Abstract
Cis-diamminedichloroplatinum(II) (cisplatin), platinum compound, is an anti-cancer agent currently used for the treatment of a number of human solid cancers. It irreversibly reacts with DNA to form an interconnection between guanine-group helices, resulting in cell death. Furthermore, it is also associated with numerous adverse effects such as nephrotoxicity, neurotoxicity, and ototoxicity. Hearing loss due to cisplatin ototoxicity is usually permanent and bilateral. Many studies have been conducted on the ototoxicity of cisplatin, however, its pathology and treatment have not been fully elucidated. Studies have reported that cisplatin inhibits the action of adenylate cyclase in the stria vascularis, and hearing loss occurs due to a decrease in the receptor current of the outer hair cells. In addition, it has been reported that morphological changes in the inner ear include changes in outer hair cells and abnormal findings in the supporting cells of the organ of Corti and Reissner’s membrane. Oxidative stress is known to be the main cause of ototoxicity. In addition, it has recently been suggested that inflammation may trigger inner ear cell death through autophagy, necrosis, and endogenous apoptosis. In this review, we intend to provide a basis for the prevention strategy of cisplatin-induced ototoxicity by revealing its molecular targets and intracellular pathways.
시스플라틴(cisplatin)은 종양세포의 증식을 억제하는 항암제로서 두경부 악성종양 및 여러 고형암(난소암, 고환암, 방광암, 폐암 등)의 치료에 이용되고 있다[1,2]. 이는 DNA에 비가역적으로 반응하여 구아닌기의 나선 가닥들 사이에서의 상호 연결을 통해 세포 파괴를 유발하는 약물로서 항암 효과 외에 가볍게는 구역, 구토 등의 부작용 일으키며, 신독성, 신경독성 및 이독성 등의 영구적인 부작용 또한 유발하는 것으로 알려져 있다[1,2]. 시스플라틴에 의한 부작용은 특히 소아에서 심하며, 투여 받는 소아의 60% 이상이 신기능장애를 경험하는 것으로 알려져 있으며, 약 60% 이상의 소아에서 영구적인 청력저하가 유발된다고 보고되었다[3-5]. 시스플라틴에 의한 난청은 양측성으로 진행하는 비가역적 감각신경성 난청의 형태로, 전 연령대에서의 유병률은 문헌에 따라 다양하여 11%-97% 정도이며, 평균 62%의 발생빈도를 보이는 것으로 알려져 있다[6,7]. 이는 투여 방법, 투여 용량, 체내 누적 용량, 환자의 나이, 다른 이독성 약물의 병용투여 및 두경부 방사선 치료 등과 밀접한 관련이 있으며, 고음역대부터 진행하는 난청 외에도 약 2%-36%에서 이명을 동반하기도 한다[8]. 시스플라틴은 혈관조에서 adenylate cyclase 작용을 억제하여, 유모세포의 수용체 전류(receptor current)를 감소시켜 난청을 유발한다고 알려져 있으며, 외유모세포 및 지지세포 등의 형태학적 이상 또한 유발하는 것으로 보고되었다[9]. 이처럼 시스플라틴 이독성을 와우의 직접적 손상으로 설명하는 것이 일반적이나, 항암제로서의 시스플라틴의 표적은 증식하는 종양 세포의 핵 DNA인데 반해, 증식하지 않는 와우세포가 시스플라틴의 영향을 받는 기전은 아직 명확히 이해되지 않는다. 따라서 현재까지 시스플라틴 이독성의 명확한 발생 부위와 기전에 대해서는 논란이 있으며, 이를 예방할 수 있는 FDA 승인 약물도 없는 상태이다[9].
본 종설에서는 현재까지 알려진 시스플라틴 항암제의 이독성 기전을 정리하여, 이독성으로부터 와우유모세포를 보호하기 위한 연구의 초석으로서 분자 표적의 이해를 돕고자 한다.
시스플라틴은 1844년 이탈리아 화학자인 Michele Peyrone에 의해 처음 합성되었으며, 1966년 미시건 대학원의 생물학자인 Barnett Rosenberg가 진핵세포 분열에 대한 자기장의 영향을 조사하던 중 우연히 자기장에서 철의 배열이 세포분열에서 볼 수 있는 유사분열 방추의 배열과 유사하다는 것을 발견하면서 이에 대한 연구가 시작되었다[10,11]. 그는 후속 연구를 통해 용해된 백금이 암모늄 및 염화물이온과 반응하여 세포 분열을 억제하는 역할을 한다는 것을 발견하였는데, 이 화합물이 시스플라틴이다[12,13]. 이후 백혈병과 육종(sarcoma) 마우스모델 실험을 통해 이 화합물의 항 종양 활성을 확인함으로써, 시스플라틴은 현재 두경부암, 식도암, 폐암, 고환암, 난소암, 자궁경부암 등 다양한 고형 종양의 치료에 널리 이용되고 있다[13-15].
시스플라틴은 다양한 기전으로 암세포를 제거하는 것으로 알려져 있는데, 종양세포 DNA와의 결합 및 부가물 형성(adduct formation)이 종양 시스플라틴의 주요 표적인 것으로 여겨진다. 시스플라틴과 종양세포 DNA의 결합은 전사와 DNA 복제를 억제하고, 시스플라틴-DNA 부가물의 복구가 제대로 이루어지지 않으면, 암세포의 세포자멸사(apoptosis)가 일어나면서 세포가 사멸한다[16-18]. 또한 시스플라틴은 종양세포의 DNA에 결합하여 강력한 산화 스트레스 반응을 생성하며, 괴사(necoptosis) 및 ferroptosis를 통해 추가적인 세포독성 기전으로 세포사멸을 일으키는 것으로 보고된다[19-22].
전신투여된 시스플라틴은 초기에는 와우의 고주파영역에서 외유모세포의 사멸을 일으키지만, 진행되면 저주파영역으로 세포 사멸이 진행된다[8,23,24]. 따라서 지속적인 이독소의 체내 누적량의 증가는 전주파수 영역으로의 영구적인 청력 손실의 위험성 증가를 의미한다[23,25]. 시스플라틴은 주로 유모세포에 직접적인 손상을 미치는 것으로 잘 알려져있으나, 유모세포 외에도 지지세포, 나선신경절 세포에도 영향을 주는 것으로 보고되었다[26-28]. 이독성에 대한 연구의 대부분이 정상 와우를 이용한 연구로 진행되기 때문에, 이독성의 일차 부위에 대한 결정은 쉽지 않다. 일부 연구에서의 복합활동전위(compound action potential, CAP)와 청성뇌간반응(auditory brainstem response, ABR) 역치 증가 결과는 나선 신경절의 손상을 반영하는데, 이는 외유모세포와 동시에 제 1형 나성 신경절의 수초 손실과 관련이 있는 것으로 확인되었다[29]. 또한 ABR 역치 증가 결과는 내림프의 Ca2+ 및 K+ 농도의 감소로 인한 결과일 수 있는데, 이는 시스플라틴에 의한 와우 측벽의 Na+/K+-ATPase 활성 감소와 관련이 있을 수 있다[30]. 현재까지 시스플라틴 이독성에 대한 분자적 이해를 돕기 위한 여러 연구들이 진행되었으나, 아직까지 명확한 기전 확인에는 한계가 있다.
여러 연구에 따르면 시스플라틴은 혈관조(stria vascularis)를 통해 우선적으로 와우로 들어간 후 내림프에서 유모세포로 들어간다고 한다[31-33]. 구리 유사 수송체-1 (copper-like transport-1, CTR1) 및 유기 양이온 수송체-2 (organic cation transporter 2, OCT2)는 혈관조의 세포에서 발현되지만 나선 인대에서는 발현되지 않는 점으로 보아, 이들이 혈액-미로 장벽(blood labyrinth barrier, BLB)을 가로질러 내림프 내로 시스플라틴을 수송하는 잠재적인 수송 메커니즘일 것으로 추정된다[34-36].
일반적으로 약물에 의한 영구적 이독성이 일어나기 위해서는 이독소가 유모세포에 들어가는 과정이 필요한데, 시스플라틴이 유모세포로 들어가는 정확한 기전은 현재까지는 알기 어려운 상태이나, Hall 등의 연구에 따르면 수동적인 확산 과정을 통해서도 배양된 유모 세포로 들어갈 수 있음이 보고되었다[37,38]. 또한 유모세포의 stereocilia 끝부분에는 비선택적 양이온채널인 막투과 채널 유사 단백질(transmembrane channel-like protein-1, TMC1)이 있어 물질의 기계적 개폐에 작용하게 되는데, 제브라피쉬를 이용한 과거 연구에서는 기능적 TMC1 채널을 가진 유모세포의 경우 시스플라틴 이독성에 가장 취약함을 확인함으로써, 시스플라틴을 유모세포로 수송되는 과정에 TMC1 채널이 관여할 것으로 추측된다[39-41]. 혈관조세포에서 발현되는 CTR1, OCT2 수송체는 와우 유모세포에서도 발현을 확인함으로써, 이들이 시스플라틴의 유모세포로의 흡수도 돕는 것으로 추정된다[40,41]. OCT 차단제인 시메티딘을 전신투여하거나 OCT의 유전적 탈락을 유발하는 황산구리를 국소 투여한 연구에서 시스플라틴 이독성의 완화 효과를 확인하여 OCT가 시스플라틴의 유모세포로의 수송에 관여함을 뒷받침하는 근거로 제시되었으나, 제브라피쉬 연구 등에서는 이러한 수송체의 발현이 발견되지 않아, 현재까지는 TMC1 채널이 주된 수송 역할을 하는 것으로 생각된다[34,35]. 흡수된 시스플라틴은 염화물 중 하나 또는 둘 모두가 물 분자에 의해 대체되어 낮은 막투과성을 갖는 아쿠아복합체를 생성하는 수화 과정을 거친다[42]. 또한 유모세포로 들어간 시스플라틴은 최소 12-18개월 동안 유지되는 것으로 알려져 있다(Fig. 1) [31].
시스플라틴 이독성은 염증반응의 자극과 관련이 있는데, 세포외 신호조절 키나아제(extracellular signal-regulated kinases, ERK)를 활성화 함으로써, 종양괴사인자(tumor necrosis factor α, TNF-α), 인터루킨-1b (interleukin 1b, IL-1b), IL-6의 방출을 유도한다[47-49]. 코르티기관에서 파생된 마우스 세포 라인인 HEI-OC1 연구에서는 시스플라틴 처리 1시간 후 기존 세포에서 염증 사이토카인이 분비되고, 이후에는 새로운 단백 합성을 통해 염증 사이토카인이 분비됨을 확인하였다[47]. 이러한 결과는 염증이 시스플라틴 이독성의 첫번째 과정일 것이라는 가설을 뒷받침한다. 또한 4일간 4 mg/kg의 시스플라틴을 복강 주사한 마우스에서는 TNF-α, IL-1b 및 IL-6의 혈청 수준이 3-7배 증가했으며, 와우에서 동일한 사이토카인의 mRNA 및 단백 수치가 증가함을 확인하였다[47]. 특히, 시스플라틴 유도 TNF-α 단백 수치는 코르티기관의 혈관조, 나선인대(spiral ligament)와 나선윤부(spiral limbus), spiral modiolar veins and lacunae과 유모세포에서 증가됨을 확인하였으며, IL-1b는 주로 나선 인대와 spiral modiolar veins 및 lacunae에서 발견된 반면, IL-6는 spiral modiolar veins 및 lacunae에서만 발견되었다[47]. 항산화제 전처리를 한 HEI-OC1 세포에서는 사이토카인 분비가 영향을 받지 않았는데, 이는 시스플라틴 유도 염증반응이 활성산소종(reactive oxygen species, ROS)의 발생보다 선행하는 반응임을 시사한다[47]. 또한, 시스플라틴 투여 전 TNF-α를 중화하게 되면 HEI-OC1 세포의 생존력이 향상되었고, UB-OC1 세포에서는 세포자멸사가 감소함을 확인하였으며. TNF-α, IL-1b, IL-6를 동시에 중화하는 경우에는 HEI-OC1 세포에서 ROS 생산이 감소하였다[47]. 이는 내이세포에서 염증성 사이토카인, 세포자멸사, ROS 생성 사이에 밀접한 관련이 있음을 시사한다. HEI-OC1 세포를 통한 또다른 연구에서 시스플라틴은 nuclear factor kappa-light-chain-enhancer of activated B cells (NF-kB)의 전위 증가, TNF-α, IL-6의 증가를 통해 염증반응을 증폭시키는 것으로 확인되었다[50].
전염증성 사이토카인(proinflammatory cytokine)의 합성은 신호변환기와 전사 활성인자(signal transducer and activator of transcription 1, STAT1)의 활성에 의해 촉진되는 것으로 알려져 있는데, STAT6 (STAT6-/-)가 비활성화된 마우스의 와우세포에서 시스플라틴은 TNF-α, IL-1b 및 IL-6의 혈청수준 및 mRNA 발현, NK-kB mRNA를 증가시키지 않았으며, 세포자멸사 또한 유도하지 않아, 시스플라틴이 매개하는 전염증반응에서 STAT6가 관여함을 확인하였다[50,51]. UB-OC1 세포 연구에서 활성형 STAT1은 염증 단백인 cyclooxygenase 2 (COX2), TNF-α, 유도성 산화질소 합성 효소(inducible nitric oxide synthase, iNOS), 면역세포침윤의 마커로 알려진 CD14 및 CD45의 발현을 통해 염증반응을 촉진하는 것으로 보고되며, 시스플라틴을 주입한 쥐의 혈관조, 나선인대, 나선윤부, 나선신경절 및 외유모세포에서 STAT1에 의해 동일한 염증 단백과 면역 마커의 상승이 확인되었다[52]. 이러한 결과는 시스플라틴이 와우세포에서 사이토카인 방출에 직접 영향을 미치고 STAT 단백과 NF-kB 활성을 강화하여 사이토카인 생성을 자극함으로써 염증을 유도한다는 가설을 뒷받침한다[52].
와우는 대사 활동이 활발한 기관으로서, 와우의 항산화 시스템에는 글루타치온 퍼옥시다제, 글루타티온 환원효소, 글루타티온-s-트랜스퍼라제, 슈퍼옥사이드 디스뮤타제, 카탈라제 및 감마-글루타밀 시스테인 합성효소와 같은 효소가 포함되며, 균형적인 항산화 시스템은 와우의 정상적인 생리와 청력 유지에 필수적인 역할을 한다. 와우는 저산소증과 허혈-재관류에 매우 민감한 기관으로 과도한 소음과 이독성 물질은 와우의 산화스트레스를 증가시키는 것으로 알려져 있다[29]. 시스플라틴 이독성은 와우의 특이적 활성산호 증가 효소인 NADPH oxidase 3 (NOX3), 크산틴 산화효소(xanthin oxidase) 및 내인성 항산화 효소 시스템의 감소를 통해 ROS를 증가시키는 것으로 보고된다[7,53-56]. 와우세포에서 시스플라틴의 흡수는 전사인자, 신호변화인자 및 STAT1을 자극하여 TRPV1과 NOX3 경로를 활성화하여 활성산소를 독성 수준까지 발생시켜 세포 사멸을 유발하며, 이는 유모세포뿐만 아니라 유모세포 항상성 유지에 중요한 비감각 와우세포 및 내림프 전위를 유발하는 혈관조의 중간세포(intermediate cell) 및 변연세포(marginal cell)도 포함된다[57-59].
ROS 생성은 다양한 항산화제 효과에 대한 연구를 통해 시스플라틴 유발 난청의 기본 기전으로 제안된다[60,61]. 와우세포에서 ROS 생성을 유발하는 주요 과정은 NADPH 산화효소 소단위의 상향조절로 확인되었으며[62-64], 강화된 NADPH 산화효소 활성을 통해 생성된 ROS는 NADPH 산화효소 소단위의 합성을 다시 자극하여 자가 촉매 루프에서 ROS 생산을 더욱 증가시킨다[64]. HEI-OC1 세포연구에서 6시간 동안 20 mM 시스플라틴에 노출된 TNF-α, IL-1b 및 IL-6는 NADPH 산화효소 활성을 20%-50% 감소시켰으며, 또다른 ROS 생성 효소인 xanthine oxidase의 활성을 증가시킴으로써 ROS 생산을 향상시키는 것으로 보고되었다[64,65]. 최근 연구에서는 18시간 동안 50 mM의 시스플라틴으로 처리한 HEIOC1 세포에서 보조인자 사이클린 A (cofactor cyclin A)의 상향조절을 통해 사이클린 의존성 키나제 2 (cyclin-dependent kinase 2, CDK2)활성이 2배 증가하는 것이 밝혀졌으며, CDK2 억제제로 처리한 마우스 explant와 CDK2-knockout cochlear ex- plant에서 시스플라틴 유도 ROS 생성 및 세포 자멸사가 유의하게 감소함을 확인하였다[66]. 그러나 아직까지 시스플라틴이 사이클린 A 및 CDK2 활성을 증가시키는 기전은 명확히 밝혀져 있지 않다.
시스플라틴에 의해 증가된 ROS는 extracellular signalregulated kinase 1 (ERK1)/STAT1 경로를 활성화하여 염증과 세포자멸사에 관여하는 단백인 COX2, TNF-α, iNOS, p53, caspase 3 및 B-cell lymphoma 2 (Bcl-2)-like protein 4 (BAX)의 더 강한 발현을 유발한다[67]. 또한 ROS는 DNA 손상을 일으켜 DNA 복구단백질 과활성화를 유발하는 것으로 알려져 있는데[68], 16 mg/kg의 시스플라틴을 주입한 마우스의 와우에서 4일 후 폴리 ADP-리보스 트랜스퍼라제 1(poly-ADP-ribose transferase 1, PARP-1) 활성이 100% 증가함을 확인하였다[69]. PARP-1은 DNA 복구단백질의 이동에 관여하는 효소이며, 이의 억제는 시스플라틴에 의한 세포 사멸 감소와 관련이 있는 것을 의미한다[69]. 또한, 20 mM 농도의 시스플라틴으로 처리한 HEI-OC1 세포에서는 NAD+/NADH 비율이 시간에 따라 감소(12시간 후 대조군 대비 -30% 및 24시간 후 -55%)하는 것이 확인되어, 시스플라틴 노출 청각세포가 DNA 복구 메커니즘을 활성화하지만 DNA 손상을 예방할 수 없음을 의미한다[69].
염증, ROS 생성, ER 스트레스, DNA 손상은 세포자멸사 경로를 활성화하는 데 관여한다. 시스플라틴을 처리한 explant에서는 외인성 세포자멸사 경로에 관여하는 Fas-associated protein with death domain (FADD)은 발현하지 않는 것으로 보고되었으나[73], 동일한 경로의 구성요소 중 하나인 caspase 8은 발현되는 모순점이 있어, 시스플라틴과 외인성 세포자멸사에 대한 기전은 추가 연구가 필요한 상태이다[74]. 외인성 세포자멸사와는 대조적으로 시스플라틴은 내인성 세포자멸사와 관련된 proapoptotic transcription factor forkhead box O3 (FOXO3a) 및 BAX 단백질의 발현 수준을 증가시키고 BAX 단백질의 미토콘드리아 막으로의 전위를 촉진함으로써, 내인성 세포자멸사 경로를 활성화한다는 점은 여러 연구를 통해 확립된 사실이다[73-75]. 또한 이는 Bcl-2 단백질의 감소, 미토콘드리아 외막의 투과, 사이토크롬 c의 방출, 세포자멸사-유도 인자(apoptosis-inducing factor, AIF)의 더 높은 발현을 유발하여, 이들의 방출 및 핵전위를 촉진한다[74-77]. 이는 또한 세포사멸 프로테아제 활성화인자 1 (apoptotic protease activating factor 1, APAF-1)의 mRNA 및 단백을 증가시키고, caspase 9 및 3의 발현 및 활성을 증가시키며[73,76,77], NF-kB 핵으로의 전위를 촉진하고, 전사인자의 세포자멸사 역할을 돕는다[77].
다양한 청각세포 모델연구에서 시스플라틴은 활성형 caspase 1을 증가시킴을 확인하였고, 이 효소는 염증성 사이토카인 IL-1b 및 IL-18의 성숙 및 분비를 촉진하는 복합체인 인플라마솜을 통해 세포사멸을 유도하는 파이롭토시스(pyroptosis) 에 관여하는 것으로 알려져, 시스플라틴에 의한 세포사멸이 pyroptosis와도 관련됨을 확인하였다[78,79].
또한 시스플라틴은 자가포식(autophagy)의 마커로 잘 알려진 Beclin-1, 미세소관 관련 단백질 체인(microtubule-associated protein light chain 3, LC3B-II), 뉴클레오티드 결합 올리고머 도메인, 류신 함유 반복체 X1 (leucine-rich repeat-containing X1, NLRX1)의 발현을 증가시켜, 세포질에 자가포식소체를 축적하여 세포자멸사와는 독립적으로 자가포식세포를 사멸시키는 것으로 보인다[80-82].
최근 연구에 따르면 괴사(necroptosis: a caspase-independent pathway of programmed necrosis)도 시스플라틴 이독성과 관련될 수 있음이 알려졌는데, 16 mg/kg 시스플라틴을 복강 내 주입한 쥐의 와우에서 수용체-상호작용 세린/트레오닌-단백질 키나제1 (receptor-interacting serine/threonine-protein kinase 1, RIPK1)의 발현이 100% 증가됨을 확인하였다[83]. RIPK1은 RIPK3과 함께 ripoptosome을 형성하여 괴사를 유발하는 물질로[84], 면역조직화학 분석 연구에서는 나선신경절, 내유모세포와 외우모세포의 뉴런에서 RIPK3 단백 발현이 증가됨을 확인하였고[83], 또다른 연구에서는 RIPK1을 약리학적으로 억제하여 시스플라틴 관련 청력 상실로부터 마우스를 보호하여 ABR 역치를 30 dB 향상시키는 것을 확인하였다[85]. RIPK3-knock out 마우스와 시스플라틴으로 처리된 Ripk3/caspase 8 double knockout 마우스는 유모세포 생존과 청력 역치(10 dB)에서 상당한 개선을 보이기도 하였다[85]. 일부에서는 시스플라틴이 caspase 8에 의해 매개되는 세포자멸사보다 와우세포의 괴사 촉진이 이독성에 더 기여할 것이라고 주장하기도 하나, 시스플라틴 이독성에서의 괴사 기전의 역할은 추가적인 연구가 필요한 상태이다.
시스플라틴 이독성을 향상시키는 위험요소에는 신부전 및 탈수, 영양 실조 등이 있으며, 혈액-뇌 장벽(blood-brain barrier) 을 파괴하는 프로토콜의 사용은 혈액-미로 장벽(BLB) 또한 파괴함으로써 이독성의 위험을 높이는 것으로 보고된다[86,87]. 지질다당류(lipopolysaccharide, LPS)로 유도된 패혈증 동물모델에서 이독성의 악화가 보고된 바 있는데[88], LPS는 일반적으로 toll-like receptor 4 (TLR4)에 결합하여 내인성 손상 관련 분자패턴을 인식하고 염증반응을 유도하는 물질로서, 염증과정에서 이독성의 악화를 일으킨 것으로 알려져 있다[89]. 또한, 위장관 방사선치료를 함께 하는 경우 방사선 조사는 공생박테리아를 박멸하고, LPS의 혈중농도를 높임으로써 LPS 유도 염증반응이 발생하기도 해, 두경부 방사선 병용 치료 외에도 이독성의 위험을 증가시킬 수 있을 것으로 생각된다[90].
특정 유전적 소인 또한 개인의 이독성 감수성에 영향을 주는 것으로 알려져 있는데, ERCC2와 XPC처럼 DNA 복구 효소에 관한 유전자의 변이 또는 항산화 효소나, 약물 유출 또는 세포막 펌프와 관련된 ABCC3, ACYP2 , COMT, TPMT의 변이가 이에 포함된다(Table 1) [91,92]. 그러나 최근에는 이러한 유전적 소인을 통한 이독성 난청 발생 예측비율이 상대적으로 높지 않으며, 오히려 잘 알려져 있는 이독성 약제인 아미노글리코사이드와 이뇨제 등의 약제 투여나 두경부 방사선 조사를 동반한 경우 소음 노출 등에서 이독성 난청 비율이 높음이 알려져 있다[59,93,94].
시스플라틴 이독성을 예방하기 위해서는 고주파 청력검사 및 distortion product otoacoustic emission (DPOAE) 등의 정기적인 검사를 통한 난청의 조기 발견이 도움이 되며, 시스플라틴 이독성을 감소시키는 방법으로 고장액 식염수의 전처치가 필요한 경우 독성이 적인 2세대 약물인 carboplatin으로의 대체 투여를 하기도 한다. 시스플라틴 항암제의 이독성 기전에 대한 연구들이 진행되면서, 세포 사멸의 억제, 활성화 산호의 중화, 염증 반응의 억제 등 잠재적 보호 가능성을 지닌 약물의 시도와 연구가 있었다. 대부분의 전임상 및 임상시험에서의 이독성 보호 약물은 항산화제 및 항염증제 범주에 속하며, 이에 관련한 경로 중 하나 또는 둘 모두를 억제하는 기전으로 작용한다. 시스플라틴을 주입한 여러 동물 모델에서 항산화제인 apocynin, lactage, alpha lipoic acids 등, 항염증 작용을 동시에 하는 flunarizine, hydrogen, N-acetylcystein 및 세포자멸사에 영향을 주는 pifithrin-a, minocycline 등의 처리시 청력 역치의 상승 및 유모세포 손상의 감소를 확인하였다(Table 2). 임상에서는 thiamin, cyanin 및 실험적으로 sodium thiosulfate, lazaroid 등이 사용되고 있으나, 현재까지 FDA 승인을 받은 약물은 없는 상태이다.
시스플라틴은 암환자의 생명을 구하는 대표적인 항암제로서의 역할을 하지만 비가역적 감각신경성 난청을 유발하는 이독성 또한 내포하는 약제이다. 염증은 시스플라틴 노출 후 처음으로 촉발되는 반응이며, 산화스트레스와 ROS 생성으로 이어지는 일련의 반응을 유발한다. ROS는 지질의 과산화, 단백질 질화, DNA 변경 및 염증 과정의 증폭을 통해 세포 손상을 일으킬 수 있으며, 와우세포는 단백질 및 DNA 복구 메커니즘을 활성화하여, 시스플라틴의 독성으로부터 항상성을 회복하기 위한 노력을 한다. 그러나, ROS로 인해 유도된 세포의 손상에 대한 방어 시스템의 실패는 결국 세포 사멸로 이어지게 되며, 내인성 세포자멸사, pyroptosis, 자가포식 및 necroptosis 또한 이에 관여할 것으로 생각된다.
현재까지 시스플라틴 이독성에 대한 다양한 연구가 진행되었으나, 연구 결과의 논란이 있거나 불완전한 결과를 보고하였고, 이독성에 대한 보편적인 청력 평가 및 이독성 등급에 대한 표준화된 지침이 없는 상태로, 임상 연구의 결과의 해석에 있어서도 논란이 있는 상태이다.
따라서 향후 연구는 시스플라틴의 치료 효능을 방해하지 않으면서, 다양한 활성 경로를 억제하는 복합 약물 사용 및 효과적인 국소 요법의 개발을 고려하는 것이 필요할 수 있다. 시스플라틴 이독성 기전에 대한 더 깊은 이해는 향후 연구자들이 시스플라틴 약물 작용 기전을 비활성화하는 표적 연구를 하는 데 도움이 될 수 있을 것이다.
ACKNOWLEDGMENTS
This research was supported by Basic Science Research Program through the National Research Foundation of Korea (NRF) funded by the Ministry of Education (2021R1F1A1052136).
REFERENCES
1. Fleischman RW, Stadnicki SW, Ethier MF, Schaeppi U. Ototoxicity of cis-dichlorodiammine platinum (II) in the guinea pig. Toxicol Appl Pharmacol. 1975; 33(2):320–32.
2. Kohn S, Fradis M, Pratt H, Zidan J, Podoshin L, Robinson E, et al. Cisplatin ototoxicity in guinea pigs with special reference to toxic effects in the stria vascularis. Laryngoscope. 1988; 98(8 Pt 1):865–71.
3. Skinner R, Pearson AD, English MW, Price L, Wyllie RA, Coulthard MG, et al. Cisplatin dose rate as a risk factor for nephrotoxicity in children. Br J Cancer. 1998; 77(10):1677–82.
4. Knight KR, Kraemer DF, Neuwelt EA. Ototoxicity in children receiving platinum chemotherapy: Underestimating a commonly occurring toxicity that may inf luence academic and social development. J Clin Oncol. 2005; 23(34):8588–96.
5. Knight KR, Kraemer DF, Winter C, Neuwelt EA. Early changes in auditory function as a result of platinum chemotherapy: Use of extended high-frequency audiometry and evoked distortion product otoacoustic emissions. J Clin Oncol. 2007; 25(10):1190–5.
6. Chirtes F, Albu S. Prevention and restoration of hearing loss associated with the use of cisplatin. Biomed Res Int. 2014; 2014:925485.
7. Rybak LP. Mechanisms of cisplatin ototoxicity and progress in otoprotection. Curr Opin Otolaryngol Head Neck Surg. 2007; 15(5):364–9.
8. Schweitzer VG. Ototoxicity of chemotherapeutic agents. Otolaryngol Clin North Am. 1993; 26(5):759–89.
9. Barron SE, Daigneault EA. Effect of cisplatin on hair cell morphology and lateral wall Na,K-ATPase activity. Hear Res. 1987; 26(2):131–7.
10. Alderden RA, Hall MD, Hambley TW. The discovery and development of cisplatin. J Chem Educ. 2006; 83(5):728.
11. Muggia FM, Bonetti A, Hoeschele JD, Rozencweig M, Howell SB. Platinum antitumor complexes: 50 years since Barnett Rosenberg’s discovery. J Clin Oncol. 2015; 33(35):4219–26.
12. Rosenberg B, Van Camp L, Grimley EB, Thomson AJ. The inhibition of growth or cell division in Escherichia coli by different ionic species of platinum(IV) complexes. J Biol Chem. 1967; 242(6):1347–52.
13. Rosenberg B, VanCamp L, Trosko JE, Mansour VH. Platinum compounds: A new class of potent antitumour agents. Nature. 1969; 222(5191):385–6.
14. Barnard C. Platinum anti-cancer agents. Platinum Metals Rev. 1989; 33(4):162–7.
15. Lebwohl D, Canetta R. Clinical development of platinum complexes in cancer therapy: An historical perspective and an update. Eur J Cancer. 1998; 34(10):1522–34.
16. Sorenson CM, Eastman A. Mechanismofcis-diamminedichloroplatinum(II)-induced cytotoxicity: Role of G2 arrest and DNA double-strand breaks. Cancer Res. 1988; 48(16):4484–8.
17. Cepeda V, Fuertes MA, Castilla J, Alonso C, Quevedo C, Pérez JM. Biochemical mechanisms of cisplatin cytotoxicity. Anticancer Agents Med Chem. 2007; 7(1):3–18.
18. Gonzalez VM, Fuertes MA, Alonso C, Perez JM. Is cisplatininduced cell death always produced by apoptosis? Mol Pharmacol. 2001; 59(4):657–63.
19. Xu Y, Ma HB, Fang YL, Zhang ZR, Shao J, Hong M, et al. Cisplatin-induced necroptosis in TNFα dependent and independent pathways. Cell Signal. 2017; 31:112–23.
20. Yu W, Chen Y, Dubrulle J, Stossi F, Putluri V, Sreekumar A, et al. Cisplatin generates oxidative stress which is accompanied by rapid shifts in central carbon metabolism. Sci Rep. 2018; 8(1):4306.
21. Dixon SJ, Lemberg KM, Lamprecht MR, Skouta R, Zaitsev EM, Gleason CE, et al. Ferroptosis: An iron-dependent form of nonapoptotic cell death. Cell. 2012; 149(5):1060–72.
22. Vandenabeele P, Galluzzi L, Vanden Berghe T, Kroemer G. Molecular mechanisms of necroptosis: An ordered cellular explosion. Nat Rev Mol Cell Biol. 2010; 11(10):700–14.
23. Brock PR, Knight KR, Freyer DR, Campbell KC, Steyger PS, Blakley BW, et al. Platinum-induced ototoxicity in children: A consensus review on mechanisms, predisposition, and protection, including a new International Society of Pediatric Oncology Boston ototoxicity scale. J Clin Oncol. 2012; 30(19):2408–17.
24. Garinis AC, Cross CP, Srikanth P, Carroll K, Feeney MP, Keefe DH, et al. The cumulative effects of intravenous antibiotic treatments on hearing in patients with cystic fibrosis. J Cyst Fibros. 2017; 16(3):401–9.
25. Garinis AC, Liao S, Cross CP, Galati J, Middaugh JL, Mace JC, et al. Effect of gentamicin and levels of ambient sound on hearing screening outcomes in the neonatal intensive care unit: A pilot study. Int J Pediatr Otorhinolaryngol. 2017; 97:42–50.
26. Ramírez-Camacho R, García-Berrocal JR, Buján J, Martín-Marero A, Trinidad A. Supporting cells as a target of cisplatin-induced inner ear damage: Therapeutic implications. Laryngoscope. 2004; 114(3):533–7.
27. Jadali A, Ying YM, Kwan KY. Activation of CHK1 in supporting cells indirectly promotes hair cell survival. Front Cell Neurosci. 2017; 11:137.
28. Wu X, Li X, Song Y, Li H, Bai X, Liu W, et al. Allicin protects auditory hair cells and spiral ganglion neurons from cisplatin -Induced apoptosis. Neuropharmacology. 2017; 116:429–40.
29. Schacht J, Talaska AE, Rybak LP. Cisplatin and aminoglycoside antibiotics: Hearing loss and its prevention. Anat Rec (Hoboken). 2012; 295(11):1837–50.
30. Cheng PW, Liu SH, Hsu CJ, Lin-Shiau SY. Correlation of increased activities of Na+, K+-ATPase and Ca2+-ATPase with the reversal of cisplatin ototoxicity induced by D-methionine in guinea pigs. Hear Res. 2005; 205(1-2):102–9.
31. Breglio AM, Rusheen AE, Shide ED, Fernandez KA, Spielbauer KK, McLachlin KM, et al. Cisplatin is retained in the cochlea indefinitely following chemotherapy. Nat Commun. 2017; 8(1):1654.
32. Chu YH, Sibrian-Vazquez M, Escobedo JO, Phillips AR, Dickey DT, Wang Q, et al. Systemic delivery and biodistribution of cisplatin in vivo. Mol Pharm. 2016; 13(8):2677–82.
33. van Ruijven MW, de Groot JC, Hendriksen F, Smoorenburg GF. Immunohistochemical detection of platinated DNA in the cochlea of cisplatin-treated guinea pigs. Hear Res. 2005; 203(1-2):112–21.
34. Ciarimboli G, Deuster D, Knief A, Sperling M, Holtkamp M, Edemir B, et al. Organic cation transporter 2 mediates cisplatininduced oto- and nephrotoxicity and is a target for protective interventions. Am J Pathol. 2010; 176(3):1169–80.
35. More SS, Akil O, Ianculescu AG, Geier EG, Lustig LR, Giacomini KM. Role of the copper transporter, CTR1, in platinum-induced ototoxicity. J Neurosci. 2010; 30(28):9500–9.
36. Waissbluth S, Daniel SJ. Cisplatin-induced ototoxicity: Transporters playing a role in cisplatin toxicity. Hear Res. 2013; 299:37–45.
37. Hiel H, Erre JP, Aurousseau C, Bouali R, Dulon D, Aran JM. Gentamicin uptake by cochlear hair cells precedes hearing impairment during chronic treatment. Audiology. 1993; 32(1):78–87.
38. Hall MD, Okabe M, Shen DW, Liang XJ, Gottesman MM. The role of cellular accumulation in determining sensitivity to platinumbased chemotherapy. Annu Rev Pharmacol Toxicol. 2008; 48:495–535.
39. Alharazneh A, Luk L, Huth M, Monfared A, Steyger PS, Cheng AG, et al. Functional hair cell mechanotransducer channels are required for aminoglycoside ototoxicity. PLoS One. 2011; 6(7):e22347.
40. Pan B, Akyuz N, Liu XP, Asai Y, Nist-Lund C, Kurima K, et al. TMC1 forms the pore of mechanosensory transduction channels in vertebrate inner ear hair cells. Neuron. 2018; 99(4):736–53. e6.
41. Thomas AJ, Hailey DW, Stawicki TM, Wu P, Coffin AB, Rubel EW, et al. Functional mechanotransduction is required for cisplatin-induced hair cell death in the zebrafish lateral line. J Neurosci. 2013; 33(10):4405–14.
42. Eljack ND, Ma HY, Drucker J, Shen C, Hambley TW, New EJ, et al. Mechanisms of cell uptake and toxicity of the anticancer drug cisplatin. Metallomics. 2014; 6(11):2126–33.
43. Jiang M, Karasawa T, Steyger PS. Aminoglycoside-induced cochleotoxicity: A review. Front Cell Neurosci. 2017; 11:308.
44. Karasawa T, Steyger PS. An integrated view of cisplatin-induced nephrotoxicity and ototoxicity. Toxicol Lett. 2015; 237(3):219–27.
45. Eastman A. The mechanism of action of cisplatin: from adducts to apoptosis. In : Lippert B, editor. Cisplatin: chemistry and biochemistry of a leading anticancer drug. Zürich: Verlag Helvetica Chimica Acta;1999. p. 111–34.
46. Karasawa T, Sibrian-Vazquez M, Strongin RM, Steyger PS. Identification of cisplatin-binding proteins using agarose conjugates of platinum compounds. PLoS One. 2013; 8(6):e66220.
47. So H, Kim H, Lee JH, Park C, Kim Y, Kim E, et al. Cisplatin cytotoxicity of auditory cells requires secretions of proinflammatory cytokines via activation of ERK and NF-kappaB. J Assoc Res Otolaryngol. 2007; 8(3):338–55.
48. Kim SJ, Kwak HJ, Kim DS, Choi HM, Sim JE, Kim SH, et al. Protective mechanism of Korean Red Ginseng in cisplatin-induced ototoxicity through attenuation of nuclear factor-κB and caspase-1 activation. Mol Med Rep. 2015; 12(1):315–22.
49. Levano S, Bodmer D. Loss of STAT1 protects hair cells from ototoxicity through modulation of STAT3, c-Jun, Akt, and autophagy factors. Cell Death Dis. 2015; 6(12):e2019.
50. Kim HJ, Oh GS, Lee JH, Lyu AR, Ji HM, Lee SH, et al. Cisplatin ototoxicity involves cytokines and STAT6 signaling network. Cell Res. 2011; 21(6):944–56.
51. Turner MD, Nedjai B, Hurst T, Pennington DJ. Cytokines and chemokines: At the crossroads of cell signalling and inflammatory disease. Biochim Biophys Acta. 2014; 1843(11):2563–82.
52. Kaur T, Mukherjea D, Sheehan K, Jajoo S, Rybak LP, Ramkumar V. Short interfering RNA against STAT1 attenuates cisplatininduced ototoxicity in the rat by suppressing inflammation. Cell Death Dis. 2011; 2(7):e180.
53. Kopke RD, Liu W, Gabaizadeh R, Jacono A, Feghali J, Spray D, et al. Use of organotypic cultures of Corti’s organ to study the protective effects of antioxidant molecules on cisplatin-induced damage of auditory hair cells. Am J Otol. 1997; 18(5):559–71.
54. Bánfi B, Malgrange B, Knisz J, Steger K, Dubois-Dauphin M, Krause KH. NOX3, a superoxide-generating NADPH oxidase of the inner ear. J Biol Chem. 2004; 279(44):46065–72.
55. Church MW, Kaltenbach JA, Blakley BW, Burgio DL. The comparative effects of sodium thiosulfate, diethyldithiocarbamate, fosfomycin and WR-2721 on ameliorating cisplatin-induced ototoxicity. Hear Res. 1995; 86(1-2):195–203.
56. Lynch ED, Gu R, Pierce C, Kil J. Reduction of acute cisplatin ototoxicity and nephrotoxicity in rats by oral administration of allopurinol and ebselen. Hear Res. 2005; 201(1-2):81–9.
57. Mukherjea D, Jajoo S, Sheehan K, Kaur T, Sheth S, Bunch J, et al. NOX3 NADPH oxidase couples transient receptor potential vanilloid 1 to signal transducer and activator of transcription 1-mediated inflammation and hearing loss. Antioxid Redox Signal. 2011; 14(6):999–1010.
58. Laurell G, Ekborn A, Viberg A, Canlon B. Effects of a single high dose of cisplatin on the melanocytes of the stria vascularis in the guinea pig. Audiol Neurootol. 2007; 12(3):170–8.
59. McAlpine D, Johnstone BM. The ototoxic mechanism of cisplatin. Hear Res. 1990; 47(3):191–203.
60. Poirrier AL, Pincemail J, Van Den Ackerveken P, Lefebvre PP, Malgrange B. Oxidative stress in the cochlea: An update. Curr Med Chem. 2010; 17(30):3591–604.
61. Rybak LP, Mukherjea D, Ramkumar V. Mechanisms of cisplatininduced ototoxicity and prevention. Semin Hear. 2019; 40(2):197–204.
62. Mukherjea D, Jajoo S, Kaur T, Sheehan KE, Ramkumar V, Rybak LP. Transtympanic administration of short interfering (si)RNA for the NOX3 isoform of NADPH oxidase protects against cisplatin-induced hearing loss in the rat. Antioxid Redox Signal. 2010; 13(5):589–98.
63. Kim HJ, Lee JH, Kim SJ, Oh GS, Moon HD, Kwon KB, et al. Roles of NADPH oxidases in cisplatin-induced reactive oxygen species generation and ototoxicity. J Neurosci. 2010; 30(11):3933–46.
64. Mukherjea D, Jajoo S, Whitworth C, Bunch JR, Turner JG, Rybak LP, et al. Short interfering RNA against transient receptor potential vanilloid 1 attenuates cisplatin-induced hearing loss in the rat. J Neurosci. 2008; 28(49):13056–65.
65. Sheth S, Mukherjea D, Rybak LP, Ramkumar V. Mechanisms of cisplatin-induced ototoxicity and otoprotection. Front Cell Neurosci. 2017; 11:338.
66. Teitz T, Fang J, Goktug AN, Bonga JD, Diao S, Hazlitt RA, et al. CDK2 inhibitors as candidate therapeutics for cisplatin- and noise-induced hearing loss. J Exp Med. 2018; 215(4):1187–203.
67. Previati M, Lanzoni I, Astolfi L, Fagioli F, Vecchiati G, Pagnoni A, et al. Cisplatin cytotoxicity in organ of Corti-derived immortalized cells. J Cell Biochem. 2007; 101(5):1185–97.
68. Van Houten B, Santa-Gonzalez GA, Camargo M. DNA repair after oxidative stress: Current challenges. Curr Opin Toxicol. 2018; 7:9–16.
69. Kim HJ, Oh GS, Shen A, Lee SB, Choe SK, Kwon KB, et al. Augmentation of NAD(+) by NQO1 attenuates cisplatin-mediated hearing impairment. Cell Death Dis. 2014; 5(6):e1292.
70. Jamesdaniel S, Coling D, Hinduja S, Ding D, Li J, Cassidy L, et al. Cisplatin-induced ototoxicity is mediated by nitroxidative modification of cochlear proteins characterized by nitration of Lmo4. J Biol Chem. 2012; 287(22):18674–86.
71. Jamesdaniel S, Rathinam R, Neumann WL. Targeting nitrative stress for attenuating cisplatin-induced downregulation of cochlear LIM domain only 4 and ototoxicity. Redox Biol. 2016; 10:257–65.
72. Rathinam R, Ghosh S, Neumann WL, Jamesdaniel S. Cisplatininduced apoptosis in auditory, renal, and neuronal cells is associated with nitration and downregulation of LMO4. Cell Death Discov. 2015; 1:15052.
73. Li Y, Li A, Wu J, He Y, Yu H, Chai R, et al. MiR-182-5p protects inner ear hair cells from cisplatin-induced apoptosis by inhibiting FOXO3a. Cell Death Dis. 2016; 7(9):e2362.
74. Devarajan P, Savoca M, Castaneda MP, Park MS, Esteban-Cruciani N, Kalinec G, et al. Cisplatin-induced apoptosis in auditory cells: Role of death receptor and mitochondrial pathways. Hear Res. 2002; 174(1-2):45–54.
75. Alam SA, Ikeda K, Oshima T, Suzuki M, Kawase T, Kikuchi T, et al. Cisplatin-induced apoptotic cell death in Mongolian gerbil cochlea. Hear Res. 2000; 141(1-2):28–38.
76. Lee JS, Kang SU, Hwang HS, Pyun JH, Choung YH, Kim CH. Epicatechin protects the auditory organ by attenuating cisplatininduced ototoxicity through inhibition of ERK. Toxicol Lett. 2010; 199(3):308–16.
77. Orzáez M, Sancho M, Marchán S, Mondragón L, Montava R, Valero JG, et al. Apaf-1 inhibitors protect from unwanted cell death in in vivo models of kidney ischemia and chemotherapy induced ototoxicity. PLoS One. 2014; 9(10):e110979.
78. Zhang M, Liu W, Ding D, Salvi R. Pifithrin-alpha suppresses p53 and protects cochlear and vestibular hair cells from cisplatininduced apoptosis. Neuroscience. 2003; 120(1):191–205.
79. Fernandes-Alnemri T, Wu J, Yu JW, Datta P, Miller B, Jankowski W, et al. The pyroptosome: A supramolecular assembly of ASC dimers mediating inf lammatory cell death via caspase-1 activation. Cell Death Differ. 2007; 14(9):1590–604.
80. Pang J, Xiong H, Zhan T, Cheng G, Jia H, Ye Y, et al. Sirtuin 1 and autophagy attenuate cisplatin-induced hair cell death in the mouse cochlea and zebrafish lateral line. Front Cell Neurosci. 2019; 12:515.
81. Xu FL, Cheng Y, Yan W. Up-regulation of autophagy and apoptosis of cochlear hair cells in mouse models for deafness. Arch Med Sci. 2018; 17(2):535–41.
82. Yin H, Yang Q, Cao Z, Li H, Yu Z, Zhang G, et al. Activation of NLRX1-mediated autophagy accelerates the ototoxic potential of cisplatin in auditory cells. Toxicol Appl Pharmacol. 2018; 343:16–28.
83. Choi MJ, Kang H, Lee YY, Choo OS, Jang JH, Park SH, et al. Cisplatin-induced ototoxicity in rats is driven by RIP3-dependent necroptosis. Cells. 2019; 8(5):409.
84. Wegner KW, Saleh D, Degterev A. Complex pathologic roles of RIPK1 and RIPK3: Moving beyond necroptosis. Trends Pharmacol Sci. 2017; 38(3):202–25.
85. Ruhl D, Du TT, Wagner EL, Choi JH, Li S, Reed R, et al. Necroptosis and apoptosis contribute to cisplatin and aminoglycoside ototoxicity. J Neurosci. 2019; 39(15):2951–64.
86. Lautermann J, Crann SA, McLaren J, Schacht J. Glutathionedependent antioxidant systems in the mammalian inner ear: Effects of aging, ototoxic drugs and noise. Hear Res. 1997; 114(1-2):75–82.
87. Neuwelt EA, Brummett RE, Remsen LG, Kroll RA, Pagel MA, McCormick CI, et al. In vitro and animal studies of sodium thiosulfate as a potential chemoprotectant against carboplatininduced ototoxicity. Cancer Res. 1996; 56(4):706–9.
88. Oh GS, Kim HJ, Choi JH, Shen A, Kim CH, Kim SJ, et al. Activation of lipopolysaccharide-TLR4 signaling accelerates the ototoxic potential of cisplatin in mice. J Immunol. 2011; 186(2):1140–50.
89. Oblak A, Jerala R. Toll-like receptor 4 activation in cancer progression and therapy. Clin Dev Immunol. 2011; 2011:609579.
90. Paulos CM, Wrzesinski C, Kaiser A, Hinrichs CS, Chieppa M, Cassard L, et al. Microbial translocation augments the function of adoptively transferred self/tumor-specific CD8+ T cells via TLR4 signaling. J Clin Invest. 2007; 117(8):2197–204.
91. Ross CJ, Katzov-Eckert H, Dubé MP, Brooks B, Rassekh SR, Barhdadi A, et al. Genetic variants in TPMT and COMT are associated with hearing loss in children receiving cisplatin chemotherapy. Nat Genet. 2009; 41(12):1345–9.
92. Xu H, Robinson GW, Huang J, Lim JY, Zhang H, Bass JK, et al. Common variants in ACYP2 influence susceptibility to cisplatininduced hearing loss. Nat Genet. 2015; 47(3):263–6.
93. Tserga E, Nandwani T, Edvall NK, Bulla J, Patel P, Canlon B, et al. The genetic vulnerability to cisplatin ototoxicity: A systematic review. Sci Rep. 2019; 9(1):3455.
94. Bokemeyer C, Berger CC, Hartmann JT, Kollmannsberger C, Schmoll HJ, Kuczyk MA, et al. Analysis of risk factors for cisplatin-induced ototoxicity in patients with testicular cancer. Br J Cancer. 1998; 77(8):1355–62.
95. Kim KH, Lee B, Kim YR, Kim MA, Ryu N, Jung DJ, et al. Evaluating protective and therapeutic effects of alpha-lipoic acid on cisplatin-induced ototoxicity. Cell Death Dis. 2018; 9(8):827.
96. Kalcioglu MT, Kizilay A, Gulec M, Karatas E, Iraz M, Akyol O, et al. The protective effect of erdosteine against ototoxicity induced by cisplatin in rats. Eur Arch Otorhinolaryngol. 2005; 262(10):856–63.
97. Bhatta P, Dhukhwa A, Sheehan K, Al Aameri RFH, Borse V, Ghosh S, et al. Capsaicin protects against cisplatin ototoxicity by changing the STAT3/STAT1 ratio and activating cannabinoid (CB2) receptors in the cochlea. Sci Rep. 2019; 9:4131.
98. Paciello F, Fetoni AR, Mezzogori D, Rolesi R, Di Pino A, Paludetti G, et al. The dual role of curcumin and ferulic acid in counteracting chemoresistance and cisplatin-induced ototoxicity. Sci Rep. 2020; 10(1):1063.
99. Borse V, Al Aameri RFH, Sheehan K, Sheth S, Kaur T, Mukherjea D, et al. Epigallocatechin-3-gallate, a prototypic chemopreventative agent for protection against cisplatin-based ototoxicity. Cell Death Dis. 2017; 8:e2921.
100. Campbell KC, Rybak LP, Meech RP, Hughes L. D-methionine provides excellent protection from cisplatin ototoxicity in the rat. Hear Res. 1996; 102(1-2):90–8.
101. Kalkanis JG, Whitworth C, Rybak LP. Vitamin E reduces cisplatin ototoxicity. Laryngoscope. 2004; 114(3):538–42.
102. Fernandez K, Spielbauer KK, Rusheen A, Wang L, Baker TG, Eyles S, et al. Lovastatin protects against cisplatin-induced hearing loss in mice. Hear Res. 2020; 389:107905.
103. Benkafadar N, Menardo J, Bourien J, Nouvian R, François F, Decaudin D, et al. Reversible p53 inhibition prevents cisplatin ototoxicity without blocking chemotherapeutic efficacy. EMBO Mol Med. 2017; 9(1):7–26.
104. Sun C, Wang X, Chen D, Lin X, Yu D, Wu H. Dexamethasone loaded nanoparticles exert protective effects against cisplatininduced hearing loss by systemic administration. Neurosci Lett. 2016; 619:142–8.
105. Bhatta P, Dhukhwa A, Sheehan K, Al Aameri RFH, Borse V, Ghosh S, et al. Capsaicin protects against cisplatin ototoxicity by changing the STAT3/STAT1 ratio and activating cannabinoid (CB2) receptors in the cochlea. Sci Rep. 2019; 9(1):4131.
106. Shafik AG, Elkabarity RH, Thabet MT, Soliman NB, Kalleny NK. Effect of intratympanic dexamethasone administration on cisplatin-induced ototoxicity in adult guinea pigs. Auris Nasus Larynx. 2013; 40(1):51–60.
107. Li G, Frenz DA, Brahmblatt S, Feghali JG, Ruben RJ, Berggren D, et al. Round window membrane delivery of L-methionine provides protection from cisplatin ototoxicity without compromising chemotherapeutic efficacy. Neurotoxicology. 2001; 22(2):163–76.
108. Wang J, Lloyd Faulconbridge RV, Fetoni A, Guitton MJ, Pujol R, Puel JL. Local application of sodium thiosulfate prevents cisplatininduced hearing loss in the guinea pig. Neuropharmacology. 2003; 45(3):380–93.
109. Kros CJ, Steyger PS. Aminoglycoside- and cisplatin-induced ototoxicity: Mechanisms and otoprotective strategies. Cold Spring Harb Perspect Med. 2019; 9(11):a033548.
110. Gentilin E, Simoni E, Candito M, Cazzador D, Astolfi L. Cisplatininduced ototoxicity: Updates on molecular targets. Trends Mol Med. 2019; 25(12):1123–32.
Fig. 1.
Cochlear trafficking routes for systemically administered cisplatin. Blood-borne cisplatin enter the cochlea primarily from the capillaries in the stria vascularis. Cisplatin enters endolymph potentially trafficking through OCT2 and CTR1 transporters in the marginal cells. From the endolymph, these ototoxin enters the hair cell across their apical membranes. OCT2, organic cation transporter-2; CTR1, copper-like transport-1; TMC1, transmembrane channel-like protein-1. Adapted from Kros and Steyger. Cold Spring Harb Perspect Med 2019;9(11):a033548 [109].
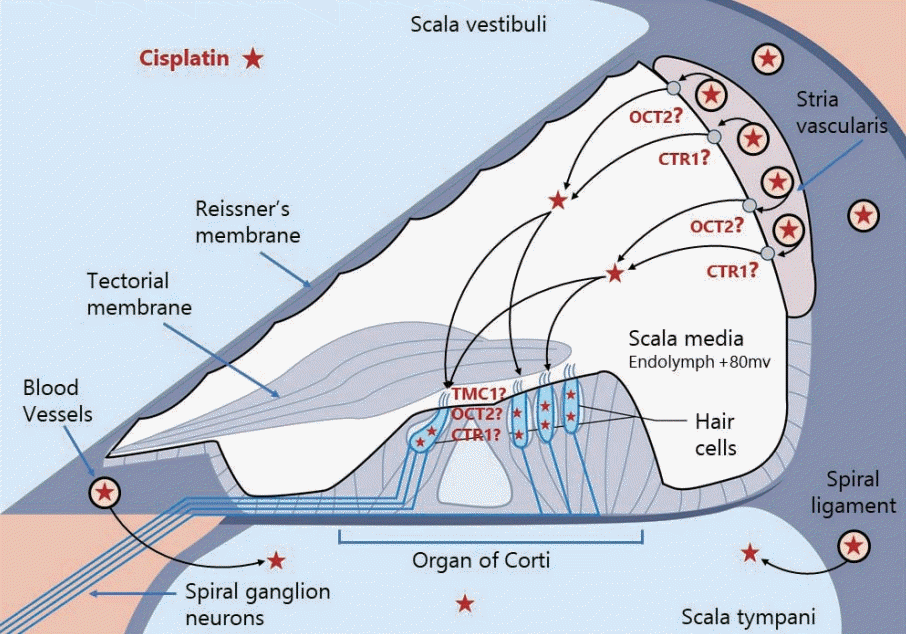
Fig. 2.
Hypothesized mechanism of cisplatin-induced ROS production and related signaling in cochlear cells. Cisplatin induce ROS production in cochlear cells by promoting the release of proinflammatory cytokines, increasing the activity of NADPH oxidases and xanthine oxidase, and depleting the antioxidant defense system. Generated ROS by cisplatin promote inflammation, ROS generation, lipid peroxidation, protein nitration, and apoptosis. BAX, Bcl-2-like protein 4; Bcl2, B-cell lymphoma 2; COX2, cyclooxygenase 2; ERK1, extracellular signal-regulated kinase 1; iNOS, inducible nitric oxide synthase; IL, interleukin; NO, nitric oxide; ROS, reactive oxygen species; STAT1, signal transducer and activator of transcription 1; TNF-α, tumor necrosis factor α; APAF, apoptotic protease activating factor. Adapted from Gentilin, et al. Trends Mol Med 2019;25(12):1123-32, with permission of Elsevier Ltd [110].
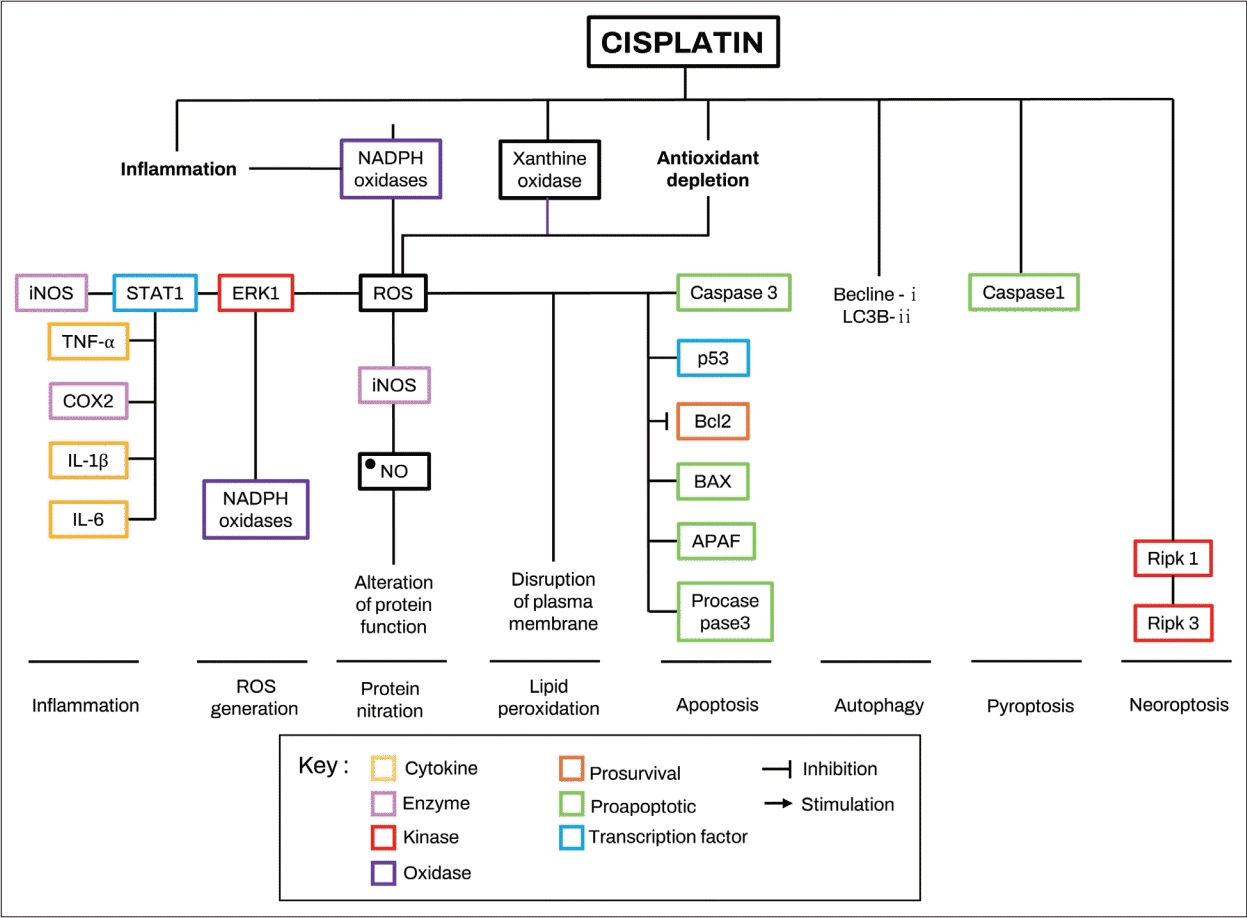
Table 1.
Summary of vulnerable gene to cisplatin ototoxicity
Table 2.
Summary of otoprotective drug on cisplatin ototoxicity in various animal models
Drugs | Animal/delivery route | Mechanism of action | Ref |
---|---|---|---|
Allicin | Rat/IP | Anti-oxidant | [28] |
Alpha-lipoic acid | Mouse/IP | Anti-oxidant | [95] |
Allopurinol | Rat/oral | Xanthine oxidase inhibitor | [56] |
Amifostine | Hamster/IP | Free radical scavenger | [55] |
β-lapachone (NAD+) | Rat/oral | Anti-oxidant | [69] |
Capsaicin | Rat/oral | TRPV1 agonist that desensitizes CB2R agonist | [97, 105] |
Rat/intratympanic | |||
Curcumin | Rat/IP | Upregulation of Nrf2/HO-1 pathway and modulating the p53, STAT3 and NF-kB activation | [98] |
Copper sulfate | Mouse/intratympanic | CTR1 inhibitor | [35] |
D-methionine (D-Met) | Rat/IP | Anti-oxidant | [100] |
Dexamethasone | Guinea pig/intratympanic | Anti-inflammatory | [106] |
Dexamethasone loaded nanoparticles (PEG-PLA) | Guinea pig/IP | Anti-inflammatory | [104] |
Ebselen | Rat/oral | Glutathione peroxidase mimic | [56, 61] |
EGCG | Rat/oral | STAT1 inhibition | [99] |
Erdosteine | Rat, guinea pig/IP | Anti-oxidant and free radical scavenger | [96] |
Etanercept | Rat/intratympanic | TNF-alpha inhibitor | [52] |
L-methionine | Rat/round-window | Anti-oxidant | [107] |
Lovastatin | Mouse/oral gavage | Inhibiting 3- HMG-CoA enzyme | [102] |
Pifithrin-α | Mouse/IP | p53 inhibitor | [103] |
Sodium thiosulphate | Guinea pig/cochlear perfusion | Anti-oxidant | [108] |
Vitamin E (α-tocopheryl succinate) | Rat, guinea pig/IP | Free radical scavenger | [101] |