sinoatrial CMs through genetic engineering of all transcription factors necessary for the development of sinoatrial CMs. This strategy is technically challenging, costly, and potentially tumorigenic, which has limited its application (19-21). The other strategy is manipulating the signaling pathways necessary for the specification of CM subtype through chemical reagents. This strategy circumvents the drawbacks of the former and has attracted much attention lately. For example, at the cardiac mesoderm stage, Protze et al. (22) showed that low concentration of bone morphogenetic protein (BMP) could promote the differentiation toward sinoatrial CMs, Yechikov et al. (23) demonstrated that inhibition of nodal signaling pathway by SB431542 down-regulated the expression of the paired-like homeodomain transcription factor 2C (PITX2c), which promoted the differentiation of pacemaker-like CMs, and we showed previously that coordinated manipulation of BMP, RA and fibroblast growth factor (FGF) signaling pathways at the cardiac mesoderm stage enriched the differentiation of sinoatrial CMs (18). Although manipulation of different signaling pathways achieved high differentiation efficiency of sinoatrial CMs, the permutation of small chemicals required and the complexity of performing the differentiation in individual protocol remain to be optimized.
In summary, we developed a de novo differentiation protocol for enrichment of CM subtypes from hiPSC by manipulating the RA signaling pathway during cardiac mesoderm stage of hiPSC differentiation in the biphasic manner, in which activation of RA signaling enabled biased differentiation of atria-like cells as reported before. Moreover, RA pathway inhibition directed the enrichment of sinoatrial node-like cells generation (Fig. 8). It further broadened the understanding of the effect of RA signaling pathway on the embryonic heart development and CM subtypes specification from human pluripotent stem cells.
Supplementary data including one table can be found with this article online at https://doi.org/10.15283/ijsc21148.
Acknowledgments
This work was supported by grants from the collaborative innovation center for prevention and treatment of cardiovascular disease of Sichuan province [HD20041].
References
1. Takahashi K, Yamanaka S. 2006; Induction of pluripotent stem cells from mouse embryonic and adult fibroblast cultures by defined factors. Cell. 126:663–676. DOI: 10.1016/j.cell.2006.07.024. PMID: 16904174. PMID: https://www.scopus.com/inward/record.uri?partnerID=HzOxMe3b&scp=33747195353&origin=inward.
2. Sung TC, Liu CH, Huang WL, Lee YC, Kumar SS, Chang Y, Ling QD, Hsu ST, Higuchi A. 2019; Efficient differentiation of human ES and iPS cells into cardiomyocytes on biomaterials under xeno-free conditions. Biomater Sci. 7:5467–5481. DOI: 10.1039/C9BM00817A. PMID: 31656967. PMID: https://www.scopus.com/inward/record.uri?partnerID=HzOxMe3b&scp=85075271004&origin=inward.
3. Kishino Y, Fujita J, Tohyama S, Okada M, Tanosaki S, Someya S, Fukuda K. 2020; Toward the realization of cardiac regenerative medicine using pluripotent stem cells. Inflamm Regen. 40:1. DOI: 10.1186/s41232-019-0110-4. PMID: 31938077. PMCID: PMC6956487. PMID: 3d846dad7b4c4d6d8f29f58d98f60373. PMID: https://www.scopus.com/inward/record.uri?partnerID=HzOxMe3b&scp=85083666553&origin=inward.
4. Hartman ME, Dai DF, Laflamme MA. 2016; Human pluripotent stem cells: prospects and challenges as a source of cardiomyocytes for in vitro modeling and cell-based cardiac repair. Adv Drug Deliv Rev. 96:3–17. DOI: 10.1016/j.addr.2015.05.004. PMID: 25980938. PMCID: PMC4644514. PMID: https://www.scopus.com/inward/record.uri?partnerID=HzOxMe3b&scp=84951860090&origin=inward.
5. Burridge PW, Zambidis ET. 2013; Highly efficient directed differentiation of human induced pluripotent stem cells into cardiomyocytes. Methods Mol Biol. 997:149–161. DOI: 10.1007/978-1-62703-348-0_12. PMID: 23546754. PMID: https://www.scopus.com/inward/record.uri?partnerID=HzOxMe3b&scp=84877078624&origin=inward.
6. He JQ, Ma Y, Lee Y, Thomson JA, Kamp TJ. 2003; Human embryonic stem cells develop into multiple types of cardiac myocytes: action potential characterization. Circ Res. 93:32–39. DOI: 10.1161/01.RES.0000080317.92718.99. PMID: 12791707. PMID: https://www.scopus.com/inward/record.uri?partnerID=HzOxMe3b&scp=0038300672&origin=inward.
7. Park M, Yoon YS. 2018; Cardiac regeneration with human pluripotent stem cell-derived cardiomyocytes. Korean Circ J. 48:974–988. DOI: 10.4070/kcj.2018.0312. PMID: 30334384. PMCID: PMC6196153. PMID: https://www.scopus.com/inward/record.uri?partnerID=HzOxMe3b&scp=85056373666&origin=inward.
8. Sugiura T, Hibino N, Breuer CK, Shinoka T. 2016; Tissue-engineered cardiac patch seeded with human induced pluripotent stem cell derived cardiomyocytes promoted the regeneration of host cardiomyocytes in a rat model. J Cardio-thorac Surg. 11:163. DOI: 10.1186/s13019-016-0559-z. PMID: 27906085. PMCID: PMC5131419. PMID: https://www.scopus.com/inward/record.uri?partnerID=HzOxMe3b&scp=84999863221&origin=inward.
9. Miao S, Zhao D, Wang X, Ni X, Fang X, Yu M, Ye L, Yang J, Wu H, Han X, Qu L, Li L, Lan F, Shen Z, Lei W, Zhao ZA, Hu S. 2020; Retinoic acid promotes metabolic maturation of human embryonic stem cell-derived cardio-myocytes. Theranostics. 10:9686–9701. DOI: 10.7150/thno.44146. PMID: 32863954. PMCID: PMC7449904. PMID: https://www.scopus.com/inward/record.uri?partnerID=HzOxMe3b&scp=85090108048&origin=inward.
10. Devalla HD, Schwach V, Ford JW, Milnes JT, El-Haou S, Jackson C, Gkatzis K, Elliott DA, Chuva de Sousa Lopes SM, Mummery CL, Verkerk AO, Passier R. 2015; Atrial-like cardiomyocytes from human pluripotent stem cells are a robust preclinical model for assessing atrial-selective pharm-acology. EMBO Mol Med. 7:394–410. DOI: 10.15252/emmm.201404757. PMID: 25700171. PMCID: PMC4403042. PMID: https://www.scopus.com/inward/record.uri?partnerID=HzOxMe3b&scp=84926165142&origin=inward.
11. Lee JH, Protze SI, Laksman Z, Backx PH, Keller GM. 2017; Human pluripotent stem cell-derived atrial and ventricular cardiomyocytes develop from distinct mesoderm popula-tions. Cell Stem Cell. 21:179–194.e4. DOI: 10.1016/j.stem.2017.07.003. PMID: 28777944. PMID: https://www.scopus.com/inward/record.uri?partnerID=HzOxMe3b&scp=85026505552&origin=inward.
12. Yu J, Vodyanik MA, Smuga-Otto K, Antosiewicz-Bourget J, Frane JL, Tian S, Nie J, Jonsdottir GA, Ruotti V, Stewart R, Slukvin II, Thomson JA. 2007; Induced pluripotent stem cell lines derived from human somatic cells. Science. 318:1917–1920. DOI: 10.1126/science.1151526. PMID: 18029452. PMID: https://www.scopus.com/inward/record.uri?partnerID=HzOxMe3b&scp=36749043230&origin=inward.
13. Burridge PW, Matsa E, Shukla P, Lin ZC, Churko JM, Ebert AD, Lan F, Diecke S, Huber B, Mordwinkin NM, Plews JR, Abilez OJ, Cui B, Gold JD, Wu JC. 2014; Chemically defined generation of human cardiomyocytes. Nat Methods. 11:855–860. DOI: 10.1038/nmeth.2999. PMID: 24930130. PMCID: PMC4169698. PMID: https://www.scopus.com/inward/record.uri?partnerID=HzOxMe3b&scp=84905242471&origin=inward.
14. Paige SL, Plonowska K, Xu A, Wu SM. 2015; Molecular regulation of cardiomyocyte differentiation. Circ Res. 116:341–353. DOI: 10.1161/CIRCRESAHA.116.302752. PMID: 25593278. PMCID: PMC4299877. PMID: https://www.scopus.com/inward/record.uri?partnerID=HzOxMe3b&scp=84921954526&origin=inward.
15. Zhang Q, Jiang J, Han P, Yuan Q, Zhang J, Zhang X, Xu Y, Cao H, Meng Q, Chen L, Tian T, Wang X, Li P, Hescheler J, Ji G, Ma Y. 2011; Direct differentiation of atrial and ventricular myocytes from human embryonic stem cells by alternating retinoid signals. Cell Res. 21:579–587. DOI: 10.1038/cr.2010.163. PMID: 21102549. PMCID: PMC3203651. PMID: https://www.scopus.com/inward/record.uri?partnerID=HzOxMe3b&scp=79954418253&origin=inward.
16. Lian X, Zhang J, Azarin SM, Zhu K, Hazeltine LB, Bao X, Hsiao C, Kamp TJ, Palecek SP. 2013; Directed cardiomyocyte differentiation from human pluripotent stem cells by modulating Wnt/β-catenin signaling under fully defined conditions. Nat Protoc. 8:162–175. DOI: 10.1038/nprot.2012.150. PMID: 23257984. PMCID: PMC3612968. PMID: https://www.scopus.com/inward/record.uri?partnerID=HzOxMe3b&scp=84872016692&origin=inward.
17. El-Battrawy I, Zhao Z, Lan H, Cyganek L, Tombers C, Li X, Buljubasic F, Lang S, Tiburcy M, Zimmermann WH, Utikal J, Wieland T, Borggrefe M, Zhou XB, Akin I. 2018; Electrical dysfunctions in human-induced pluripotent stem cell-derived cardiomyocytes from a patient with an arrhythmogenic right ventricular cardiomyopathy. Europace. 20:f46–f56. DOI: 10.1093/europace/euy042. PMID: 29566126. PMID: https://www.scopus.com/inward/record.uri?partnerID=HzOxMe3b&scp=85048631276&origin=inward.
18. Liu F, Fang Y, Hou X, Yan Y, Xiao H, Zuo D, Wen J, Wang L, Zhou Z, Dang X, Zhou R, Liao B. 2020; Enrichment differentiation of human induced pluripotent stem cells into sinoatrial node-like cells by combined modulation of BMP, FGF, and RA signaling pathways. Stem Cell Res Ther. 11:284. DOI: 10.1186/s13287-020-01794-5. PMID: 32678003. PMCID: PMC7364513. PMID: 78f108ffbfa84397ae25d6418e0cb953. PMID: https://www.scopus.com/inward/record.uri?partnerID=HzOxMe3b&scp=85088212795&origin=inward.
19. Kapoor N, Liang W, Marbán E, Cho HC. 2013; Direct conversion of quiescent cardiomyocytes to pacemaker cells by expression of Tbx18. Nat Biotechnol. 31:54–62. DOI: 10.1038/nbt.2465. PMID: 23242162. PMCID: PMC3775583. PMID: https://www.scopus.com/inward/record.uri?partnerID=HzOxMe3b&scp=84872192083&origin=inward.
20. Gorabi AM, Hajighasemi S, Tafti HA, Atashi A, Soleimani M, Aghdami N, Saeid AK, Khori V, Panahi Y, Sahebkar A. 2019; TBX18 transcription factor overexpression in human-induced pluripotent stem cells increases their differentiation into pacemaker-like cells. J Cell Physiol. 234:1534–1546. DOI: 10.1002/jcp.27018. PMID: 30078203. PMID: https://www.scopus.com/inward/record.uri?partnerID=HzOxMe3b&scp=85052653407&origin=inward.
21. Zhao H, Wang F, Zhang W, Yang M, Tang Y, Wang X, Zhao Q, Huang C. 2020; Overexpression of TBX3 in human induced pluripotent stem cells (hiPSCs) increases their differentiation into cardiac pacemaker-like cells. Biomed Ph-armacother. 130:110612. DOI: 10.1016/j.biopha.2020.110612. PMID: 32771895. PMID: https://www.scopus.com/inward/record.uri?partnerID=HzOxMe3b&scp=85089030426&origin=inward.
22. Protze SI, Liu J, Nussinovitch U, Ohana L, Backx PH, Gepstein L, Keller GM. 2017; Sinoatrial node cardiomyocytes derived from human pluripotent cells function as a biological pacemaker. Nat Biotechnol. 35:56–68. DOI: 10.1038/nbt.3745. PMID: 27941801. PMID: https://www.scopus.com/inward/record.uri?partnerID=HzOxMe3b&scp=85011032595&origin=inward.
23. Yechikov S, Kao HKJ, Chang CW, Pretto D, Zhang XD, Sun YH, Smithers R, Sirish P, Nolta JA, Chan JW, Chiamvimonvat N, Lieu DK. 2020; NODAL inhibition promotes differentiation of pacemaker-like cardiomyocytes from human induced pluripotent stem cells. Stem Cell Res. 49:102043. DOI: 10.1016/j.scr.2020.102043. PMID: 33128951. PMCID: PMC7814970. PMID: https://www.scopus.com/inward/record.uri?partnerID=HzOxMe3b&scp=85093081098&origin=inward.
Fig. 1
Characterization of the hiPSC. hiPSC expresses high levels of OCT4 (A, E, I), NANOG (B, F, J), TRA-1-60 (C, K), and Ki67 (D, H, L) by IF, qPCR and flow cytometry respectively. In addition, pluripotent marker SOX2 was also highly expressed in hiPSC using the qPCR data (G). For qPCR analysis (E∼G), the terminal differentiated human fibroblast (hF) cell line (BJ) served as negative control and human embryonic stem cell (hESC) line (HN4) as positive control. The expression was normalized to that of GAPDH. Data are presented as ‘Mean±SD’ from at least 3 independent experiments with duplicate on each sample, with ns denoting non-significant and nd, not detectable. Scale bars=100 μm (400× magnification).
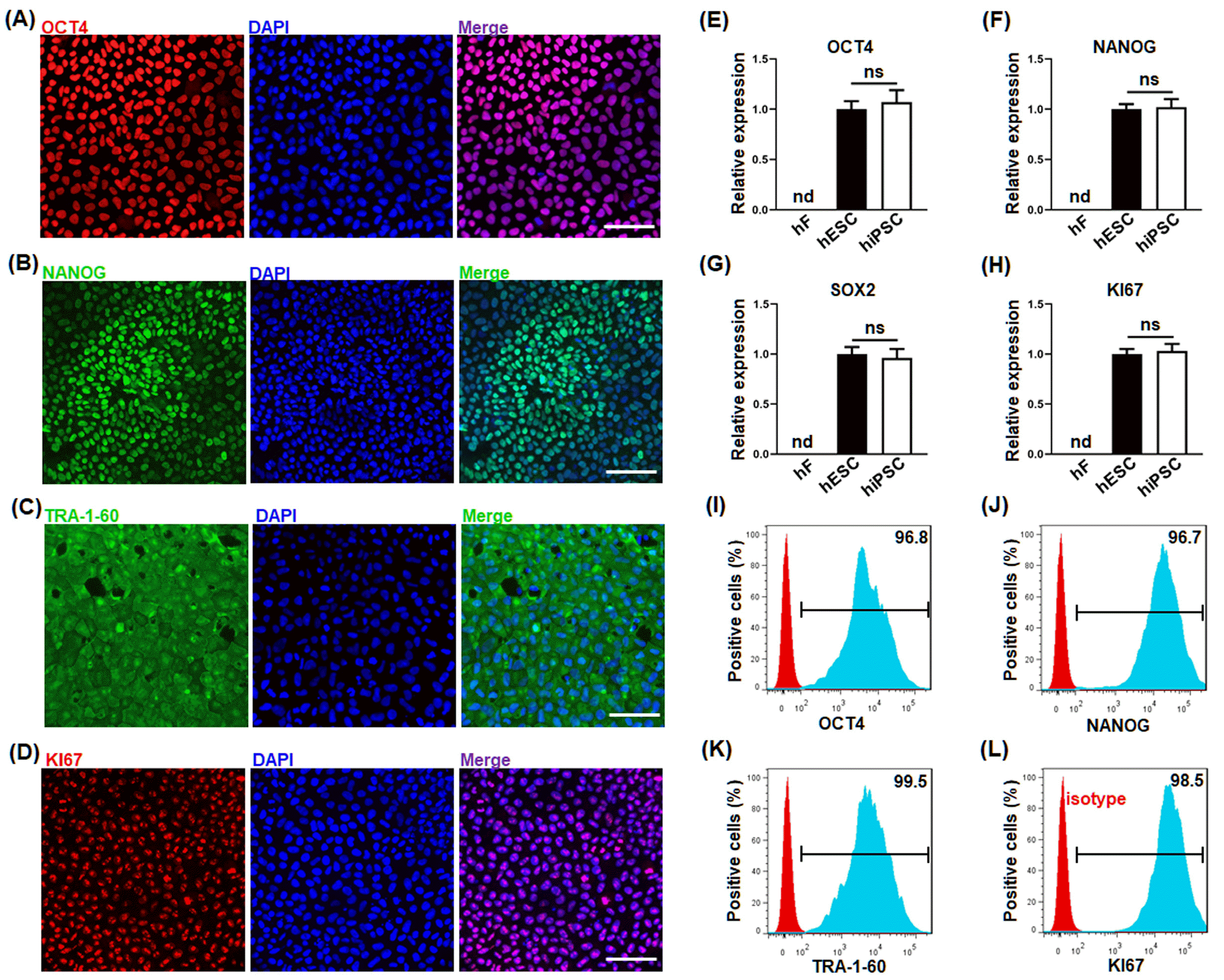
Fig. 2
Characterization of the cardiomyocytes derived from hiPSC. Representative images of immunofluorescence and FACS plots showing that cardiomyocytes derived from hiPSC on day 35 after differentiation express α-actinin by IF (A) and cTNT by IF (B) and flow cytometry (C). Scale bars=100 μm (400× magnification).
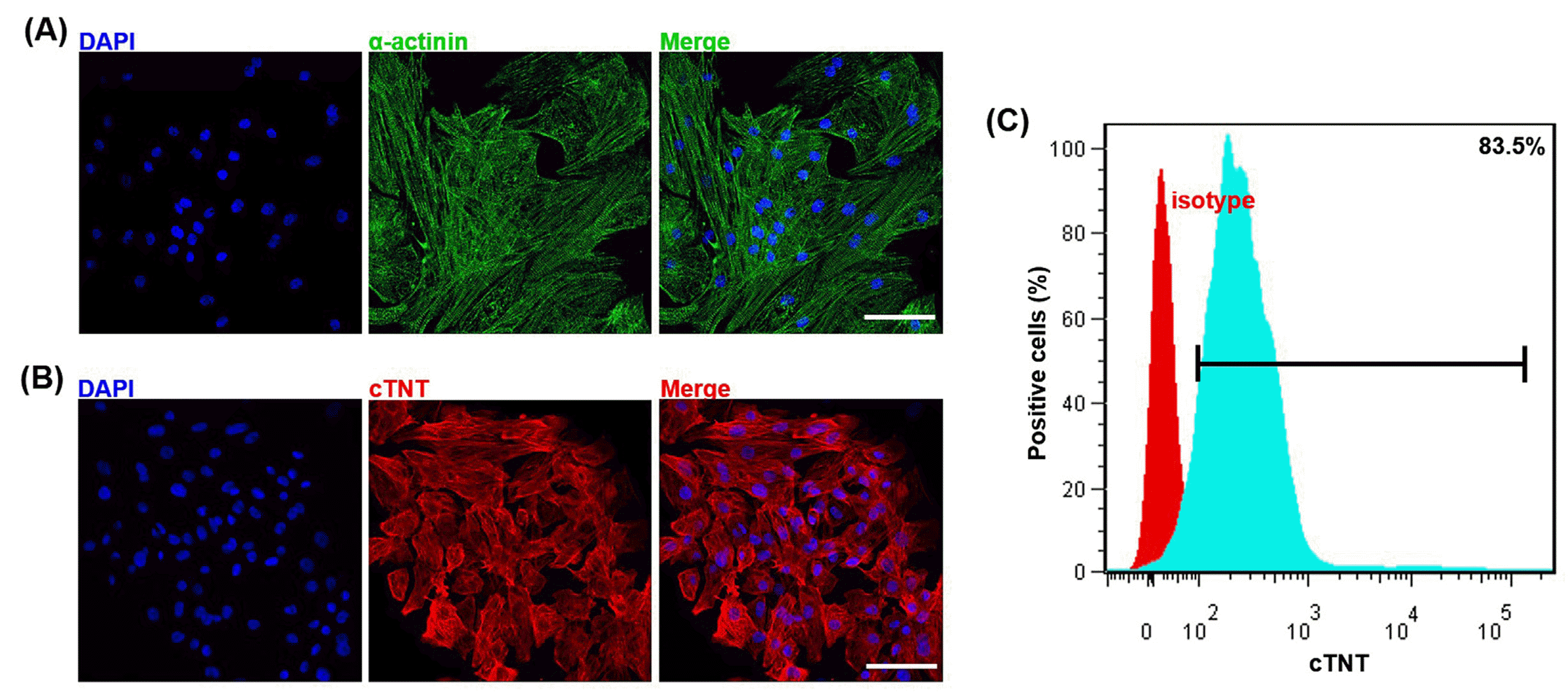
Fig. 3
Activation of RA signaling pathway by RA biases the differentiation of hiPSC toward atrial cardiomyocytes. (A) RA was introduced on day 5 at the concentrations indicated, the effect on the expression of NPPA, MYL7, COUPTFII, KCNJ5, and CX40 was analyzed by qPCR. (B) 2 μM RA was introduced on day 5 after the differentiation, and the expression of SHOX2, TBX18, TBX3, HCN4, ISL1, CX30.2, CACNB1, CACNA1A, KCNN4, KCNK2, KCND2, and SCN3B was quantitated by qPCR on day 21. The expression was normalized to that of GAPDH. Data are presented as ‘Mean±SD’ from at least 3 indepen-dent experiments with duplicate on each sample, with ns denoting non -significant, *denoting p<0.05, and **denoting p<0.01.
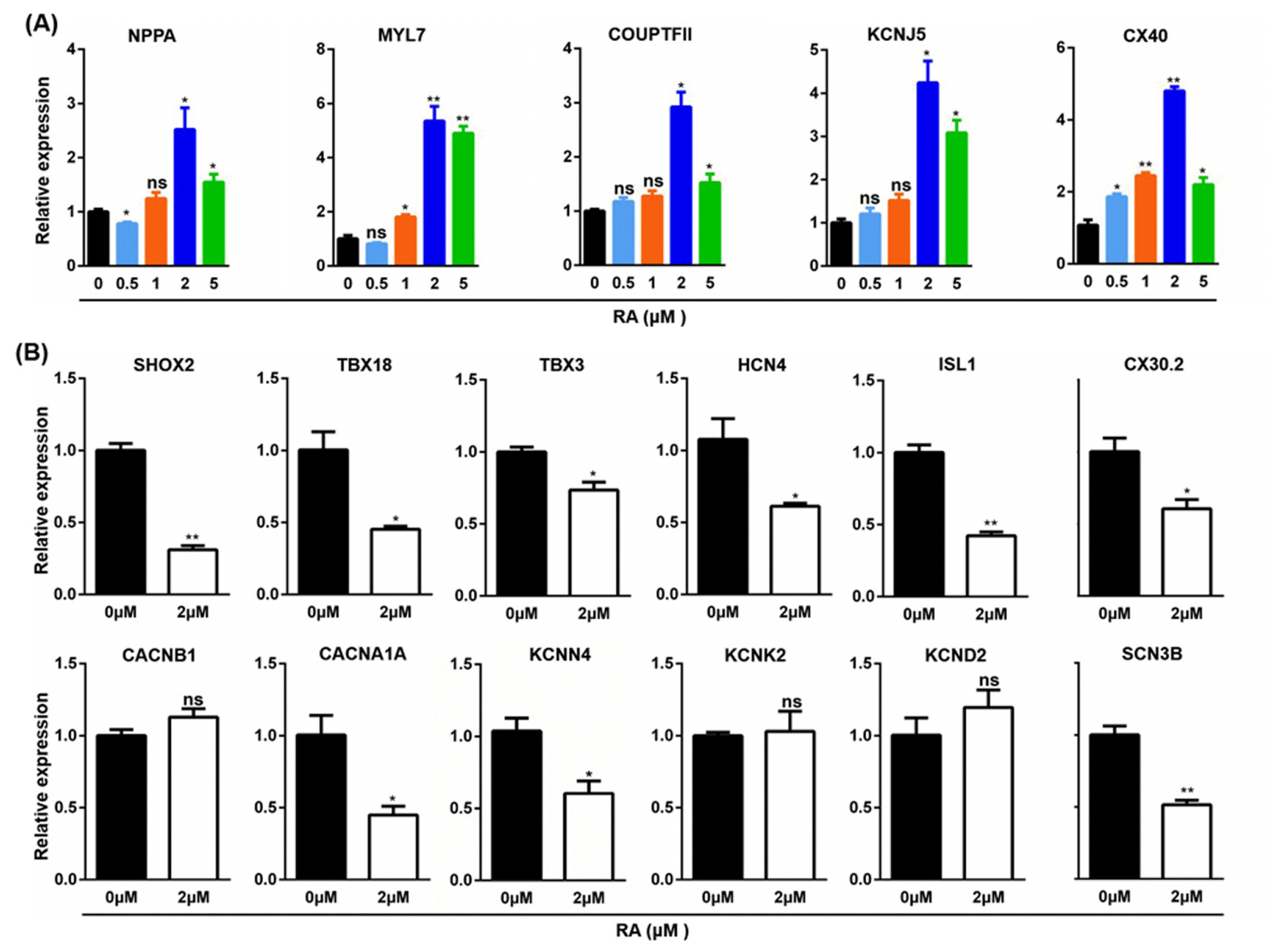
Fig. 4
Inhibition of RA signaling pathway by BMS biases the differentiation of hiPSC toward Sinoatrial node-like cells. (A) BMS was introduced on day 5 at the concentrations indicated, the effect on the expression of NPPA, MYL7, COUPTFII, KCNJ5, and CX40 was analyzed by qPCR. (B) 5 μM BMS was introduced on day 5 after the differentiation, and the expression of SHOX2, TBX18, TBX3, HCN4, ISL1, CX30.2, CACNB1, CACNA1A, KCNN4, KCNK2, KCND2, and SCN3B was quantitated by qPCR on day 21. The expression was normalized to that of GAPDH. Data are presented as ‘Mean±SD’ from at least 3 independent experiments with duplicate on each sample, with ns denoting non-significant, *denoting p<0.05, **denoting p<0.01, and ***denoting p<0.001.
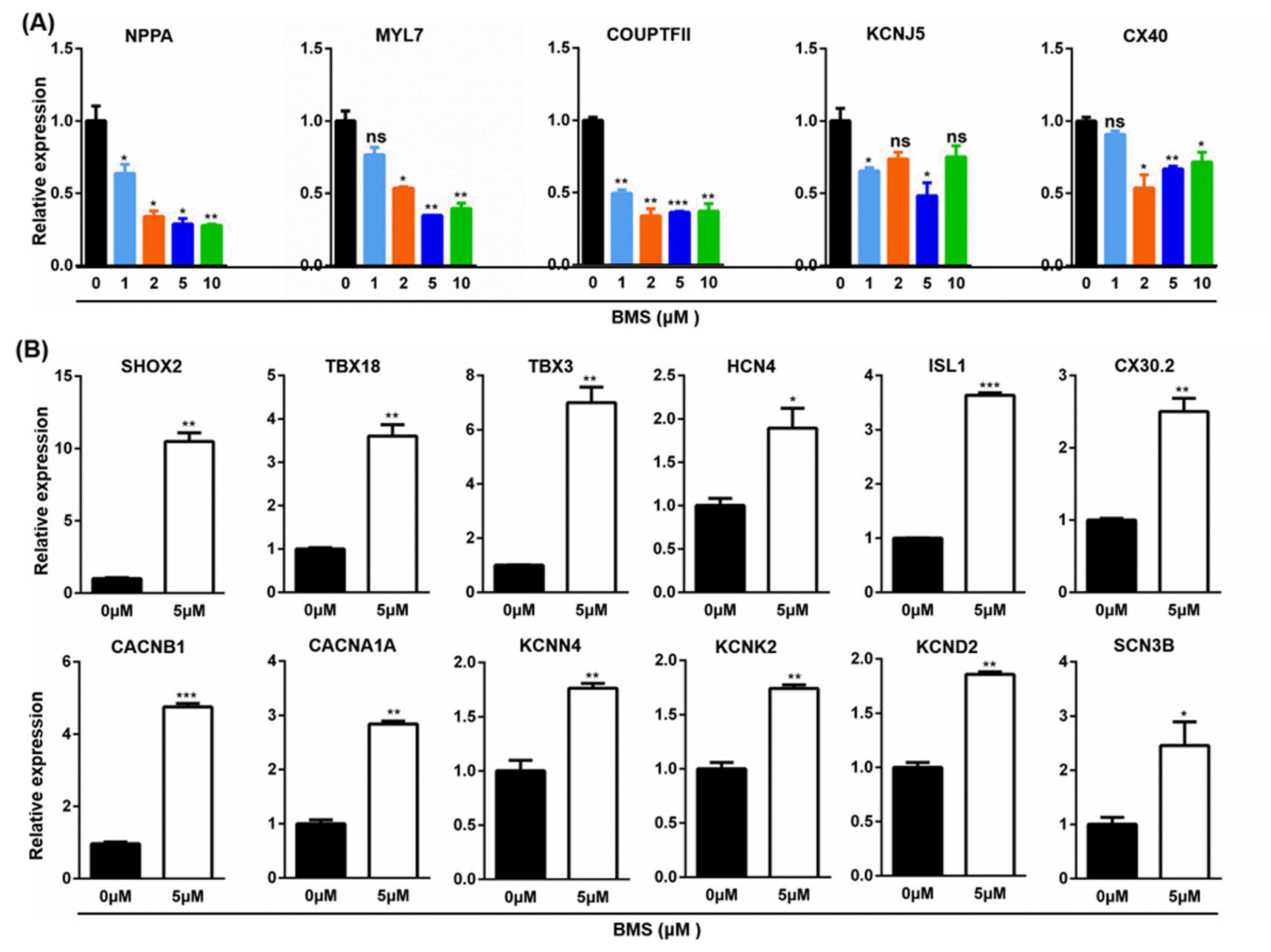
Fig. 5
Inhibition of RA signaling pathway by BMS biases the differentiation of hiPSC to Sinoatrial node-like cells. BMS at 5 μM was introduced on day 5 after the differentiation, and the expression of COUPTFII (A, B), TBX18 (C, D), and TBX3 (E, F) was evaluated by IF. Representative images and corresponding quantitations showing that inhibition of RA signaling pathway by BMS decreases the percentage of COUPTFII (A, B) positive cardio-myocytes and increases the percentage of TBX18-(C, D) and TBX3-positive (E, F) cardiomyocytes. Scale bars=100 μm (400× magnification).
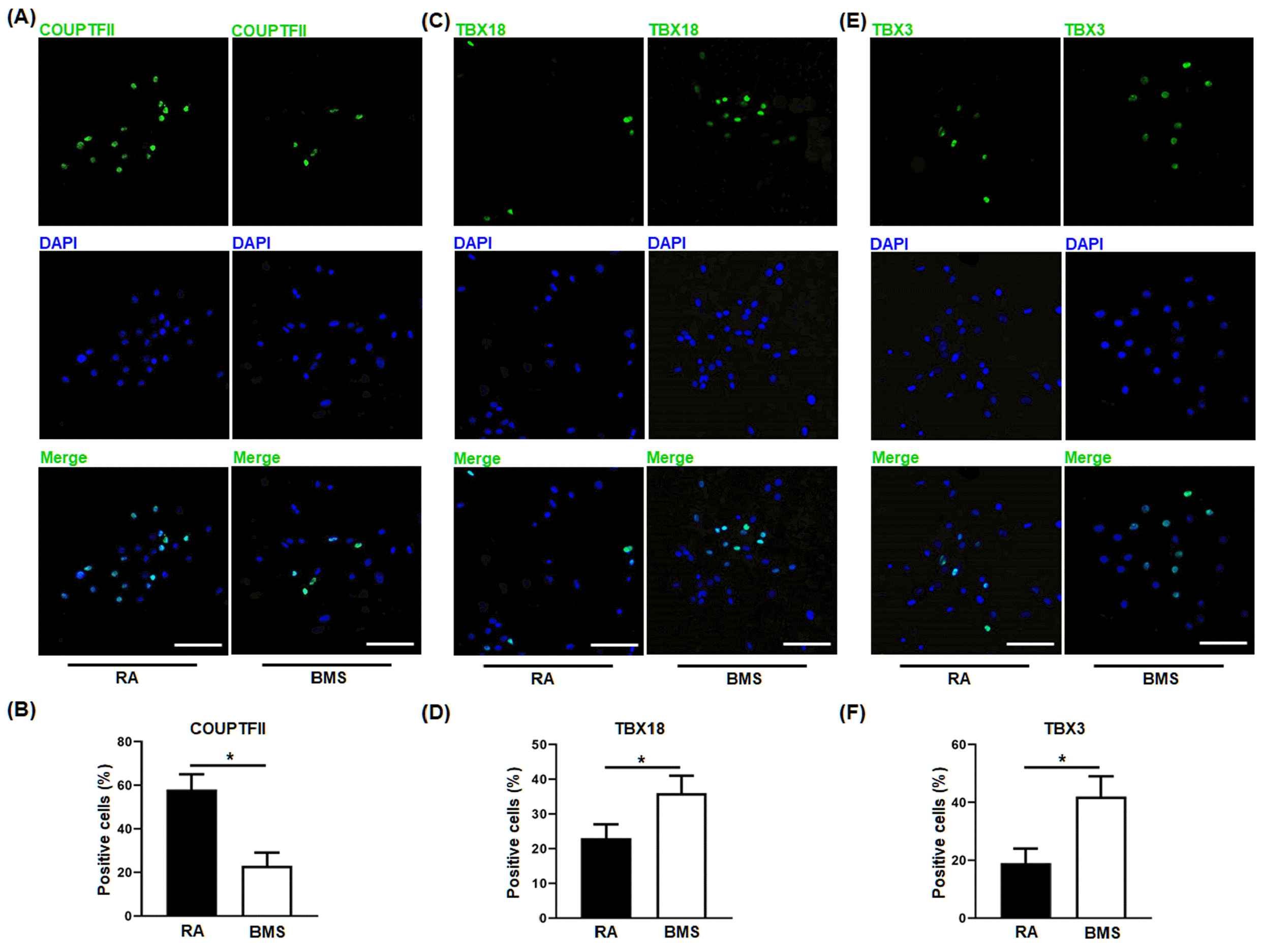
Fig. 6
Inhibition of RA signaling pathway by BMS biases the differentiation of hiPSC to Sinoatrial node-like cells. BMS at 5 μM was introduced on day 5 after the differentiation, and the expression of COUPTFII, cTNT+/SHOX2+, and cTNT+/NKX2.5− was evaluated by flow cytometry. Representative FACS plots showing that inhibition of RA signaling pathway by BMS decreases the percentage of COUPTFII positive cardiomyocytes (A) and increases the percentage of SHOX2+/cTNT+ (B) and cTNT+/NKX2.5− (C) cardio-myocytes.
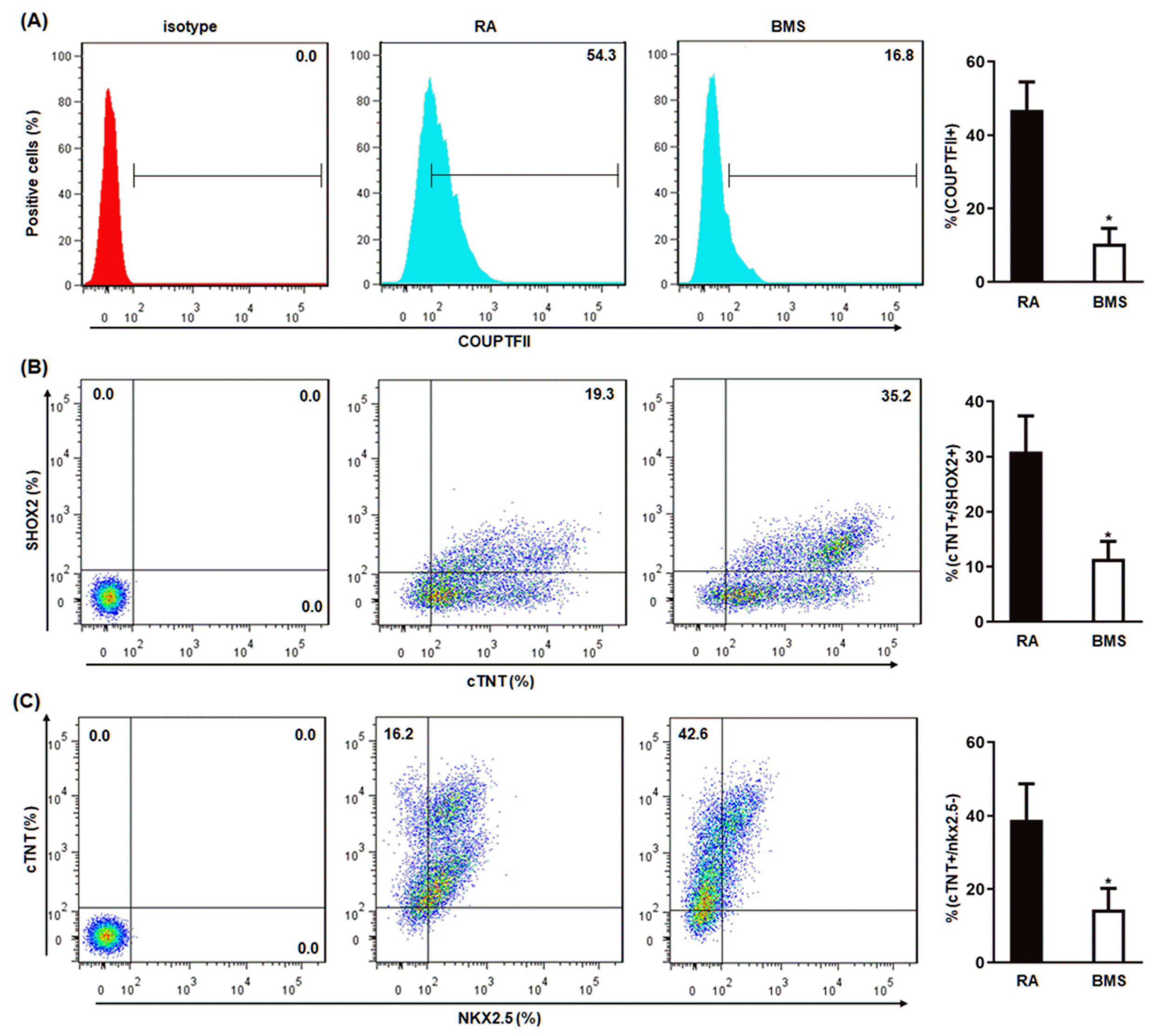
Fig. 7
Inhibition of RA signaling pathway by BMS biases the differentiation of cardiomyocytes possessing typical electrophysiological features of Sinoatrial node-like cells. BMS at 5 μM was introduced on day 5 after the differentiation, and the beating frequency and AP were analyzed after the differentiation on day 21 and 60 respectively. BMS significantly increased the beating frequency of cardiomyocytes (A), sensitized cardiomyocytes to TCN ion channel inhibitor (B), decreased the ratio of cardiomyocytes displaying typical atrial-like AP (C, middle panel; D, blue bar, 5/15), and increased the ratio of cardiomyocytes displaying typical pacemaker-like AP (C, bottom panel; D, green bar, 4/15), compared to RA group (10/15 and 2/15 respectively). Data in (A) and (B) are presented as ‘Mean±SD’ from recording of 7 cardiomyocytes, with *denoting p<0.05 and ****denoting p<0.0001.
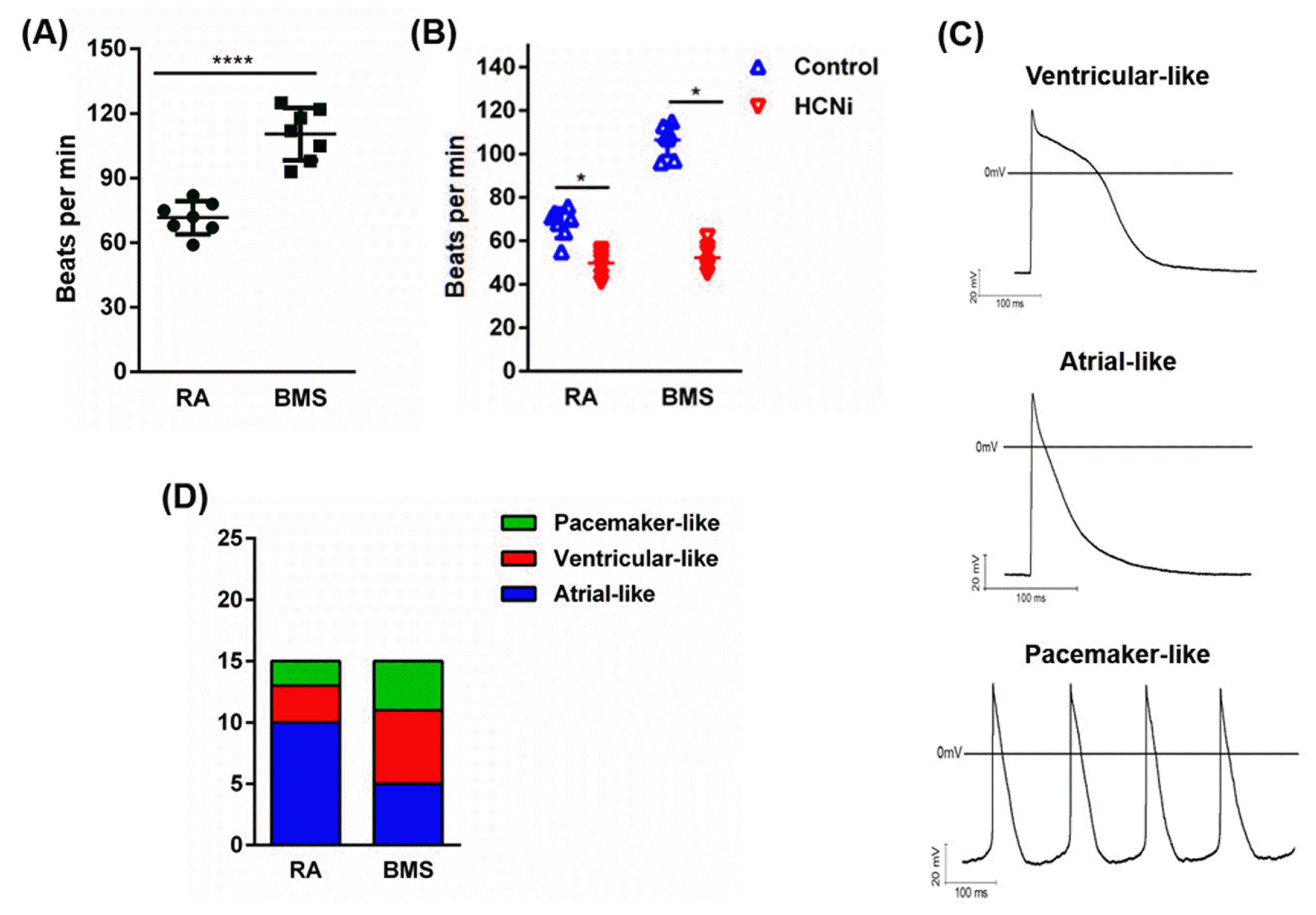
Fig. 8
Graphic abstract. Working model of biphasic effect of modulating RA signaling on the enrichment differentiation of atrial-like and sinoatrial node-like cells based on the GiWi method. During the cardiac mesoderm stage of pan cardio-myocytes differentiation from hiPSCS, activation of RA signaling pathway promotes biased differentiation of atrial-like cells. In contrast, enrichment differentiation of sinoatrial node-like cells could be enabled by RA inhibition.
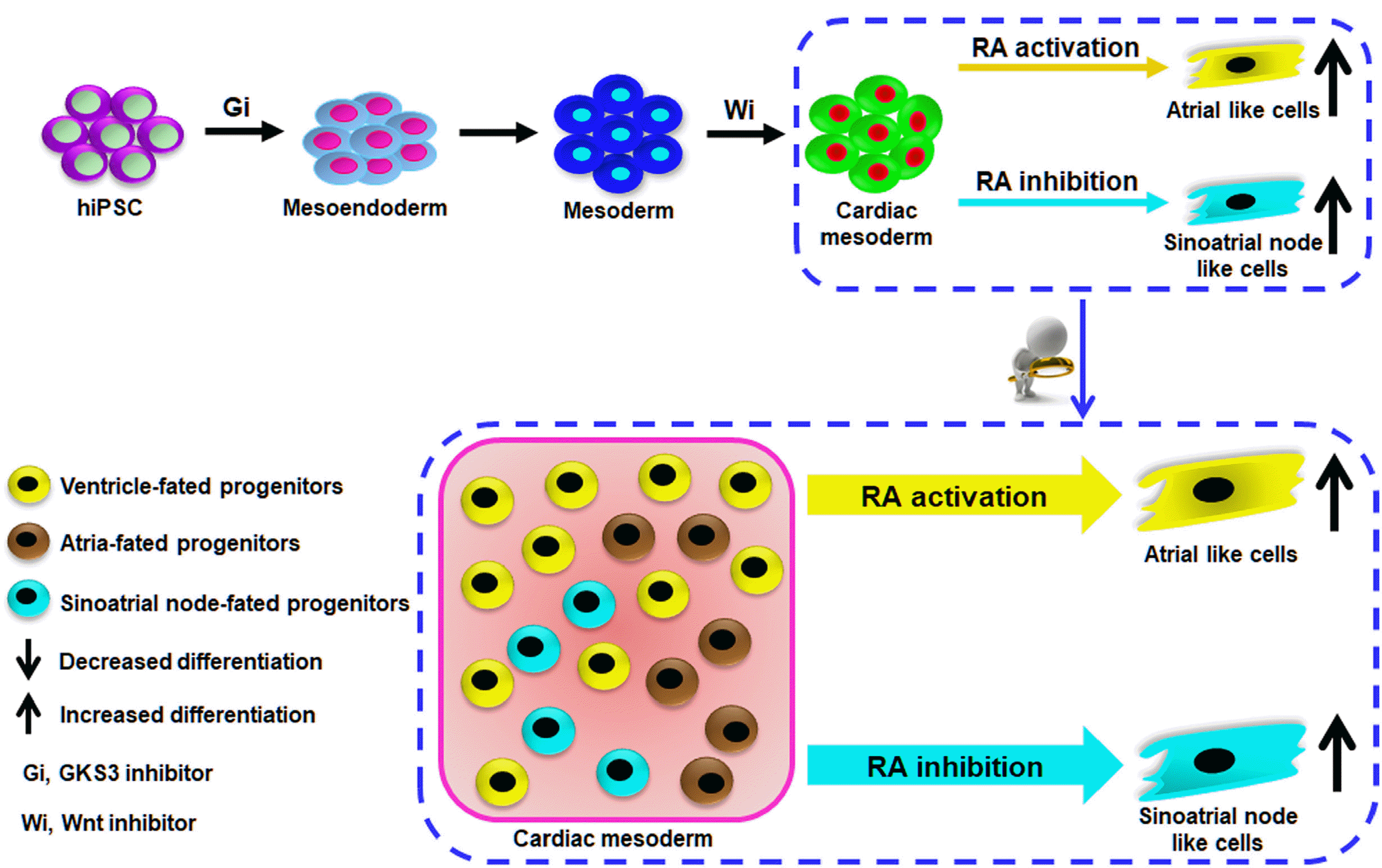