Abstract
Objective
Patients with mild ischemic stroke experience various sequela and residual symptoms, such as anxious behavior and deficits in movement. Few approaches have been proved to be effective and safe therapeutic approaches for patients with mild ischemic stroke by acute stroke. Sildenafil (SIL), a phosphodiesterase-5 inhibitor (PDE5i), is a known remedy for neurodegenerative disorders and vascular dementia through its angiogenesis and neurogenesis effects. In this study, we investigated the efficacy of PDE5i in the emotional and behavioral abnormalities in rats with mild ischemic stroke.
Methods
We divided the rats into four groups as follows (n=20, respectively) : group 1, naïve; group 2, middle cerebral artery occlusion (MCAo30); group 3, MCAo30+SIL-pre; and group 4, MCAo30+SIL-post. In the case of drug administration groups, single dose of PDE5i (sildenafil citrate, 20 mg/kg) was given at 30-minute before and after reperfusion of MCAo in rats. After surgery, we investigated and confirmed the therapeutic effect of sildenafil on histology, immunofluorescence, behavioral assays and neural oscillations.
Results
Sildenafil alleviated a neuronal loss and reduced the infarction volume. And results of behavior task and immunofluorescence shown possibility that anti-inflammation process and improve motor deficits sildenafil treatment after mild ischemic stroke. Furthermore, sildenafil treatment attenuated the alteration of theta-frequency rhythm in the CA1 region of the hippocampus, a known neural oscillatory marker for anxiety disorder in rodents, induced by mild ischemic stroke.
Ischemic stroke, one of the leading causes of death worldwide, is caused by interruption or significant impairment of blood supply to the brain leading to molecular and metabolic disorders such as brain injury, epileptic seizures, sensorimotor deficits, and vascular dementia [10,19,30,34,50,63]. Furthermore, while the symptoms of minor stroke or transient ischemic attack (TIA) are short term and are associated with fast recovery, patients with TIA experience various sequela and residual symptoms including deficits in emotion and cognition and anxiety disorder [41,71]. Especially, ischemia/reperfusion injury, which is characterized by a cascade of events leading to neuronal injury, facilitates the release of nitric oxide (NO), and the induction of programmed cell death [72].
Although diverse treatment methods for stroke have been developed, very few approaches have been proved to be effective and safe therapeutic approaches for patients with acute stroke [47]. For example, thrombolysis, which aims at rehabilitating blood flow to the infarct site of the brain tissue following ischemic attack, is an effective treatment for acute ischemic stroke [67]. In spite of its efficacy, thrombolysis treatment has several limitations, such as a comparatively short therapeutic time-window (up to 4.5 hours) and adverse side effects induced by hemorrhage [60]. Thus, new therapeutic approaches for acute ischemic stroke targeting different molecular and cellular substrates are necessary.
Phosphodiesterase-5 (PDE5) is a cyclic nucleotide phosphodiesterase expressed in several brain cell types and smooth muscle cells [17,35,54,65]. PDE5 activates the cyclic guanosine monophosphate pathway, one of the second messengers signaling, and triggers its hydrolysis to GMP [17,43]. Through protein kinase G (PKG), cGMP activates downstream intracellular mechanisms, such as the cyclic adenosine monophosphate-response element binding protein (CREB), a cellular transcription factor, involved in cell survival and synaptic plasticity [16,39]. Sildenafil (SIL), branded as Viagra®, is a selective inhibitor of cGMP-specific PDE5 with the efficacy of blocking the degradative action of PDE5 on cGMP [66]. Furthermore, previous imaging studies using positron emission tomography in mice indicated that sildenafil crosses the blood-brain barrier (BBB) and interact with endothelium and/or smooth muscle cells of brain vessels and PDE5 expressed in brain cells although mechanisms underlying their penetration into BBB have not yet been suggested [27]. PDE5 inhibitor (PDE5i) have therapeutic effects in erectile dysfunction, pulmonary hypertension, modulates neurogenesis and gliosis, improves cognition deficits, and has been shown to ameliorate amyloid burden in Alzheimer’s disease mouse model [24,77]. However, the therapeutic efficacy of PDE5i in anxiety disorder following mild stroke remains unclear; the prevalence of post-stroke anxiety is high and has been associated with an increased risk of incident stroke.
In this study, therefore, we characterized distinctive loss of neuronal cells, brain tissue damage, and emotional and behavioral abnormalities in rats with mild ischemic stroke. We hypothesized that the use of PDE5i is a novel therapeutic approach for the improvement of cerebral blood flow in the infarct region in the brain, and investigated the efficacy of PDE5i in emotional and behavioral abnormalities.
All animal studies and experimental procedures were approved by the Administrative Panel on Laboratory Animal Care of Soonchunhyang University (permit No. SCH16-0024). All possible efforts were made to avoid the suffering of the rats and to minimize the number used during the experiments.
Male Sprague-Dawley rats (8 weeks of age and 260–280 g of body weight) were used to establish a middle cerebral artery occlusion (MCAo) model. Rats were provided with a commercial diet and water ad libitum under controlled temperature, humidity and lighting conditions (light/dark cycle 12 : 12, and 22°C±2°C, 55%±5%).
Focal brain ischemia was induced using a relatively non-invasive technique as described previously [51]. Briefly, rats were anesthetized with 2% isoflurane in a mixture of oxygen and nitrous oxide gases (0.3 : 0.7), and body temperature was maintained at 37°C with a heating pad during surgery. The tip of a 4-0-monofilament nylon suture (Nylon suture; Medtronic PLC, Minneapolis, MN, USA) was coated with dental silicone to a thickness of 0.37±0.02 mm and a piece of the filament was coated with 0.01% (w/v) poly-L-lysine (Sigma-Aldrich, St. Luis, MO, USA). The monofilament was inserted into the left common carotid artery, and advanced from 18 mm point toward the internal carotid artery to occlude the ostium of the MCA. A laser Doppler probe (Millwey, Axminste, UK) was fixed on the parietal bone (1.5 mm posterior and 5 mm to the bregma point) to measure the local cortical blood flow supplied by the MCA during surgery. Successful occlusion was determined by 20% decrease to baseline in local cortical blood flow. Thirty-minute after brain ischemia, MCA was reperfused from transient ischemia (MCAo30), and the rats recovered from anesthesia within a few minutes. Seven days after surgery, the rats were screened using behavioral tasks of examining movement disorders, such as inability to extend the contralateral forelimb and circling towards the contralateral to infarct following neurological deficits, for verifying an induction of MCAo as described previously [51]. At designated time courses, the rats screened for the MCAo-related deficits in movement were used for anxiety-related behavioral tests, electrophysiology, and immunohistochemistry. Age-matched naïve rats were used as controls.
To evaluate whether sildenafil citrate has an effect on the improvement of diverse phenotypical sequela induced by mild ischemic stroke, PDE5i (sildenafil citrate, 20 mg/kg; Hanmi Parm., Seoul, Korea) was intraperitoneally (i.p.) administered into the rats. A single dose of PDE5i dissolved in 0.9% saline was given at 30-minute before and after reperfusion of MCAo respectively, as described previously [14]. We divided the rats into four groups as follows (n=20, respectively) : group 1, naïve; group 2, MCAo30; group 3, MCAo30+SIL-pre; and group 4, MCAo30+SIL-post.
To allow adaption to the environmental conditions of all behavioral assays, the rats were exposed to white noise for 30-minute before the ongoing behavioral tests, which is created by combining sounds of the identical frequency at 60 Hz.
Behavioral assay for locomotor activity and anxiety was carried out according to the procedures described previously [23,64]. The total distance moved was used as a parameter to evaluate the locomotor activity. The open-field box (60×60×40 cm; length, width, height) was placed in an isolated room without any clues. Each individual rat was placed in the openfield arena under diffusing light. The extent of their spontaneous movement in the open-field box within 30-minute was recoded, and analyzed using EthoVision 3.1 software (Noldus Information Technology, Wageningen, the Netherlands).
Behavioral assay for anxiety was performed as described previously. The light-dark box (45×27×27 cm; length, width, height) had two compartments, three fifths for the light compartment and two fifths for the dark compartment. The compartments were connected by an entrance (9×9 cm) located on the floor. Illumination was adjusted at the center of compartments to set the light compartment to 300 lux and the dark compartment to 0-1 lux. Each individual rat was placed in the light compartment and recorded with a video camera for 5-minute during exploration of the environmente.
Behavioral assay for anxiety was performed as described previously [64]. Each rat was placed in an elevated (60 cm above floor level) plus-maze with two opposite open arms (50×10 cm each) and two opposite closed arms (50×10 cm each) with 50 cm-high walls. Illumination was adjusted at the center of the plus maze to 200 lux. The number of entries into and the time spent on individual arms were measured for 5-minute.
The task was performed as previously described with minor modifications [6,32]. Y-maze with three identical arms was placed in an isolated room without a clue, and the arms were labeled A, B, and C. Animals were placed the end of one arm (40×5×20 cm; length, width, height) and recorded the number of arm entries and spontaneous alternations during an 8-minute period. To assess the direction preference, the number of turns to the ipsilateral direction, infarct side in the brain hemisphere, was analyzed. The average percentage of circling behavior in the direction ipsilateral to the infarct was calculated as (number of turn into an ipsilateral infarct) / (number of total turn) × 100.
LFP were recorded in the hippocampus of the rats as described previously with some modifications [36,74]. The rats were anesthetized by intraperitoneal injection of urethane (1.5 g/kg) and placed in a stereotaxic frame. Glass microelectrodes (microfilament capillary 1.2 outer diameter; 5–10 MΩ) filled with artificial cerebrospinal fluid (ACSF, in mM; NaCl 126, KCl 5, CaCl2 2, MgCl2 2, NaH2PO4 1.25, NaHCO3 26, D-glucose 10, pH 7.2) were used. The coordinates (in mm) referenced to bregma were as follows (mm to CA1) : 3.8 caudal, 2.5 lateral to bregma, and 2.9 depth. LFP recordings in vivo were performed with a QP511 AC amplifier (0.1–3000 Hz bandpass; GRASS Technologies, Kent County, RI, USA) and data were digitized (5 kHz) and analyzed using Clampfit 10.2 (Axon Instruments, San Jose, CA, USA). LFP was monitored for 2-hour. Analysis of the single-channel electrical traces was carried out using the Clampfit 10.2 software (Axon Instruments). To analyze changes in the normalized power of LFP, the amplitude spectrum of the normalized power was estimated by event frequency. The root mean square values were used to derive estimates of spectral power (mV2) in the 1 Hz frequency bins for each electrode site. Spectral power values were averaged across all epochs within a single base-line, and the resulting power was expressed as mV2/Hz. For each subject, fast fourier transform (FFT) of the epochs with a resolution of 0.61 Hz was computed for all the electrodes and then averaged. Non-overlapping hamming windows controlled spectral leakage. The FFT power values within each frequency between 1–50 Hz were averaged to create 50 non-overlapping <1 Hz frequency bins, because the frequency bands of interest were defined as θ (4–7 Hz) [1,23]. After LFP recording, renal histology and immunohistochemistry were conducted with a few animal specimens.
To measure the infarct size following mild ischemic stroke, the brains tissue obtained from the animals after decapitation were cooled at 4℃ to harden the tissues. Six or seven coronal sections of 2 mm thickness were made from the olfactory bulb to the cerebellum. Then, each coronal section was immersed for 20-minute into 2% 2,3,5-TTC (Sigma-Aldrich, St. Louis, MO, USA) solution at room temperature. The brain sections stained by TTC were captured using a model DP72 digital camera and DP2-BSW microscope digital camera software (Olympus, Tokyo, Japan), the ischemic damaged area in the infarcted hemisphere for each brain slice was measured using ImageJ (NIH, Bethesda, MD, USA). Quite complete want of TTC staining was defined as core area of infarct zone [9]. Pink colored-area in the damaged brain tissue was defined as penumbra placed periphery zone of core area, and red colored-area was defined as the undamaged normal brain tissues [26]. Total infarction volume was regarded as the sum of core area and penumbra. Damaged volume was calculated as the average percentage of the hemisphere volume and adjusted for edema as described previously [53]. Results are presented as the mean±standard error of mean (SEM) for the different animal groups. A p-value <0.01 or <0.05 was considered statistically significant by Bonferroni’s test.
Animals were transcardially perfused with phosphate buffered saline (PBS) followed by 4% paraformaldehyde in 0.1 M phosphate buffer (pH 7.4) under urethane anesthesia (1.5 g/kg, i.p.). The brains were removed and postfixed in the same fixative for 4-hour. The brain tissues were cryoprotected by infiltration with 30% sucrose overnight. Thereafter, the entire hippocampus was frozen and sectioned with a cryostat at 30 µm and the consecutive sections were transferred into six-well plates containing PBS. For the stereological study, every sixth section in the series throughout the entire hippocampus was used in some animals. To analyze the cell death induced by MCAo, the brain slices were mounted on gelatin-coated slides and each slide was stained with CV acetate (Sigma, St. Louis, MO, USA). After that, images were captured and used for the measurements of cell damage/death following mild ischemic attack using DP72 digital camera and DP2-BSW microscope digital camera software (Olympus).
The immunofluorescence staining was performed as described previously with some modifications [74]. Double immunofluorescence staining for S100 calcium-binding protein B (S100B) and aquaporin 4 (AQP4) was performed to identify the morphological changes following mild ischemic stroke with or without sildenafil treatments in the same hippocampal tissue. The brain slices were incubated overnight at room temperature in a mixture of mouse anti-S100B immunoglobulin G (IgG) (diluted 1 : 500; Sigma)/rabbit anti-AQP4 IgG (diluted 1 : 200; Alomone labs, Jerusalem, Israel). After washing three times for 10-minute with PBS, the slices were incubated in the mixture of Cy2- and Cy3-conjugated secondary antisera (diluted 1 : 200; Sigma) for 1-hour at room temperature. Then, these slices were incubated in DAPI (diluted 1 : 500; Invitrogen, Middlesex Country, MA, USA) as counterstaining for 15-minute at room temperature. After washing with PBS, the slices were placed on a slide and mounted with DPX (Sigma). All the images were captured using a model Fluoview FV10i and FV10i software (Olympus).
Quantification and statistical analysis of each data were performed as described in the previous described study with some modifications [74]. Optical fractionation was used to estimate the cell numbers. The technique combines optical dissection counting and fractionator sampling, and is a stereological method based on a well-designed, systematic random sampling method. By definition, the approach yields unbiased estimates of population number. Samples of deep tissue were used (optical dissector height [h], was 5 µm). Statistical analyses were performed using GraphPad Prism 7 (GraphPad Software, San Diego, CA, USA). All data obtained from the quantitative measurements were analyzed using one-way analysis of variance to determine statistical significance. Bonferroni’s test was used for post-hoc comparisons. Bonferroni’s test was used for post-hoc comparisons. Statistical significance was defined as p<0.05, p<0.01, and p<0.001.
We measured the infarct size following mild ischemic stroke by performing TTC staining of brain slices from the MCAo rats and sildenafil-treated MCAo rats three days after MCAo (Fig. 1A). Although infarct lesion was clearly observed in the brain slices of MCAo30 rat models as compared with naïve controls, infarct lesion in the brains of MCAo30 rats was significantly rehabilitated by sildenafil treatment (Fig. 1B). The infarction volume in the brains for both the a MCAo30+SIL-pre (F1,9=18.82, p<0.01; Fig. 1C), and MCAo30+SIL-post groups (F1,8=60.4, p<0.001; Fig. 1C) was significantly attenuated in comparison with that of the MCAo30 rats. To further evaluate the neuronal cell death/damage in mild ischemic stroke following MCAo30, we also performed the CV staining. The CV-labelled neurons were not seen in the infarct areas in the several brain regions, such as the striatum and the piriform cortex, of MCAo30 rats (Fig. 2B), however, these CV-positive neurons were seen in the same brain regions of the naïve controls (Fig. 2A). Notably, the CV-positive neurons were significantly enhanced both the MCAo30+SIL-pre (Fig. 2C) and MCAo30+SIL-post groups (Fig. 2D) in comparison with the MCAo30 group.
To verify the functional alterations of the astrocytes sheathing the blood vessels in the hippocampus and the penumbra of MCAo30 rats, we performed double immunofluorescence labelling of S100B and AQP4 (Fig. 3). In MCAo30 rats, S100B-positive immunoreactivities in the astrocytes and vessels in the hippocampal CA1 and the penumbra region were significantly increased as compared with naïve controls (Fig. 3A and B). The number of double-labelled, S100B and AQP4, astrocytes and vessels in the CA1 (F1,9=21.03, p<0.01; Fig. 3B and E) and the penumbra (F1,9=265.3, p<0.001; Fig. 3B and F) were also higher in the MCAo30 rats as compared to the naïve controls. On the other hand, the number of S100B- and AQP4-positive astrocytes and vessels in the CA1 and the penumbra region of both the MCAo30+SIL-pre (F1,10=16.98, p<0.01, CA1, Fig. 3C and E; F1,10=76.17, p<0.001, penumbra, Fig. 3C and F) and MCAo30+SIL-post (F1,10=10.63, p<0.01, CA1, Fig. 3D and E; F1,10=35.24, p<0.001, penumbra, Fig. 3D and F) was significantly reduced in comparison with that of the MCAo30 rats. However, double-labelled, S100B and AQP4, immunofluorescences in the hippocampal CA1 area of the MCAo30+SIL-pre group were down-regulated to the level of naïve controls (F1,9=7.20, p=0.19; Fig. 3C and E).
To examine whether sildenafil treatment alleviates anxiety disorder induced by MCAo30, we analyzed levels of anxiety-related behaviors of the MCAo rats and sildenafil-treated MCAo rats by performing elevated plus-maze and light/dark transition task (Fig. 4B and C). Anxiety-related behavior tests that may cause stress in the animals were conducted after open-field test and Y-maze test were performed (Fig. 4A). In the elevated plus-maze task, MCAo30 rats made fewer entries into the aversive open arms as compared to the naïve controls (F1,11=9.85, p<0.01; Fig. 4B). Especially, MCAo30+SIL-post group showed normal anxiety-like behavior, similar to the level of naïve rats (F1,10=7.31, p<0.05, MCAo30+SIL-post vs. MCAo30; Fig. 4B), however, MCAo30+SIL-pre group did not show improvement of MCAo30 induced anxiogenic behavior (F1,11=8.01, p<0.05, MCAo30+SIL-pre vs. naïve; Fig. 4B). The total number of entries into both, open and closed, arms of the MCAo30 rats was decreased in comparison with naïve controls (F1,11=10.72, p<0.01; Fig. 4C). Notably, these deficits in locomotion was improved in the both MCAo30+SIL-pre (F1,11=12.57, p<0.01, MCAo30+SIL-pre vs. MCAo30; Fig. 4C) and MCAo30+SIL-post groups (F1,11=5.72, p<0.05, MCAo30 +SIL-post vs. MCAo30; Fig. 4C), to a level similar to that of the naïve controls (F1,14=0.04, p=0.84, MCAo30+SIL-pre vs. naïve; F1,12=0.57, p=0.46, MCAo30+SIL-post vs. naïve; Fig. 4C). In the light-dark transition test, the time that the MCAo30 rat spent in the light chamber was significantly shorter than that of the naïve controls (F1,11=205.6, p<0.001, MCAo30 vs. naïve; Fig. 4E). Consistently, MCAo30 rats made fewer transitions from the dark to the light compartment than the naïve controls (F1,11=5.56, p<0.05, MCAo30 vs. naïve; Fig. 4F). Duration in the light compartment was increased in the MCAo30+SIL-pre (F1,11=21.47, p<0.001, MCAo30+SIL-post vs. MCAo30; Fig. 4E) and MCAo30+SIL-post (F1,11=10.22, p<0.01, MCAo30+SIL-post vs. MCAo30; Fig. 4E) groups in comparison with the MCAo30 rats. Furthermore, the MCAo30+SIL-post group showed alleviated anxious behavior, to a level similar to that of the naïve control (F1,11=1.78, p=0.20, MCAo30+SIL-post vs. naïve; Fig. 4F). There was high correlation between the infarct size and anxiogenic behavior (Pearson correlation coefficient r=-0.8392, p<0.01, Fig. 4D; r=-0.86914, p<0.01, Fig. 4G). Together, our findings demonstrate that sildenafil treatment attenuates anxiogenic behavior induced by mild ischemic stroke following MCAo in rats.
In the open-field assay, MCAo30 rats showed deficits in locomotor activity in comparison with the naïve controls (F1,24=47.67, p<0.001, MCAo30 vs. naïve; Fig. 5A and B). Total distance moved in the open-field arena was increased in both the MCAo30+SIL-pre (F1,13=29.18, p<0.001, MCAo30+SIL-pre vs. MCAo30; Fig. 5B) and the MCAo30+SIL-post (F1,15=6.6, p<0.05, MCAo30+SIL-post vs. MCAo30; Fig. 5B) groups in comparison with MCAo30 rats. In addition, MCAo30 rat models showed left rotation behavior, and the number of ipsilateral circling turns was increased in comparison with naïve controls (F1,10=20.56, p<0.01, MCAo30 vs. naïve; Fig. 5C). Interestingly, we observed that these motor defects were ameliorated by sildenafil treatment (F1,10=18.09, p<0.01, MCAo30+SIL-pre vs. MCAo30; F1,10=20.13, p<0.01, MCAo30+SIL-post vs. MCAo30; Fig. 5C) and found a correlation coefficient between infarct size and movement disorder (Pearson correlation coefficient r=-0.92008, p<0.001, Fig. 5D).
Furthermore, we noticed that the MCAo30 rats showed preference for left direction in the Y-maze (Fig. 5E). We measured direction preference in the Y-maze task as described previously, and confirmed that the MCAo30 rats showed higher preference for left direction, ipsilateral side of the MCAo30 in the left hemisphere of the brain, than naïve controls (F1,17=133.59, p<0.001, MCAo30 vs. naïve; Fig. 5F). We also found that the direction preference induced by MCAo could be improved by sildenafil treatment (F1,15=13.46, p<0.01, MCAo30+SIL-pre vs. MCAo30; F1,13=78.51, p<0.001, MCAo30+SIL-post vs. MCAo30; Fig. 5F), with the MCAo30+SIL-post group showing a normal level of direction preference in comparison with naïve controls (F1,14=2.13, p=0.16, MCAo30+SIL-post vs. naïve; Fig. 5F). Thus, our findings indicate that sildenafil treatment alleviates deficits of locomotion and direction preference induced by mild ischemic stroke following MCAo in rats.
To examine 1) whether alteration of neural oscillations at 4–7 Hz, associated with anxiety disorder [1,23,49,59], can be induced by MCAo, if so, 2) whether altered theta-frequency oscillations can be recovered by sildenafil treatment, we performed LFP recordings in the hippocampal CA1 region of MCAo30 rats and sildenafil-treated MCAo30 rats under urethane anesthesia (1.5 g/kg, i.p.) (Fig. 6A and B). Power spectral analysis showed that power of theta-frequency oscillations was significantly increased in the MCAo30 in comparison with that of the naïve controls (F1,8=8.78, p<0.05, MCAo30 vs. naïve; Fig. 6C). Importantly, sildenafil treatment alleviated theta power to a level similar to that of the naïve rats (F1,8=33.25, p<0.001, MCAo30+SIL-pre vs. MCAo30; F1,8=16.77, p<0.01, MCAo30+SIL-post vs. MCAo30; Fig. 6C). Normalized LFP power of MCAo30+SIL-pre group was decreased to the level of naïve controls (F1,8=2.59, p=0.14, MCAo30+SIL-pre vs. naïve; F1,8=12.32, p<0.05, MCAo30+SIL-pre vs. MCAo30; Fig. 6D), however, the level in MCAo30+SIL-post group was higher than that of the naïve group (MCAo30, F1,8=8.28, p<0.05; MCAo30+SIL-post, F1,8=5.21, p<0.001; Fig. 6D). Together, these results indicate that sildenafil treatment alleviates alteration of theta-frequency oscillations, a neural oscillatory marker for anxiety disorder, induced by mild ischemic stroke in rats.
In this study, we investigated the therapeutic effect of sildenafil on anxiogenic behavior and movement disorder induced by mild ischemic stroke in rats and demonstrated the use of PDE5i as a novel therapeutic approach for the improvement of cerebral blood flow in the infarct region in the brain.
Consistent with a previous study, a large infarct area, likely an edema lesion, was observed in the striatum and the piriform cortex following mild stroke (Figs. 1B and 2B) [52]. In addition, neuronal population labelled by CV were not observable in this infarcted region of the brain. Recent studies in rodents also suggested that treatment with PDE5i increased cerebral blood flow, and improved brain edema, infarct volume, neuronal damage, and neurological function after ischemic stroke [14,75,76]. PDE5i was effective in increasing levels of synaptophysin, brain derived neurotrophic factor/tropomyosin-related kinase (TrK) B, nerve growth factor/TrkA, and postsynaptic density protein 95/neuronal NO synthases [14]. Furthermore, PDE5i prevented neurotoxicity induced by reactive oxygen species (ROS), and has been regarded as a possible therapeutic tool for the brain edema, inflammation and neuronal damage in the central nervous system [45]. Here we found that infarction volume induced by mild ischemic stroke was significantly reduced in the brains of both the MCAo30+SIL-pre- and MCAo30+SIL-post groups (Figs. 1 and 2). Sildenafil inhibits cGMP-specific PDE5 and increases cGMP, its effect is similar to the upregulation of NO [31]. Enhancing NO-cGMP signaling by sildenafil increases blood flow and has neuroprotective effects in the rat model of hypoxic-ischemic brain injury [13]. Furthermore, upregulation of cGMP signaling by sildenafil activates cGMP-PKG leading to decreased levels of intracellular calcium ions [3,18]. Ca2+ ions play a vital role as a major initiator and activator in the ischemic cell death pathways [15]. Elevated levels of intracellular Ca2+ ions in serum plays a role in decrease of infarct volume and outcome in ischemic stroke patients [11,12,48]. Ca2+ accumulation triggers mitochondrial damage and contributes to delayed neuronal death as well as ROS [15,40]. Especially, reducing level of Ca2+ by sildenafil in the mitochondria plays a protective role in the neuronal cells of hippocampus [5,61]. These results indicate that downregulation of mitochondrial calcium signaling caused by sildenafil treatment may contribute to reducing infarction volume induced by mild ischemic stroke.
Previously, diverse outcomes of sildenafil treatment in the myocardial infarct size of rabbits have been shown, and these results were influenced by dosage, difference of sildenafil treatment time after stroke [33]. In our present study, MCAo30 +SIL-post-treatment were more effective on damage following mild ischemic stroke than MCAo30+SIL-pre-treatment. Antiinflammatory effects of sildenafil provide protection against lesion extension following treatment of sildenafil after surgical in permanent MCAo of neonatal mice [42]. These results may suggest that sildenafil is beneficial for the therapy of mild ischemic stroke patients. Sildenafil evokes neurogenesis and reduces neurological deficits through increased levels of cGMP dependent on the activation of the mitogen-activated protein kinase [55]. Increasing of neural stem cells proliferation by cGMP enhanced neurogenesis, and these may result from decreasing of infarction volume by ischemia. Enhancement of neurogenesis may contribute to functional recovery after sildenafil treatment. For more information, biochemical and pharmacological experiments are required to reveal the efficacy of sildenafil in this process.
Increased S100B expression was observed in blood vessels and astrocytes, as swelling form of cell body, in the hippocampus following MCAo (Fig. 3). Previously, upregulation of S100B, glial fibrillary acidic protein, and neuron specific enolase in the blood of patients with stroke, brain trauma, and hypoxic encephalopathy were reported [70]. S100B is involved in regulating the calcium influxes caused by the reaction of astrocytes to injury, and stimulating astrocytic proliferation via interaction with the transcription factors [7,8,57,58]. In the present study, we observed that S100B expression was decreased in the hippocampal CA1 and the penumbra of both MCAo30+SIL-pre and MCAo30+SIL-post indicating neuroprotective efficacies and the novelty of sildenafil as a therapeutic agent (Fig. 3). AQP4 regulates extracellular matrix volume, potassium buffering, cerebrospinal fluid circulation, neuro-inflammation, osmosensation, and calcium signaling [44]. It has been detected in the astrocytic end-feet, and encapsulates the blood vessels in a similar way as S100B [4,46,70]. AQP4 expression was enhanced at 30-minute after permanent MCAo [38]. However, other studies reported downregulation, and sudden loss of AQP4, and sudden loss of the AQP4 in astrocytic end-feet following transient ischemia in regions of vascular damage of the post-ischemic phase [21,22,62]. These discrepancies may result from the varying severity of ischemic stroke. In the present study, markedly suppressed co-expression of AQP4 and S100B in the astrocytic structures of the MCAo30+SIL-pre and MCAo30+SIL-post (Fig. 3) indicate that treatment with sildenafil effectively improve brain infarction induced by MCAo, and can be regarded as a neuroprotective reagent. These results further strengthen the previous reports on the neuroprotective effects of sildenafil. In addition, our findings support the reports that S100B down-regulation after treatment with sildenafil is involved in the anti-inflammation process through the inhibition of astrogliosis, and that the brain damage induced by stroke may be related to functional alteration of AQP4 and S100B after mild ischemic stroke. However, further studies are needed to confirm this hypothesis.
Based on our findings, anxiety disorder (Fig. 4) and locomotion deficits (Fig. 5) in MCAo30 rats were significantly reduced with sildenafil therapy. Previous studies showed that NO-cGMP pathway is associated with anxious behaviors, although there are also arguments of the neural mechanism underlying these findings [20,25,37,68,69]. In the present study, sildenafil treatment improved anxiogenic behavior induced by mild ischemic stroke in the elevated plus maze and light-dark transition test (Fig. 4). In addition, the MCAo in rats caused hypolocomotion and stereotypic events with expected simultaneous enhancement in resting duration following mild ischemic stroke [73]. cGMP upregulation promotes the proliferation of motor neurons. Both PDE5i and 8q-cGMP, an analogue of cGMP, provided neuroprotection against neurotoxicity induced by ROS [45]. Similarly, the results of our behavioral experiments showed marked improvement in locomotion deficits of both the MCAo30+SIL-pre and the MCAo30+SIL-post groups (Fig. 5A and B). A spontaneous alteration assay using Y-maze task has been used for measuring working memory, like short-term memory in a new environment, in rodents [56]. However, contrary to the objectives of the experiment, the MCAo rat models showed a preference for the left direction, the ipsilateral side of MCAo in the left hemisphere of the brain, in the open-field and Y-maze test (Fig. 5). Notably, preference for the left direction of the MCAo30 rats in the Y-maze test was alleviated by sildenafil treatment (Fig. 5F), and the MCAo30+SIL-post group showed a normal level of direction preference similar to that of the naïve controls (Fig. 5F). Thus, our findings indicate that motor deficits following mild ischemic stroke in rats can be improved by sildenafil treatment.
Altered theta-frequency oscillations in the hippocampus are associated with anxiety disorder in rodents [1,49,59]. Enhanced hippocampal theta rhythm was observed during fear responses and anxiogenic states, supporting the involvement of hippocampal theta rhythm in the anxiety [1,2,28,29]. Based on our anxiety-related behavioral assays, sildenafil treatment alleviated alteration of theta-frequency oscillations induced by MCAo30 (Fig. 6). These findings suggest a possible role of sildenafil, a selective PDE5i, in the improvement of theta signal and phenotype anxiety and therefore improvement of anxiety disorder and movement deficits induced by mild ischemic stroke in rats.
Our findings in the present study suggest sildenafil as an effective pharmacological agent for preventing neuronal damage in the brain. In addition, our study extends the definitive knowledge of diverse phenotypical sequela and functional complications of mild ischemic stroke, and provides preclinical evidence for PDE5i efficacy in anxiety and movement disorders as well as other residue disorders induced by mild ischemic stroke. But, when applying the results of animal experiments to humans, should be considered to a lot of variables that dosage, frequency and way. As well, these hypotheses about enhancing mitochondrial death, neurogenesis by cGMP and functional alteration of AQP4 and S100B by sildenafil is required definitive knowledge about pathophysiology mechanisms.
Notes
Author contributions
Conceptualization : YHY, SWK, JK, JSO, DSK; Data curation : YHY, SWK, JK; Formal analysis : YS, HI, SJK, DYY; Funding acquisition : JSO, DSK; Methodology : MRL, DKP; Project administration : JSO, DSK; Visualization : YHY, SWK, JK; Writing - original draft : YHY, SWK, JK, JSO, DSK; Writing - review & editing : YHY, SWK, JK, YS, HI, SJK, DYY, MRL, DKP, JSO, DSK
ACKNOWLEDGMENTS
This work was supported by the Soonchunhyang University and a Basic Science Research Program (NRF-2017R1D1A1B05036195 & NRF-2020R1F1A1066362) through the National Research Foundation of Korea funded by the Ministry of Science.
References
1. Adhikari A, Topiwala MA, Gordon JA. Synchronized activity between the ventral hippocampus and the medial prefrontal cortex during anxiety. Neuron. 65:257–269. 2010.
2. Adhikari A, Topiwala MA, Gordon JA. Single units in the medial prefrontal cortex with anxiety-related firing patterns are preferentially influenced by ventral hippocampal activity. Neuron. 71:898–910. 2011.
3. Ala M, Mohammad Jafari R, Dehpour AR. Sildenafil beyond erectile dysfunction and pulmonary arterial hypertension: thinking about new indications. Fundam Clin Pharmacol. 35:235–259. 2021.
4. Amiry-Moghaddam M, Otsuka T, Hurn PD, Traystman RJ, Haug FM, Froehner SC, et al. An alpha-syntrophin-dependent pool of AQP4 in astroglial end-feet confers bidirectional water flow between blood and brain. Proc Natl Acad Sci U S A. 100:2106–2111. 2003.
5. Ascah A, Khairallah M, Daussin F, Bourcier-Lucas C, Godin R, Allen BG, et al. Stress-induced opening of the permeability transition pore in the dystrophin-deficient heart is attenuated by acute treatment with sildenafil. Am J Physiol Heart Circ Physiol. 300:H144–H153. 2011.
6. Bak J, Pyeon HI, Seok JI, Choi YS. Effect of rotation preference on spontaneous alternation behavior on Y maze and introduction of a new analytical method, entropy of spontaneous alternation. Behav Brain Res. 320:219–224. 2017.
7. Barger SW, Van Eldik LJ. S100 beta stimulates calcium fluxes in glial and neuronal cells. J Biol Chem. 267:9689–9694. 1992.
8. Baudier J, Delphin C, Grunwald D, Khochbin S, Lawrence JJ. Characterization of the tumor suppressor protein p53 as a protein kinase C substrate and a S100b-binding protein. Proc Natl Acad Sci U S A. 89:11627–11631. 1992.
9. Bederson JB, Pitts LH, Germano SM, Nishimura MC, Davis RL, Bartkowski HM. Evaluation of 2,3,5-triphenyltetrazolium chloride as a stain for detection and quantification of experimental cerebral infarction in rats. Stroke. 17:1304–1308. 1986.
10. Bogousslavsky J, Martin R, Regli F, Despland PA, Bolyn S. Persistent worsening of stroke sequelae after delayed seizures. Arch Neurol. 49:385–388. 1992.
11. Borah M, Dhar S, Gogoi DM, Ruram AA. Association of serum calcium levels with infarct size in acute ischemic stroke: observations from northeast india. J Neurosci Rural Pract. 7(Suppl 1):S41–S45. 2016.
12. Buck BH, Liebeskind DS, Saver JL, Bang OY, Starkman S, Ali LK, et al. Association of higher serum calcium levels with smaller infarct volumes in acute ischemic stroke. Arch Neurol. 64:1287–1291. 2007.
13. Charriaut-Marlangue C, Nguyen T, Bonnin P, Duy AP, Leger PL, Csaba Z, et al. Sildenafil mediates blood-flow redistribution and neuroprotection after neonatal hypoxia-ischemia. Stroke. 45:850–856. 2014.
14. Chen XM, Wang NN, Zhang TY, Wang F, Wu CF, Yang JY. Neuroprotection by sildenafil: neuronal networks potentiation in acute experimental stroke. CNS Neurosci Ther. 20:40–49. 2014.
15. Chung JW, Ryu WS, Kim BJ, Yoon BW. Elevated calcium after acute ischemic stroke: association with a poor short-term outcome and longterm mortality. J Stroke. 17:54–59. 2015.
16. Ciani E, Guidi S, Bartesaghi R, Contestabile A. Nitric oxide regulates cGMP-dependent cAMP-responsive element binding protein phosphorylation and Bcl-2 expression in cerebellar neurons: implication for a survival role of nitric oxide. J Neurochem. 82:1282–1289. 2002.
17. Corbin JD, Francis SH. Cyclic GMP phosphodiesterase-5: target of sildenafil. J Biol Chem. 274:13729–13732. 1999.
18. Cruz-Burgos M, Losada-Garcia A, Cruz-Hernández CD, Cortés-Ramírez SA, Camacho-Arroyo I, Gonzalez-Covarrubias V, et al. New approaches in oncology for repositioning drugs: the case of PDE5 inhibitor sildenafil. Front Oncol. 11:627229. 2021.
19. Deb P, Sharma S, Hassan KM. Pathophysiologic mechanisms of acute ischemic stroke: an overview with emphasis on therapeutic significance beyond thrombolysis. Pathophysiology. 17:197–218. 2010.
20. Eroğlu L, Cağlayan B. Anxiolytic and antidepressant properties of methylene blue in animal models. Pharmacol Res. 36:381–385. 1997.
21. Friedman B, Schachtrup C, Tsai PS, Shih AY, Akassoglou K, Kleinfeld D, et al. Acute vascular disruption and aquaporin 4 loss after stroke. Stroke. 40:2182–2190. 2009.
22. Frydenlund DS, Bhardwaj A, Otsuka T, Mylonakou MN, Yasumura T, Davidson KG, et al. Temporary loss of perivascular aquaporin-4 in neocortex after transient middle cerebral artery occlusion in mice. Proc Natl Acad Sci U S A. 103:13532–13536. 2006.
23. Gangadharan G, Shin J, Kim SW, Kim A, Paydar A, Kim DS, et al. Medial septal GABAergic projection neurons promote object exploration behavior and type 2 theta rhythm. Proc Natl Acad Sci U S A. 113:6550–6555. 2016.
24. García-Barroso C, Ricobaraza A, Pascual-Lucas M, Unceta N, Rico AJ, Goicolea MA, et al. Tadalafil crosses the blood-brain barrier and reverses cognitive dysfunction in a mouse model of AD. Neuropharmacology. 64:114–123. 2013.
25. Gilhotra N, Dhingra D. Involvement of NO-cGMP pathway in antianxiety effect of aminoguanidine in stressed mice. Prog Neuropsychopharmacol Biol Psychiatry. 33:1502–1507. 2009.
26. Gill R, Sibson NR, Hatfield RH, Burdett NG, Carpenter TA, Hall LD, et al. A comparison of the early development of ischaemic damage following permanent middle cerebral artery occlusion in rats as assessed using magnetic resonance imaging and histology. J Cereb Blood Flow Metab. 15:1–11. 1995.
27. Gómez-Vallejo V, Ugarte A, García-Barroso C, Cuadrado-Tejedor M, Szczupak B, Dopeso-Reyes IG, et al. Pharmacokinetic investigation of sildenafil using positron emission tomography and determination of its effect on cerebrospinal fluid cGMP levels. J Neurochem. 136:403–415. 2016.
28. Gordon JA, Lacefield CO, Kentros CG, Hen R. State-dependent alterations in hippocampal oscillations in serotonin 1A receptor-deficient mice. J Neurosci. 25:6509–6519. 2005.
29. Hoeller AA, Duzzioni M, Duarte FS, Leme LR, Costa AP, Santos EC, et al. GABA-A receptor modulators alter emotionality and hippocampal theta rhythm in an animal model of long-lasting anxiety. Brain Res. 1532:21–31. 2013.
30. Kilpatrick CJ, Davis SM, Tress BM, Rossiter SC, Hopper JL, Vandendriesen ML. Epileptic seizures in acute stroke. Arch Neurol. 47:157–160. 1990.
31. Kim KK, Kim DG, Ku YH, Lee YJ, Kim WC, Kim OJ, et al. Bilateral cerebral hemispheric infarction associated with sildenafil citrate (Viagra) use. Eur J Neurol. 15:306–308. 2008.
32. Koh HY, Kim D, Lee J, Lee S, Shin HS. Deficits in social behavior and sensorimotor gating in mice lacking phospholipase Cbeta1. Genes Brain Behav. 7:120–128. 2008.
33. Kolettis TM, Kontaras K, Spartinos I, Maniotis C, Varnavas V, Koutouzis M, et al. Dose-dependent effects of sildenafil on post-ischaemic left ventricular function in the rat isolated heart. J Pharm Pharmacol. 62:346–351. 2010.
34. Krishnamurthi RV, Feigin VL, Forouzanfar MH, Mensah GA, Connor M, Bennett DA, et al. Global and regional burden of first-ever ischaemic and haemorrhagic stroke during 1990-2010: findings from the global burden of disease study 2010. Lancet Glob Health. 1:e259–e281. 2013.
35. Kruuse C, Khurana TS, Rybalkin SD, Birk S, Engel U, Edvinsson L, et al. Phosphodiesterase 5 and effects of sildenafil on cerebral arteries of man and guinea pig. Eur J Pharmacol. 521:105–114. 2005.
36. Kwak SE, Kim JE, Kim SC, Kwon OS, Choi SY, Kang TC. Hyperthermic seizure induces persistent alteration in excitability of the dentate gyrus in immature rats. Brain Res. 1216:1–15. 2008.
37. Liebenberg N, Harvey BH, Brand L, Wegener G, Brink CB. Chronic treatment with the phosphodiesterase type 5 inhibitors sildenafil and tadalafil display anxiolytic effects in flinders sensitive line rats. Metab Brain Dis. 27:337–340. 2012.
38. Lu H, Hu H, He ZP. Reperfusion of the rat brain tissues following acute ischemia: the correlation among diffusion-weighted imaging, histopathology, and aquaporin-4 expression. Chin Med J (Engl). 124:3148–3153. 2011.
39. Lu YF, Kandel ER, Hawkins RD. Nitric oxide signaling contributes to latephase LTP and CREB phosphorylation in the hippocampus. J Neurosci. 19:10250–10261. 1999.
40. Ludhiadch A, Sharma R, Muriki A, Munshi A. Role of calcium homeostasis in ischemic stroke: a review. CNS Neurol Disord Drug Targets. 21:52–61. 2022.
41. Moran GM, Fletcher B, Feltham MG, Calvert M, Sackley C, Marshall T. Fatigue, psychological and cognitive impairment following transient ischaemic attack and minor stroke: a systematic review. Eur J Neurol. 21:1258–1267. 2014.
42. Moretti R, Leger PL, Besson VC, Csaba Z, Pansiot J, Di Criscio L, et al. Sildenafil, a cyclic GMP phosphodiesterase inhibitor, induces microglial modulation after focal ischemia in the neonatal mouse brain. J Neuroinflammation. 13:95. 2016.
43. Mullershausen F, Friebe A, Feil R, Thompson WJ, Hofmann F, Koesling D. Direct activation of PDE5 by cGMP: long-term effects within NO/cGMP signaling. J Cell Biol. 160:719–727. 2003.
44. Nagelhus EA, Ottersen OP. Physiological roles of aquaporin-4 in brain. Physiol Rev. 93:1543–1562. 2013.
45. Nakamizo T, Kawamata J, Yoshida K, Kawai Y, Kanki R, Sawada H, et al. Phosphodiesterase inhibitors are neuroprotective to cultured spinal motor neurons. J Neurosci Res. 71:485–495. 2003.
46. Nielsen S, Nagelhus EA, Amiry-Moghaddam M, Bourque C, Agre P, Ottersen OP. Specialized membrane domains for water transport in glial cells: high-resolution immunogold cytochemistry of aquaporin-4 in rat brain. J Neurosci. 17:171–180. 1997.
47. O’Collins VE, Macleod MR, Donnan GA, Horky LL, Van der Worp BH, Howells DW. 1,026 experimental treatments in acute stroke. Ann Neurol. 59:467–477. 2006.
48. Ovbiagele B, Starkman S, Teal P, Lyden P, Kaste M, Davis SM, et al. Serum calcium as prognosticator in ischemic stroke. Stroke. 39:2231–2236. 2008.
49. Padilla-Coreano N, Bolkan SS, Pierce GM, Blackman DR, Hardin WD, Garcia-Garcia AL, et al. Direct ventral hippocampal-prefrontal input is required for anxiety-related neural activity and behavior. Neuron. 89:857–866. 2016.
50. Pantoni L, Garcia JH, Gutierrez JA. Cerebral white matter is highly vulnerable to ischemia. Stroke. 27:1641–1646. 1996.
51. Park JH, Cho JH, Ahn JH, Choi SY, Lee TK, Lee JC, et al. Neuronal loss and gliosis in the rat striatum subjected to 15 and 30 minutes of middle cerebral artery occlusion. Metab Brain Dis. 33:775–784. 2018.
52. Popp A, Jaenisch N, Witte OW, Frahm C. Identification of ischemic regions in a rat model of stroke. PLoS One. 4:e4764. 2009.
53. Reglodi D, Tamás A, Somogyvári-Vigh A, Szántó Z, Kertes E, Lénárd L, et al. Effects of pretreatment with PACAP on the infarct size and functional outcome in rat permanent focal cerebral ischemia. Peptides. 23:2227–2234. 2002.
54. Rybalkin SD, Rybalkina IG, Feil R, Hofmann F, Beavo JA. Regulation of cGMP-specific phosphodiesterase (PDE5) phosphorylation in smooth muscle cells. J Biol Chem. 277:3310–3317. 2002.
55. Santos AI, Carreira BP, Nobre RJ, Carvalho CM, Araújo IM. Stimulation of neural stem cell proliferation by inhibition of phosphodiesterase 5. Stem Cells Int. 2014:878397. 2014.
56. Sarter M, Bodewitz G, Stephens DN. Attenuation of scopolamine-induced impairment of spontaneous alteration behaviour by antagonist but not inverse agonist and agonist beta-carbolines. Psychopharmacology (Berl). 94:491–495. 1988.
57. Scotto C, Deloulme JC, Rousseau D, Chambaz E, Baudier J. Calcium and S100B regulation of p53-dependent cell growth arrest and apoptosis. Mol Cell Biol. 18:4272–4281. 1998.
58. Selinfreund RH, Barger SW, Pledger WJ, Van Eldik LJ. Neurotrophic protein S100 beta stimulates glial cell proliferation. Proc Natl Acad Sci U S A. 88:3554–3558. 1991.
59. Shin J, Gireesh G, Kim SW, Kim DS, Lee S, Kim YS, et al. Phospholipase C beta 4 in the medial septum controls cholinergic theta oscillations and anxiety behaviors. J Neurosci. 29:15375–15385. 2009.
60. Siket MS. Treatment of acute ischemic stroke. Emerg Med Clin North Am. 34:861–882. 2016.
61. Son Y, Kim K, Cho HR. Sildenafil protects neuronal cells from mitochondrial toxicity induced by β-amyloid peptide via ATP-sensitive K+ channels. Biochem Biophys Res Commun. 500:504–510. 2018.
62. Steiner E, Enzmann GU, Lin S, Ghavampour S, Hannocks MJ, Zuber B, et al. Loss of astrocyte polarization upon transient focal brain ischemia as a possible mechanism to counteract early edema formation. Glia. 60:1646–1659. 2012.
63. Stephens S, Kenny RA, Rowan E, Allan L, Kalaria RN, Bradbury M, et al. Neuropsychological characteristics of mild vascular cognitive impairment and dementia after stroke. Int J Geriatr Psychiatry. 19:1053–1057. 2004.
64. Tóthová L, Bábíčková J, Borbélyová V, Filová B, Šebeková K, Hodosy J. Chronic renal insufficiency does not induce behavioral and cognitive alteration in rats. Physiol Behav. 138:133–140. 2015.
65. Teich AF, Sakurai M, Patel M, Holman C, Saeed F, Fiorito J, et al. PDE5 exists in human neurons and is a viable therapeutic target for neurologic disease. J Alzheimers Dis. 52:295–302. 2016.
66. Tzoumas N, Farrah TE, Dhaun N, Webb DJ. Established and emerging therapeutic uses of PDE type 5 inhibitors in cardiovascular disease. Br J Pharmacol. 177:5467–5488. 2020.
67. Viswanathan A, Chabriat H. Cerebral microhemorrhage. Stroke. 37:550–555. 2006.
68. Volke V, Soosaar A, Kõks S, Bourin M, Männistö PT, Vasar E. 7-Nitroindazole, a nitric oxide synthase inhibitor, has anxiolytic-like properties in exploratory models of anxiety. Psychopharmacology (Berl). 131:399–405. 1997.
69. Volke V, Wegener G, Vasar E. Augmentation of the NO-cGMP cascade induces anxiogenic-like effect in mice. J Physiol Pharmacol. 54:653–660. 2003.
70. Wang DD, Bordey A. The astrocyte odyssey. Prog Neurobiol. 86:342–367. 2008.
71. Yaghi S, Willey JZ, Khatri P. Minor ischemic stroke: triaging, disposition, and outcome. Neurol Clin Pract. 6:157–163. 2016.
72. Yen TL, Hsu CK, Lu WJ, Hsieh CY, Hsiao G, Chou DS, et al. Neuroprotective effects of xanthohumol, a prenylated flavonoid from hops (Humulus lupulus), in ischemic stroke of rats. J Agric Food Chem. 60:1937–1944. 2012.
73. Yousuf S, Atif F, Ahmad M, Hoda N, Ishrat T, Khan B, et al. Resveratrol exerts its neuroprotective effect by modulating mitochondrial dysfunctions and associated cell death during cerebral ischemia. Brain Res. 1250:242–253. 2009.
74. Yu YH, Lee K, Sin DS, Park KH, Park DK, Kim DS. Altered functional efficacy of hippocampal interneuron during epileptogenesis following febrile seizures. Brain Res Bull. 131:25–38. 2017.
75. Zhang L, Zhang RL, Wang Y, Zhang C, Zhang ZG, Meng H, et al. Functional recovery in aged and young rats after embolic stroke: treatment with a phosphodiesterase type 5 inhibitor. Stroke. 36:847–852. 2005.
76. Zhang R, Wang Y, Zhang L, Zhang Z, Tsang W, Lu M, et al. Sildenafil (viagra) induces neurogenesis and promotes functional recovery after stroke in rats. Stroke. 33:2675–2680. 2002.
77. Zhu L, Yang JY, Xue X, Dong YX, Liu Y, Miao FR, et al. A novel phosphodiesterase-5 inhibitor: yonkenafil modulates neurogenesis, gliosis to improve cognitive function and ameliorates amyloid burden in an APP/PS1 transgenic mice model. Mech Ageing Dev. 150:34–45. 2015.
Fig. 1.
Sildenafil (SIL) treatment reduced the infarction volume induced by mild ischemic stroke. A : Representative diagram showing the overall surgical groups and histology time schedules. B : Brain slices showing major infarct zones in the brains of MCAo30 rats. The infarct size in the brains of both the MCAo30+SIL-pre and the MCAo30+SIL-post groups were significantly reduced, as compared with MCAo30 rat and to a level similar to the naïve controls. White areas indicate infarct zones in the brain tissue after mild ischemic stroke; red areas indicate intact tissues; a dotted line indicates the core area of infarct zone. C : Quantitative analysis of the infarct size using TTC staining. Data were presented as the mean±standard error of mean. *p<0.01, †p<0.001; one-way analyzed using one-way analysis of variance. MCAo : middle cerebral artery occlusion, Inj. : injection, TTC : triphenyltetrazolium chloride.
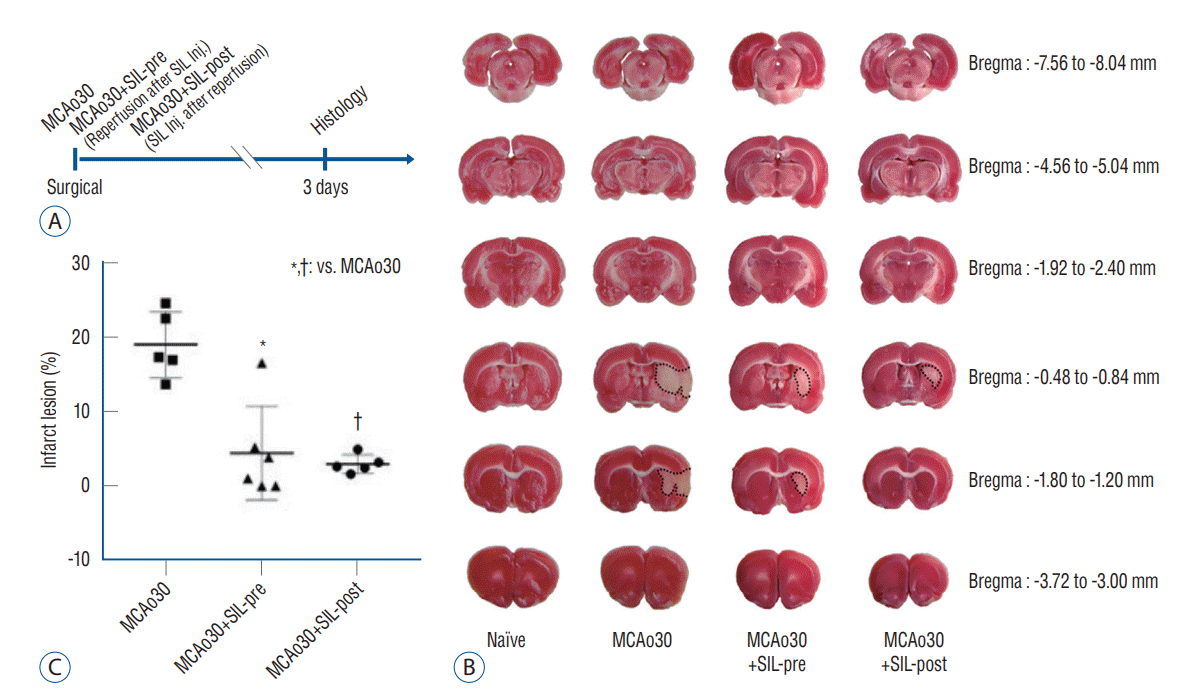
Fig. 2.
Neuroprotective effect of sildenafil (SIL) on the infarction induced by mild ischemic stroke. CV labeling in the ipsilateral side of the striatum and piriform cortex of (A) the naïve controls, (B) the MCAo30, (C) the MCAo30+SIL-pre, and (D) the MCAo30+SIL-post rat models. Rectangles in panels A, B, C and D indicate the high-magnification of panels A1 and A2, B1 and B2, C1 and C2, and D1 and D2, respectively. Arrows in panels C1, C2, D1 and D2 indicate the CV-positive neuronal cell body. Scale bar=280 μm (panels A, B, C, and D) and 50 μm (panels A1, A2, B1, B2, C1, C2, D1, and D2). MCAo : middle cerebral artery occlusion, CV : cresyl violet.
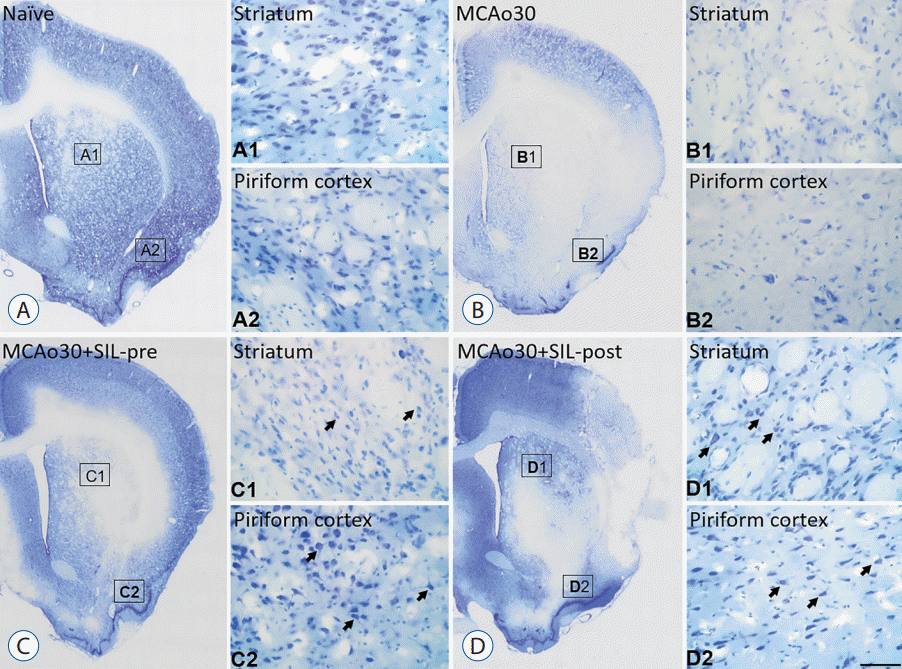
Fig. 3.
Sildenafil (SIL) treatment reduced cerebral edema in the hippocampus induced by mild ischemic stroke. Double labelling of S100B and AQP4 in the hippocampus and the penumbra of (A) the naïve controls, (B) the MCAo30, (C) the MCAo30+SIL-pre, and (D) the MCAo30+SIL-post rat models. S100B (green); AQP4 (red); Merged images (yellow). Scale bar=18.8 μm. Data are presented as the mean a standard error of mean. The averaged number of double-labelled astrocytes and vessels in the CA1 (E) and the penumbra (F). *p<0.05, †p<0.01, ‡p<0.001, compared with the naïve controls; §p<0.01, ||p<0.001, compared with the MCAo30 group; one-way analyzed using one-way analysis of variance. S100B : S100 calcium-binding protein B, AQP4 : aquaporin 4, MCAo : middle cerebral artery occlusion, A.U., arbitrary unit.
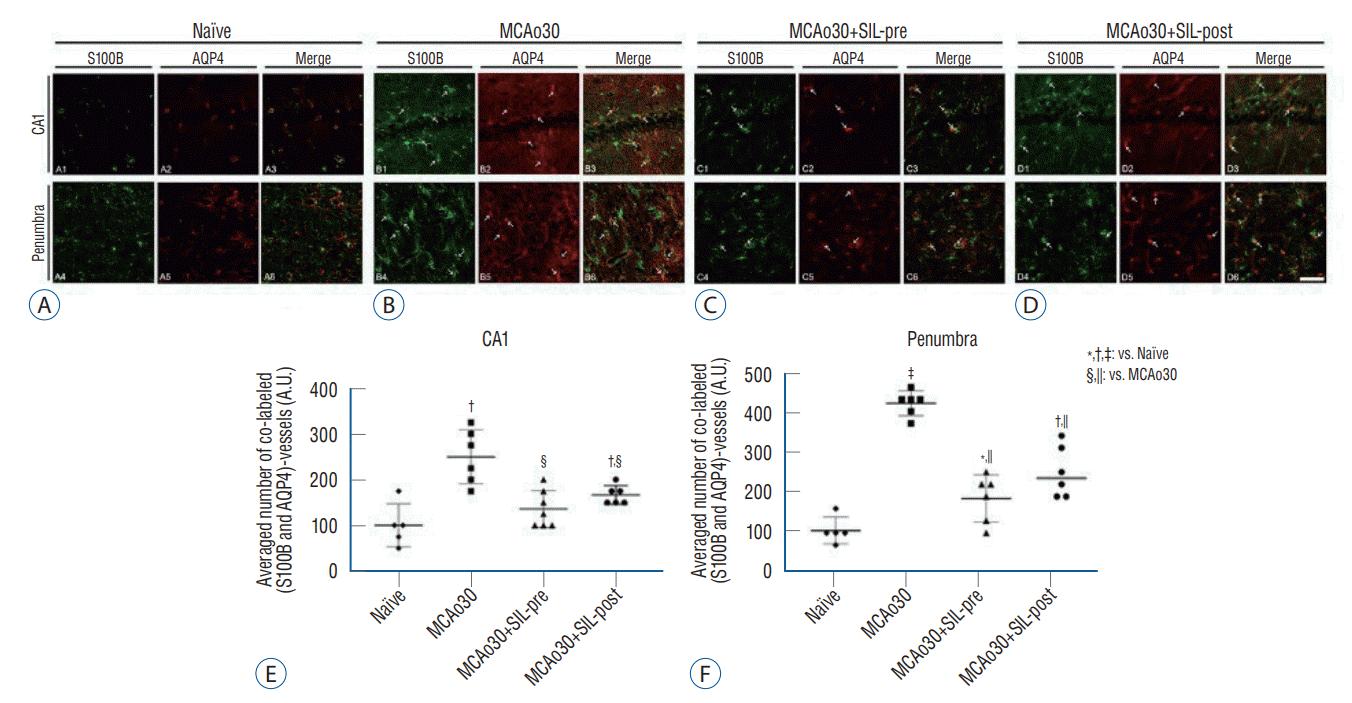
Fig. 4.
Sildenafil (SIL) treatment alleviated anxiogenic behavior induced by mild ischemic stroke. A : Representative diagram showing the overall surgical groups and timeline of experiment. B-D : Elevated plus-maze task. Percentage of entries into the open arms (B) and total number of entries into the open and closed arms of the naïve controls, the MCAo30, the MCAo30+SIL-pre, and the MCAo30+SIL-post groups (C). Scatter plots shows correlation between the MCAo infarct size and anxiogenic behavior about elevated plus-maze (D). E-G : Light/dark transition task. The total time in the light box (E) and number of light/dark transition were validated in the naïve controls, the MCAo30, the MCAo30+SIL-pre, and the MCAo30+SIL-post groups (F). Scatter plots shows correlation between the MCAo infarct size and anxiety about light/dark box (G). Data are presented as the mean a standard error of mean. *p<0.05, †p<0.01, ‡p<0.001, compared with the naïve controls; §p<0.05, ||p<0.01, ¶p<0.001, compared with the MCAo30 group. **p<0.05, a comparison between the MCAo30+SIL-pre and the MCAo30+SIL-post; one-way analyzed using one-way analysis of variance. MCAo : middle cerebral artery occlusion, Inj. : injection, IHC : immunohistochemistry.
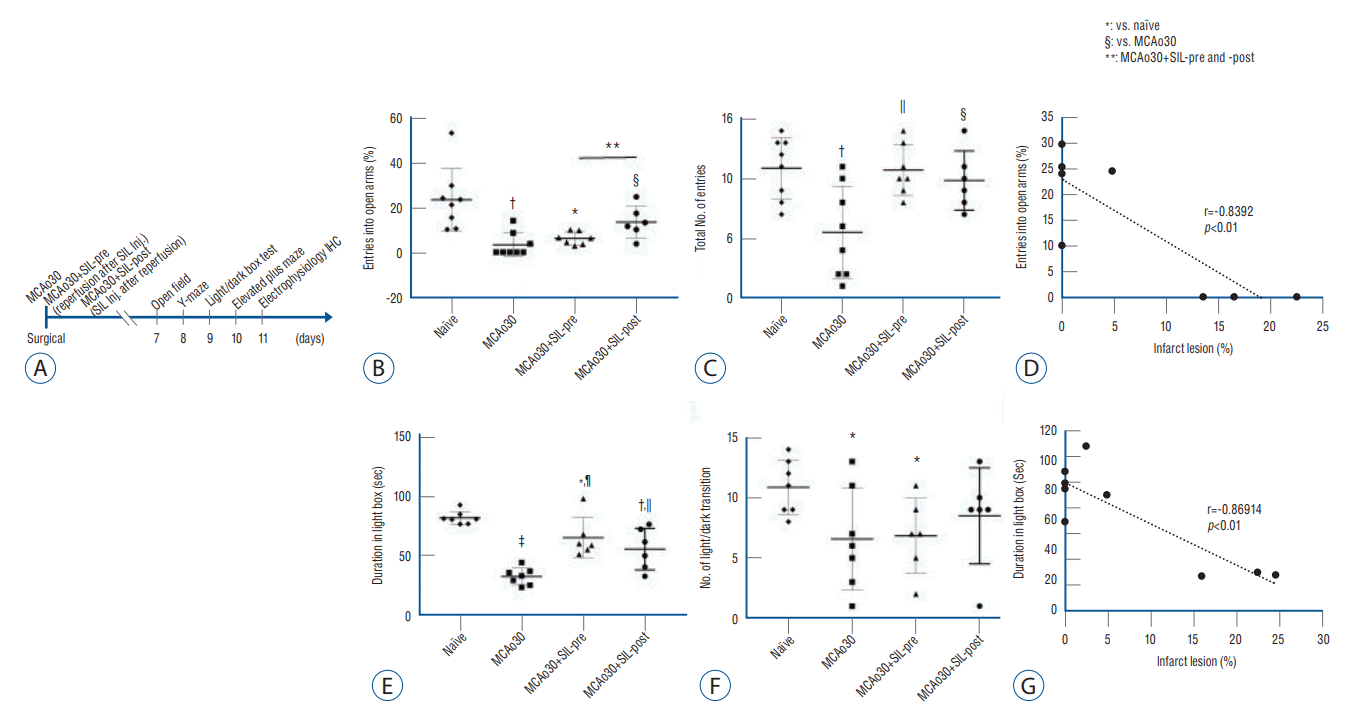
Fig. 5.
Sildenafil (SIL) treatment improved locomotion deficits and directional preference accompanying mild ischemic stroke. A-D : Open-field test. Representative cumulative traces of navigation pathways of naïve controls, the MCAo30, the MCAo30+SIL-pre, and the MCAo30+SIL-post groups during exploratory behavior in the open-field arena (A). Total distance moved in the open-field arena of naïve controls, the MCAo30, the MCAo30+SIL-pre, and the MCAo30+SIL-post groups (B). Circling behavior in the Y-maze arena of naïve controls, the MCAo30, the MCAo30+SIL-pre, and the MCAo30+SIL-post groups (C). Graph shows correlation between the MCAo infarct size and movement disorders (D). E and F : Y-maze test. The schematic diagram showing the MCAo rat model’s preference for left direction, ipsilateral side of MCAo in the left hemisphere of the brain, in the Y-maze (E). The averaged percentage of preference for left direction in the naïve controls, the MCAo30, the MCAo30+SIL-pre, and the MCAo30+SIL-post (F). Data are presented as the mean a SEM. *p<0.001, compared with the naïve controls; †p<0.01, ‡p<0.001, compared with the MCAo30 group; §p<0.01, comparison between the MCAo30+SIL-pre and the MCAo30+SIL-post; one-way analyzed using one-way analysis of variance. ||p<0.01. MCAo : middle cerebral artery occlusion.
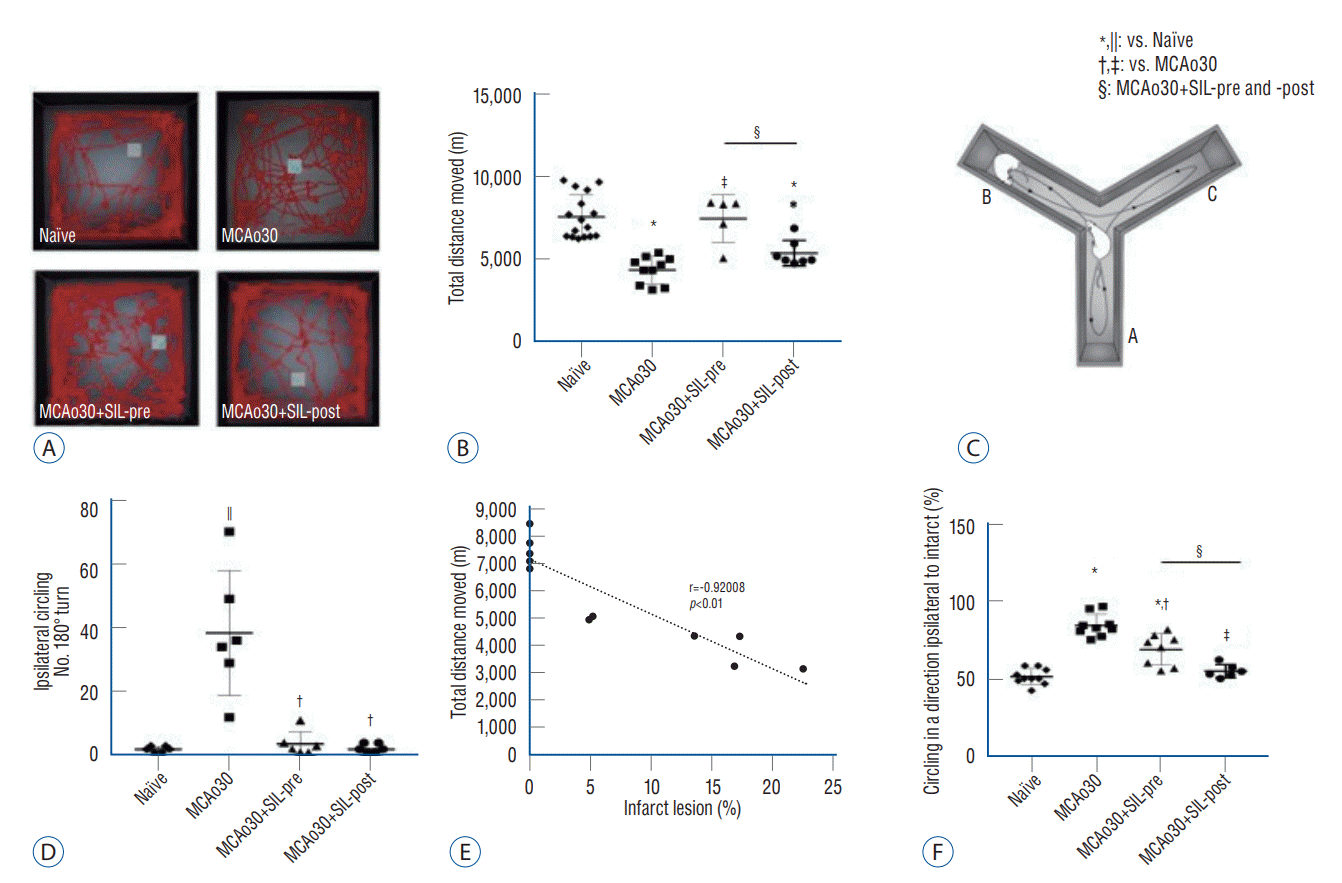
Fig. 6.
Sildenafil (SIL) treatment alleviated the alteration of theta-frequency oscillations induced by mild ischemic stroke. A : Representative raw traces of LFP recordings in the CA1 region of the hippocampus during urethane-induced anesthesia. B : Power spectral analysis of LFP in the CA1 region of naïve controls, the MCAo30, the MCAo30+SIL-pre, and the MCAo30+SIL-post groups. C and D : Power spectral analysis of 4-7 Hz neural oscillations in the hippocampal CA1 region (C) and normalized total power (D) of naïve controls, the MCAo30, the MCAo30+SIL-pre, and the MCAo30+SIL-post rat models. Data are presented as the mean±standard error of mean. *p<0.05, †p<0.001, compared with the naïve controls; ‡p<0.01, §p<0.001, compared with the MCAo30 group; ||p<0.01, comparison between the MCAo30+SIL-pre and the MCAo30+SIL-post; one-way analyzed using one-way analysis of variance. ¶p<0.05. MCAo : middle cerebral artery occlusion.
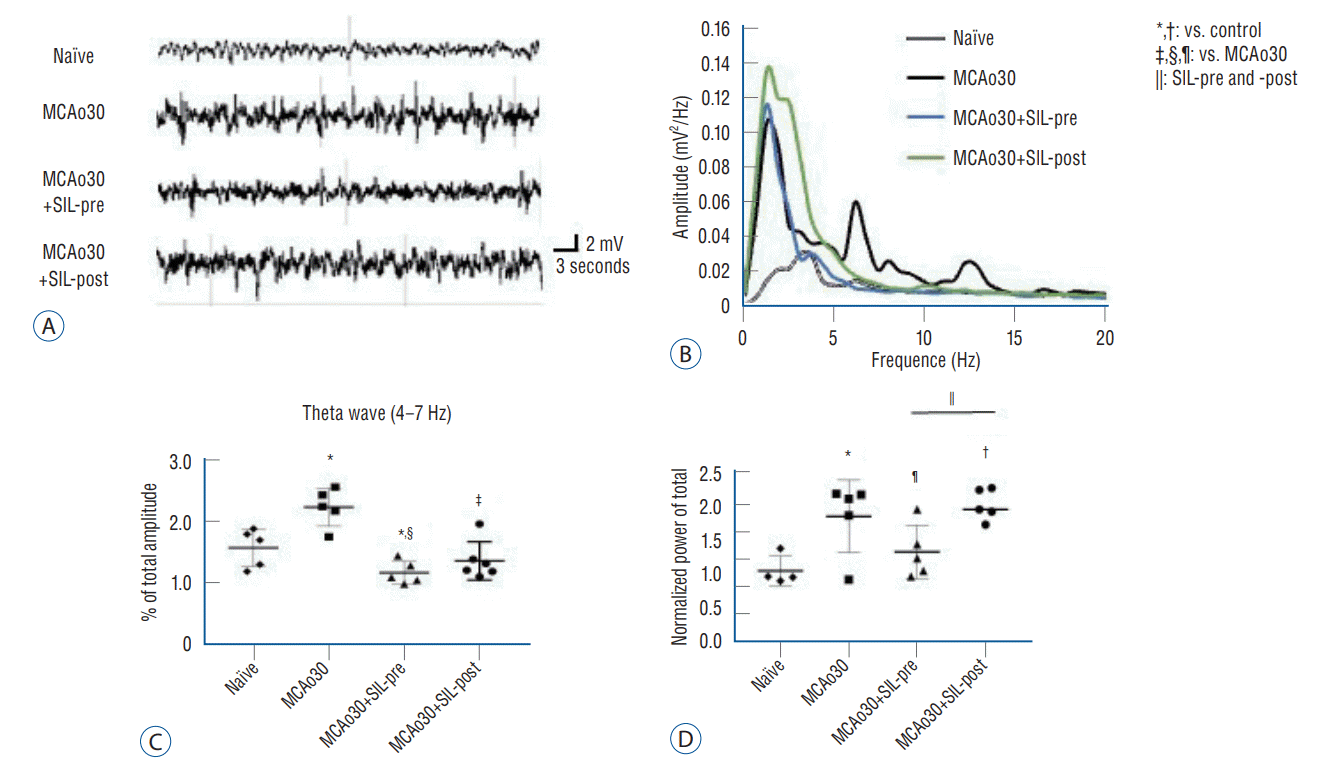