Abstract
Interventional oncology (IO) local therapies of hepatocellular carcinoma (HCC) can activate anti-cancer immunity and it is potentially leading to an anti-cancer immunity throughout the body. For the development of an effective HCC treatment regime, great emphasis has been dedicated to different IO local therapy mediated immune modulation and possible combinations with immune checkpoint inhibitor immunotherapy. In this review paper, we summarize the status of combination of IO local therapy and immunotherapy, as well as the prospective role of therapeutic carriers and locally administered immunotherapy in advanced HCC.
Most systemic and regional therapies for hepatocellular carcinoma (HCC) offer palliation rather than cure. Systemic chemotherapy offers limited survival benefit 1,2. The first line systemic sorafenib therapy has shown less than 1 year median survival time and the tumor response rate of less than 5%. Local ablation therapies, including thermal and chemical ablation, have limited efficacy with significant recurrence 3,4. Representatively, the 5-year overall survival (OS) of radiofrequency ablation (RFA) has been reported as 40.1-86.0% 5,6, but recurrence after ablation of early-stage HCC occurs in up to 60-85% of patients by 5 years 7. Other treatment options include catheter-directed therapies, such as transcatheter arterial embolization, transarterial chemoembolization (TACE), and 90Y (yttrium)-radioembolization (90Y-RE) 8. Catheter directed therapies improve liver cancer patient survival but the overall prognosis of these patients remains poor with potential metastasis 9,10. The overall median survival of the catheter directed therapies is about 8.0-30.0 months 11. As demonstrated promising immuno-therapeutic outcomes in various types of tumors such as melanoma, lung cancer and renal cell carcinoma and so on 12, immune checkpoint inhibitors (ICIs) immunotherapy have emerged as an effective and promising treatment for HCC 13,14. Currently, the US Food and Drug Administration (FDA) approved ICIs have been being evaluated for the treatment of HCC in clinical trials (Table 1). Nivolumab (programmed cell death protein-1, PD-1) ICI was approved for the treatment of advanced HCC patients after sorafenib treatment by FDA with an accelerated process. FDA also granted the use of pembrolizumab for the HCC with a clinical result of 20% objective tumor remission rate and prolonged survival. However, following this FDA approvals, phase III studies of single-agent nivolumab (CheckMate 459) and pembrolizumab (KEYNOTE-240) in the first line and second-line settings, respectively, did not meet their primary overall survival end points 14,15. Nivolumab monotherapy was voluntarily withdrawn from the US market. Unique immune suppressive tumor microenvironment (TME) of HCC might be a significant challenge to achieve satisfactory therapeutic efficacy level of ICI monotherapies. Indeed, TME of HCC is dominated by various immunosuppressive cells including macrophages (Kupffer cells), monocyte-derived macrophages, regulatory T (Treg) cells and myeloid-derived suppressor cells (MDSCs) and signals that foster immunosuppressive roles implicated in HCC immune evasion 16.
Additional therapeutics which can convert immune suppressive TME in HCC are required. Recent studies revealed that the response to PD-1/programmed cell death ligand-1 (PD-L1) ICI immunotherapy significantly relies on a pre-existing immune status. Various immunogenic interventional oncology (IO) local therapies such as RFA, cryoablation, percutaneous ethanol ablation, irreversible electroporation, TACE, 90Y-RE and so on that can overturn the immune suppressive TME of HCC have been actively investigated in clinical trials. However, finding optimal synergistic combination and managing the treatment-related adverse effects (TRAEs) or immune-related adverse effects (irAEs) are the main challenges. More understanding on immune response of IO local therapies and subsequent evaluation for the synergistic combination with ICI immunotherapies are required. Development of new therapeutic regimens with advanced image guide technique and therapeutic delivery technologies will be imperative tasks for advancing immunotherapy for the treatment of HCC. Recent development of various multifunctional carriers and locally administered immunotherapy will allow enhanced immunotherapy of HCC. Here we are summarizing recent progress of combination of IO local therapies and ICI immunotherapy. Future direction and potential role of therapeutic carriers and local combination immunotherapy for an advanced immuno-therapeutic of HCC will be discussed.
IO local therapies treating the primary tumor induces the shrinkage of untreated distant tumors as known as abscopal effect. The immunogenicity of IO local therapies can activate antigen presenting cells (APCs) being recognized by the dendritic cells and it is potentially activating an anti-cancer immunity throughout the body. Indeed, the investigation of various IO local therapy mediated immune modulation and anti-cancer immunity are now in great interest for the potential combination with ICI immunotherapy. Such local tumoral accessibility of clinical IO therapies makes HCC ideal for the local interventions that can cause immunogenic cell death (ICD) or local immune conversion in immune suppressive TME of HCC.
ICD induced by IO local therapies commonly can convert the immune suppressive TME in HCC. ICD releases the tumor-associated antigens, high mobility group box 1, and adenosine triphosphate to recruit the various immune cells to TME and expresses the surface calreticulin as a “eat-me” signal. Circulating phagocytic APCs accumulate to immunogenic TME by ICD and subsequently synergize with ICI cancer immunotherapy (Fig. 1) 17. Therefore, various kinds of clinical trials in a different combinations of immunogenic IO local therapy and ICI immunotherapy are on-going to improve the overall therapeutic outcomes and survival benefit versus monotherapy 18-20. Recently, Duffy et al. showed enhanced cytotoxic lymphocytes (CTLs) accumulation in the tumor after a synergistic combination of anti-cytotoxic T lymphocyte-associated antigen-4 (aCTLA-4) immunotherapy and various ablation techniques such as TACE, RFA, and cryoablation 21,22. Partial tumor ablation with RFA or TACE in advanced HCC patients receiving systemic tremelimumab resulted in a response rate of 26% and a disease control rate of 89%, with 45% of the stabilizations lasting longer than 6 months, and an overall survival of 12.3 months 23. These encouraging data have triggered many different combinational clinical trials in which systemically administered ICIs are given in combination with IO local therapies of ablations, TACE or TARE. Percutaneous ablation (KEYNOTE-937 [NCT03867084], EMRALD-2 [NCT03847428], CHECKMATE-9DX [NCT03383458], IMBRAVE-050 [NCT04102098], and so on), TACE (EMRALD-1 [NCT03778957], CHECKMATE-74W [NCT04340193], TACE3 [NCT04268888], and so on), and ROWAN [NCT05063565], and so on) are primarily ongoing to evaluate the various forms of combination IO local therapies and ICI immunotherapy. Additional information is added in Table 2 and more details can be found in other review papers 11,24,25. Indeed, combination of IO local therapy and ICI immunotherapy is an emerging strategy to overcome current challenges of both IO local therapies and immunotherapies. More effort to develop the image guided combination IO local therapy and ICI immunotherapy are urgently required to establish optimal benefit of combination IO local therapy and immunotherapy in overall therapeutic outcomes and safety.
Combination of IO local therapy and ICI immunotherapy is promising for enhancing the therapeutic efficacy. However, current standard approaches to combine IO local therapies with systemically administered ICI immunotherapy are initiated based on minimum data with a shortage of clinical information. Most recent data showed that systemically administered ICI immunotherapy can induce therapeutic resistance/ignorance and severe side effects involved with autoimmunity. When it combined with IO local therapies, additional TRAEs can be occurred and ended up being moderate therapeutic outcomes. Severe side effect (Grade 3 or 4) incidence has been reported as high as 90% in the combination of systemic ICIs immunotherapies following an IO local therapy 26.
Current ICI immunotherapies are performed with systemic administration of anti-CTLA4, anti-PD-1 (aPD-1) or anti-PD-L1 (aPD-L1) immunoglobulin G (IgG) based monoclonal antibodies (mAbs). Those ICI mAbs may not be effective to achieve an anti-cancer immune response with IO local therapies in immune-suppressive HCC 27-29. Upon systemic administration of mAb, non-specific binding and short circulation time of ICI mAbs can affect the therapeutic efficacy 30. Current ICI mAbs are mostly humanized or human IgG antibodies. The pharmacokinetics of ICI mAbs are similar with other therapeutic mAbs in the systemic administration. Systemically administrated ICI mAbs circulate in the central vasculature and are distributed to peripheral tissues and tumors. During the circulation, off-target binding with IC molecules of normal tissues and proteolytic clearance limits the tumor specific ICI mAb dose 30-32.
The limited pharmacokinetics of ICI mAbs can induce an excessive immune response after combination of ICI immunotherapy and IO local therapy 33. These symptoms are mostly accompanied with the pneumonitis, colitis, hepatitis, myocarditis, as a category of irAEs 34. Steroid-based treatments are commonly given to suppress the immune responses. Those irAEs and concurrent immunosuppressive treatment subsequently reduces the efficacy of immunotherapy by increasing incident rate and mortality 35. In the clinical data, 85% patients treated with ipilimumab (aCTLA-4) ICI immunotherapy in monotherapy experienced irAEs 36. 26% patient treated with PD-1 ICIs (nivolumab, pembrolizumab, and cemiplimab), and 14% patient treated with PD-L1 ICIs (atezolizumab, avelumab, and durvalumab) showed irAEs 37. The combination of ICIs with IO local therapy for better therapeutic response led to more severe incidence of irAEs (93%) 38-41. The combination ICI immunotherapies and IO local therapy might need additional consideration of additive side effect of IO local therapies. Unfortunately, once irAEs is occurred with autoimmunity, discontinued ICI immunotherapies might not be resumed with immunological memory effect 42. Intensive investigation of minimizing TRAEs including irAEs is required to find the synergistic combination of IO local therapy and ICI immunotherapy.
Development of new strategy to enhance targeting and controlled release of ICI molecules at desired immune activation sites is the key to increase the response rates and control the TRAEs of combination IO local therapy and ICI immunotherapy. Multifunctional carriers including injectable therapeutic carriers, nanocarriers, and local administration routes may overcome physical TME barriers and enhance the controlled immune modulation for the treatment of HCC.
Drug delivery carriers have shown excellence in improving the pharmacokinetics of anti-cancer agents. Currently, 45 different nano-drug carrier-formulations have been approved for the clinical uses by the FDA, and over 80 clinical trials are on-going to evaluate the potential clinical translation of nanocarriers 43. Nanocarriers basically provides high surface area where can load various therapeutic molecules and the size scale is compatible with cellular component allowing easy penetration. An enhanced permeability and retention (EPR) effect using the characteristic high permeability of tumor vessels and the retention effect in tumors by poor lymphatic clearance demonstrated the potential of nanocarriers for delivering ICI mAbs 44. Active targeting utilizing tumor specific molecules can further increase targeting efficiency of nanocarriers. In preclinical studies, nanocarriers have been suggested for the delivery of various immunotherapies, as a form of nano-immunotherapy. For ICI cancer immunotherapy, nanocarriers incorporating ICI molecules have suggested to improve the therapeutic efficacy of ICI immunotherapy. Many preclinical studies have demonstrated the enhanced targeted delivery of ICI and sustained ICI release of ICI loaded nanocarriers 45-48. Various ICI mAb conjugated nanocarriers have shown enormous potential to improve the efficacy of ICI immunotherapy and combinational ICI immunotherapy 49-51. However, ICI mAb-nanocarriers often lose the available Fab which can bind with immune checkpoints and at the same time, FcγR of ICI mAb are exposed outside that causes rapid clearance with the FcγR mediated endocytosis 52-55. More efforts to improve the ICI mAbs loading protocol is necessary for the high affinity and specificity of ICI mAb-nanocarrier 56. Beyond the nanocarriers, injectable carriers are a promising approach to deliver and release ICI locally and combine additional IO local therapy together. Lipiodol, iodinated ethyl esters of fatty acids from poppy seed oil, exhibits transient and plastic embolic effects and facilitates localized delivery of doxorubicin to HCC during cTACE of HCC 57,58. The development of lipiodol-based formulations or various injectable gels that can enhance targeted ICI delivery may allow an opportunity for safe incorporation of potent ICI immunomodulatory agents with IO local therapies.
Current limitations relying on systemic administration of ICI immunotherapy and ICI loaded carriers might be overcome with image guided local ICI administration route 59. Image guided local delivery including intra-tumoral injection and tumor associated vascular injection may result in high doses of ICI combination therapy in local tumor and TME without systemic exposure of toxic therapeutics. HCC receives most of their blood supply from hepatic arteries unlike the normal liver. Even hepatic metastases >3 mm derive 80-100% of their blood supply from the hepatic arterial rather than the portal venous circulation 60. Moreover, the density of arterial vessels around a metastatic lesion is estimated to be 3 times more than in normal liver tissue 61. Thus, if ICI molecules or ICI loaded carriers are infused into the hepatic artery, the infused dose preferentially reaches the tumor as opposed to the normal liver. Currently, ICI agents, lipiodol, gel-form, microspheres, nanocarriers and so on have been tested for the hepatic intra-arterial infusion for high local delivery of therapeutics in HCC 46,62-68. During the IA infusion, MRI, CT and X-ray angiography are used to practice tumor specific hepatic arterial infusion, monitor the procedure, and confirm the distribution of infused therapeutics. A phase III clinical trial (NCT03949231) is ongoing to compare the effects of IA infusion and IV administration of PD-1/PD-L1 ICIs on the survival benefit of patients with advanced liver cancer, including ORR, DCR, median survival time, and safety. Clinical trials (NCT04945720 and NCT04191889) also are testing IA infused chemotherapy and aPD-1/aPD-L1 (durvaluamab or camrelizumab) mixture for the efficacy and safety in advanced HCC. A clinical trial (NCT02850536) also testing hepatic arterials infusion of CAR-T for CEA-Expressing Liver Metastases. Another clinical trial (NCT04823403) is investigating the optimized dosage of hepatic IA administration of Ipilimumab in combination with IV administered nivolumab for advanced HCC (HIPANIV).
Percutaneous intra-tumoral therapeutic delivery also plays a key role in the management of HCC. Percutaneous intratumoral ethanol injection is a well-established technique for the treatment of HCC 69. Ultrasound real-time guidance of intratumoral ethanol injection allows faster procedure, precise centering of the needle in the tumor target, and continuous monitoring of the injection. This local injection is conveniently performed under local anesthesia on an out-patient basis and the treatment sessions and schedule can be flexible according to the distribution of the injected ethanol within the tumor and the prognosis. Several clinical trials of local intratumoral administration of immunotherapy are on-going. Intratumorally injected aPD-1 and aCTLA-4 ICIs (NCT03058289) are being tested in HCC. A phase 1 clinical trials are testing tumor targeted injected TLR9 agonist CpG oligonucleotides and OX40 agonist (NCT03831295). Phase I-II study (NCT03792724) evaluates the safety and activity of intratumoral urelumab combined with systemic nivolumab in patients with advanced solid tumors. Additionally, intracavitary infusions 70,71 and the direct lymph node infusion could be available for the local delivery of toxic immune adjuvants.
Various clinical trials evaluating the combination of IO local therapies and ICI immunotherapy has been tested and promising interim data has been released 11. When the IO local therapies are combined with ICI immunotherapy, the median survival, ORR, PFS are surpassing those indications of IO local therapy alone. It is implicating the rationale for the combination of IO local therapy and ICI immunotherapy can be synergistic (Fig. 1). Although additional robust clinical evidence is further required, the development of various synergistic combination strategies should be investigated in a consideration of TRAEs. Since each IO local therapy and ICI monotherapy itself showed high percentage of complications, new approaches that can minimize side effect of the combination IO local therapy and ICI immunotherapy are urgently required. Specially, those side effect can easily impair liver functions during the HCC treatment, lowered side effect during the combination will be an important consideration. Developed multifunctional therapeutic carriers and effective local delivery routes can critically contribute to the safe and effective combination of IO local therapy and ICI immunotherapy. Carriers mediated ICI delivery, controlled ICI release, and immune modulation have demonstrated the effectiveness to overcome the ICI therapeutic tumor resistance, ignorance, and off-target side effect (irAEs). Additionally, the multifunctionality of carriers in imaging and therapeutic delivery have shown excellent potential for improving the interventional procedures. Development of delivery route for ICI immunotherapy or ICI loaded carriers is another essential component to further enhance the therapeutic efficacy of combination IO local therapy and ICI immunotherapy. Most of ICI immunotherapy has been tested with systemic administration. The efficacy evaluation of local administration of ICI immunotherapy comparing to systemic administration of ICI has been initiated recently. Established hepatic artery local administration and percutaneous intra-tumoral administration routes in HCC will allow rapid development and optimization of local ICI immunotherapy and the combination of IO local therapy and ICI immunotherapy. During the process, current ICI dosage regime should be revised for each administration routes and in-depth biodistribution studies are further needed. Additionally, ICI residence time and following time-dependent immunity changes after the locally administered combination immunotherapy should be investigated and compared with systemic administration of ICI immunotherapy. Optimal sequencing and interval of IO local therapies and ICI immunotherapy in the combination also need to be investigated. Lastly, it is critical to develop key biomarkers that can identify immune response and therapeutic response to the combination of IO local therapy and ICI immunotherapy. Considering the complexity of the immune suppressive TME and anti-cancer immunity in HCC, substantial effort is required to integrate multifunctional carriers and image guided local delivery technique into the novel combination of IO local therapy and ICI immunotherapy strategies for treating various stages of HCC safely and effectively.
ACKNOWLEDGMENTS
The author appreciates all former and current BIGMed lab members.
Illustrations were originally created by authors through Biorender.
Notes
Conflicts of Interest
D.H.K, a contributing editor of the Journal of Liver Cancer, was not involved in the editorial evaluation or decision to publish this article.
Funding Statement
This work was mainly supported by grants R01CA218659 and R01EB026207 from the National Cancer Institute and National Institute of Biomedical Imaging and Bioengineering.
REFERENCES
1. Chow PK, Tai BC, Tan CK, Machin D, Win KM, Johnson PJ, et al. High-dose tamoxifen in the treatment of inoperable hepatocellular carcinoma: a multicenter randomized controlled trial. Hepatology. 2002; 36:1221–1226.
2. Lai CL, Wu PC, Chan GC, Lok AS, Lin HJ. Doxorubicin versus no antitumor therapy in inoperable hepatocellular carcinoma. A prospective randomized trial. Cancer. 1988; 62:479–483.
3. Ahmed M, Goldberg SN. Thermal ablation therapy for hepatocellular carcinoma. J Vasc Interv Radiol. 2002; 13:S231–S244.
4. Clark TW, Soulen MC. Chemical ablation of hepatocellular carcinoma. J Vasc Interv Radiol. 2002; 13:S245–S252.
5. Vitale A, Peck-Radosavljevic M, Giannini EG, Vibert E, Sieghart W, Van Poucke S, et al. Personalized treatment of patients with very early hepatocellular carcinoma. J Hepatol. 2017; 66:412–423.
6. European Association for the Study of the Liver. EASL clinical practice guidelines: management of hepatocellular carcinoma. J Hepatol. 2018; 69:182–236.
7. N'Kontchou G, Mahamoudi A, Aout M, Ganne-Carrié N, Grando V, Coderc E, et al. Radiofrequency ablation of hepatocellular carcinoma: long-term results and prognostic factors in 235 Western patients with cirrhosis. Hepatology. 2009; 50:1475–1483.
8. Ackerman NB. Experimental studies on the circulation dynamics of intrahepatic tumor blood supply. Cancer. 1972; 29:435–439.
9. Marelli L, Stigliano R, Triantos C, Senzolo M, Cholongitas E, Davies N, et al. Transarterial therapy for hepatocellular carcinoma: which technique is more effective? A systematic review of cohort and randomized studies. Cardiovasc Intervent Radiol. 2007; 30:6–25.
10. Biolato M, Marrone G, Racco S, Di Stasi C, Miele L, Gasbarrini G, et al. Transarterial chemoembolization (TACE) for unresectable HCC: a new life begins? Eur Rev Med Pharmacol Sci. 2010; 14:356–362.
11. Llovet JM, De Baere T, Kulik L, Haber PK, Greten TF, Meyer T, et al. Locoregional therapies in the era of molecular and immune treatments for hepatocellular carcinoma. Nat Rev Gastroenterol Hepatol. 2021; 18:293–313.
12. Yarchoan M, Albacker LA, Hopkins AC, Montesion M, Murugesan K, Vithayathil TT, et al. PD-L1 expression and tumor mutational burden are independent biomarkers in most cancers. JCI Insight. 2019; 4:e126908.
13. Kudo M. Immune checkpoint blockade in hepatocellular carcinoma. Liver Cancer. 2015; 4:201–207.
14. El-Khoueiry AB, Melero I, Crocenzi TS, Welling TH, Yau TC, Yeo WN, et al. Phase I/II safety and antitumor activity of nivolumab in patients with advanced hepatocellular carcinoma (HCC): CA209-040. J Clin Oncol. 2015; 33 Suppl 15:LBA101.
15. Rizvi NA, Mazières J, Planchard D, Stinchcombe TE, Dy GK, Antonia SJ, et al. Activity and safety of nivolumab, an anti-PD-1 immune checkpoint inhibitor, for patients with advanced, refractory squamous non-small-cell lung cancer (CheckMate 063): a phase 2, single-arm trial. Lancet Oncol. 2015; 16:257–265.
16. Ringelhan M, Pfister D, O'Connor T, Pikarsky E, Heikenwalder M. The immunology of hepatocellular carcinoma. Nat Immunol. 2018; 19:222–232.
17. Krysko DV, Garg AD, Kaczmarek A, Krysko O, Agostinis P, Vandenabeele P. Immunogenic cell death and DAMPs in cancer therapy. Nat Rev Cancer. 2012; 12:860–875.
18. Lu J, Liu X, Liao YP, Salazar F, Sun B, Jiang W, et al. Nano-enabled pancreas cancer immunotherapy using immunogenic cell death and reversing immunosuppression. Nat Commun. 2017; 8:1811.
19. Emens LA, Middleton G. The interplay of immunotherapy and chemotherapy: harnessing potential synergies. Cancer Immunol Res. 2015; 3:436–443.
20. El-Khoueiry AB, Sangro B, Yau T, Crocenzi TS, Kudo M, Hsu C, et al. Nivolumab in patients with advanced hepatocellular carcinoma (CheckMate 040): an open-label, non-comparative, phase 1/2 dose escalation and expansion trial. Lancet. 2017; 389:2492–2502.
21. Xie C, Duffy AG, Mabry-Hrones D, Wood B, Levy E, Krishnasamy V, et al. Tremelimumab in combination with microwave ablation in patients with refractory biliary tract cancer. Hepatology. 2019; 69:2048–2060.
22. Duffy AG, Ulahannan SV, Makorova-Rusher O, Rahma O, Wedemeyer H, Pratt D, et al. Tremelimumab in combination with ablation in patients with advanced hepatocellular carcinoma. J Hepatol. 2017; 66:545–551.
23. Kudo M. Immuno-oncology therapy for hepatocellular carcinoma: current status and ongoing trials. Liver Cancer. 2019; 8:221–238.
24. Sangro B, Sarobe P, Hervás-Stubbs S, Melero I. Advances in immunotherapy for hepatocellular carcinoma. Nat Rev Gastroenterol Hepatol. 2021; 18:525–543.
25. Liu Z, Liu X, Liang J, Liu Y, Hou X, Zhang M, et al. Immunotherapy for hepatocellular carcinoma: current status and future prospects. Front Immunol. 2021; 12:765101.
26. Zhan C, Ruohoniemi D, Shanbhogue KP, Wei J, Welling TH, Gu P, et al. Safety of combined yttrium-90 radioembolization and immune checkpoint inhibitor immunotherapy for hepatocellular carcinoma. J Vasc Interv Radiol. 2020; 31:25–34.
27. Prieto J, Melero I, Sangro B. Immunological landscape and immunotherapy of hepatocellular carcinoma. Nat Rev Gastroenterol Hepatol. 2015; 12:681–700.
28. Greten TF, Wang XW, Korangy F. Current concepts of immune based treatments for patients with HCC: from basic science to novel treatment approaches. Gut. 2015; 64:842–848.
29. Tsuchiya N, Sawada Y, Endo I, Uemura Y, Nakatsura T. Potentiality of immunotherapy against hepatocellular carcinoma. World J Gastroenterol. 2015; 21:10314–10326.
30. Centanni M, Moes DJAR, Trocóniz IF, Ciccolini J, van Hasselt JGC. Clinical pharmacokinetics and pharmacodynamics of immune checkpoint inhibitors. Clin Pharmacokinet. 2019; 58:835–857.
31. Keizer RJ, Huitema AD, Schellens JH, Beijnen JH. Clinical pharmacokinetics of therapeutic monoclonal antibodies. Clin Pharmacokinet. 2010; 49:493–507.
32. Riley RS, June CH, Langer R, Mitchell MJ. Delivery technologies for cancer immunotherapy. Nat Rev Drug Discov. 2019; 18:175–196.
33. Friedman CF, Proverbs-Singh TA, Postow MA. Treatment of the immune-related adverse effects of immune checkpoint inhibitors: a review. JAMA Oncol. 2016; 2:1346–1353.
34. Puzanov I, Diab A, Abdallah K, Bingham CO 3rd, Brogdon C, Dadu R, et al. Managing toxicities associated with immune checkpoint inhibitors: consensus recommendations from the Society for Immunotherapy of Cancer (SITC) Toxicity Management Working Group. J Immunother Cancer. 2017; 5:95.
35. Wang DY, Salem JE, Cohen JV, Chandra S, Menzer C, Ye F, et al. Fatal toxic effects associated with immune checkpoint inhibitors: a systematic review and meta-analysis. JAMA Oncol. 2018; 4:1721–1728.
36. Horvat TZ, Adel NG, Dang TO, Momtaz P, Postow MA, Callahan MK, et al. Immune-related adverse events, need for systemic immunosuppression, and effects on survival and time to treatment failure in patients with melanoma treated with ipilimumab at Memorial Sloan Kettering Cancer Center. J Clin Oncol. 2015; 33:3193–3198.
37. Weber JS, Hodi FS, Wolchok JD, Topalian SL, Schadendorf D, Larkin J, et al. Safety profile of nivolumab monotherapy: a pooled analysis of patients with advanced melanoma. J Clin Oncol. 2017; 35:785–792.
38. Wolchok JD, Chiarion-Sileni V, Gonzalez R, Rutkowski P, Grob JJ, Cowey CL, et al. Overall survival with combined nivolumab and ipilimumab in advanced melanoma. N Engl J Med. 2017; 377:1345–1356.
39. Hodi FS, Chesney J, Pavlick AC, Robert C, Grossmann KF, McDermott DF, et al. Combined nivolumab and ipilimumab versus ipilimumab alone in patients with advanced melanoma: 2-year overall survival outcomes in a multicentre, randomised, controlled, phase 2 trial. Lancet Oncol. 2016; 17:1558–1568.
40. Hellmann MD, Paz-Ares L, Bernabe Caro R, Zurawski B, Kim SW, Carcereny Costa E, et al. Nivolumab plus ipilimumab in advanced non-small-cell lung cancer. N Engl J Med. 2019; 381:2020–2031.
41. Larkin J, Hodi FS, Wolchok JD. Combined nivolumab and ipilimumab or monotherapy in untreated melanoma. N Engl J Med. 2015; 373:1270–1271.
42. Nakajima EC, Lipson EJ, Brahmer JR. Challenge of rechallenge: when to resume immunotherapy following an immune-related adverse event. J Clin Oncol. 2019; 37:2714–2718.
43. Choi YH, Han HK. Nanomedicines: current status and future perspectives in aspect of drug delivery and pharmacokinetics. J Pharm Investig. 2018; 48:43–60.
44. Kim DH. Image-Guided Cancer Nanomedicine. J Imaging. 2018; 4:18.
45. Yu B, Zhang W, Kwak K, Choi H, Kim DH. Electric pulse responsive magnetic nanoclusters loaded with indoleamine 2,3-dioxygenase inhibitor for synergistic immuno-ablation cancer therapy. ACS Appl Mater Interfaces. 2020; 12:54415–54425.
46. Choi B, Choi H, Yu B, Kim DH. Synergistic local combination of radiation and anti-programmed death ligand 1 immunotherapy using radiation-responsive splintery metallic nanocarriers. ACS Nano. 2020; 14:13115–13126.
47. Yu B, Choi B, Li W, Kim DH. Magnetic field boosted ferroptosis-like cell death and responsive MRI using hybrid vesicles for cancer immunotherapy. Nat Commun. 2020; 11:3637.
48. Choi B, Jung H, Yu B, Choi H, Lee J, Kim DH. Sequential MR imageguided local immune checkpoint blockade cancer immunotherapy using ferumoxytol capped ultralarge pore mesoporous silica carriers after standard chemotherapy. Small. 2019; 15:e1904378.
49. Wang D, Wang T, Yu H, Feng B, Zhou L, Zhou F, et al. Engineering nanoparticles to locally activate T cells in the tumor microenvironment. Sci Immunol. 2019; 4:e. aau6584.
50. Bu J, Nair A, Iida M, Jeong WJ, Poellmann MJ, Mudd K, et al. An avidity-based PD-L1 antagonist using nanoparticle-antibody conjugates for enhanced immunotherapy. Nano Lett. 2020; 20:4901–4909.
51. Jiang CT, Chen KG, Liu A, Huang H, Fan YN, Zhao DK, et al. Immunomodulating nano-adaptors potentiate antibody-based cancer immunotherapy. Nat Commun. 2021; 12:1359.
52. Wilhelm S, Tavares AJ, Dai Q, Ohta S, Audet J, Dvorak HF, et al. Analysis of nanoparticle delivery to tumours. Nat Rev Mater. 2016; 1:16014.
53. Blanco E, Shen H, Ferrari M. Principles of nanoparticle design for overcoming biological barriers to drug delivery. Nat Biotechnol. 2015; 33:941–951.
54. Bertrand N, Grenier P, Mahmoudi M, Lima EM, Appel EA, Dormont F, et al. Mechanistic understanding of in vivo protein corona formation on polymeric nanoparticles and impact on pharmacokinetics. Nat Commun. 2017; 8:777.
55. Desnoyer A, Broutin S, Delahousse J, Maritaz C, Blondel L, Mir O, et al. Pharmacokinetic/pharmacodynamic relationship of therapeutic monoclonal antibodies used in oncology: part 2, immune checkpoint inhibitor antibodies. Eur J Cancer. 2020; 128:119–128.
56. Yong KW, Yuen D, Chen MZ, Porter CJH, Johnston APR. Pointing in the right direction: controlling the orientation of proteins on nanoparticles improves targeting efficiency. Nano Lett. 2019; 19:1827–1831.
57. Kwak K, Yu B, Mouli SK, Larson AC, Kim DH. Sodium cholate bile acid-stabilized ferumoxytol-doxorubicin-lipiodol emulsion for transcatheter arterial chemoembolization of hepatocellular carcinoma. J Vasc Interv Radiol. 2020; 31:1697–1705. e3.
58. Park W, Cho S, Kang D, Han JH, Park JH, Lee B, et al. Tumor microenvironment targeting nano-bio emulsion for synergistic combinational X-Ray PDT with oncolytic bacteria therapy. Adv Healthc Mater. 2020; 9:e1901812.
59. Choi B, Kim DH. Multifunctional nanocarriers-mediated synergistic combination of immune checkpoint inhibitor cancer immunotherapy and interventional oncology therapy. Adv NanoBiomed Res. 2021; 1:100010.
60. Lien WM, Ackerman NB. The blood supply of experimental liver metastases. II. A microcirculatory study of the normal and tumor vessels of the liver with the use of perfused silicone rubber. Surgery. 1970; 68:334–340.
61. Kennedy AS, Nutting C, Coldwell D, Gaiser J, Drachenberg C. Pathologic response and microdosimetry of (90)Y microspheres in man: review of four explanted whole livers. Int J Radiat Oncol Biol Phys. 2004; 60:1552–1563.
62. Choi H, Choi B, Yu B, Li W, Matsumoto MM, Harris KR, et al. Ondemand degradable embolic microspheres for immediate restoration of blood flow during image-guided embolization procedures. Biomaterials. 2021; 265:120408.
63. Ji J, Park WR, Cho S, Yang Y, Li W, Harris K, et al. Iron-oxide nanocluster labeling of clostridium novyi-NT spores for MR imaging-monitored locoregional delivery to liver tumors in rat and rabbit models. J Vasc Interv Radiol. 2019; 30:1106–1115. e1.
64. Cho S, Min NG, Park W, Kim SH, Kim DH. Janus microcarriers for magnetic field-controlled combination chemotherapy of hepatocellular carcinoma. Adv Funct Mater. 2019; 29:1901384.
65. Matsumoto MM, Kim DH, Larson AC, Mouli SK. Interventional nanotheranostics: advancing nanotechnology applications with IR. J Vasc Interv Radiol. 2019; 30:1824–1829. e1.
66. Gournaris E, Park W, Cho S, Bentrem DJ, Larson AC, Kim DH. Nearinfrared fluorescent endoscopic image-guided photothermal ablation therapy of colorectal cancer using dual-modal gold nanorods targeting tumor-infiltrating innate immune cells in a transgenic TS4 CRE/APC loxΔ468 mouse model. ACS Appl Mater Interfaces. 2019; 11:21353–21359.
67. Park W, Cho S, Ji J, Lewandowski RJ, Larson AC, Kim DH. Development and validation of sorafenib-eluting microspheres to enhance therapeutic efficacy of transcatheter arterial chemoembolization in a rat model of hepatocellular carcinoma. Radiol Imaging Cancer. 2021; 3:e200006.
68. Park W, Kim SJ, Cheresh P, Yun J, Lee B, Kamp DW, et al. Magneto mitochondrial dysfunction mediated cancer cell death using intracellular magnetic nano-transducers. Biomater Sci. 2021; 9:5497–5507.
69. Lencioni R, Cioni D, Crocetti L, Bartolozzi C. Percutaneous ablation of hepatocellular carcinoma: state-of-the-art. Liver Transpl. 2004; 10(2 Suppl 1):S91–S97.
70. Adusumilli PS, Zauderer MG, Rusch VW, O'Cearbhaill RE, Zhu A, Ngai DA, et al. A phase I clinical trial of malignant pleural disease treated with regionally delivered autologous mesothelin-targeted CAR T cells: Safety and efficacy. Cancer Res. 2019; 79(Suppl 13):CT036.
71. Ishikawa W, Kikuchi S, Ogawa T, Tabuchi M, Tazawa H, Kuroda S, et al. boosting replication and penetration of oncolytic adenovirus by paclitaxel eradicate peritoneal metastasis of gastric cancer. Mol Ther Oncolytics. 2020; 18:262–271.
Figure 1.
Combination of IO local therapy and ICI immunotherapy of HCC. Combination of IO local therapies and locally delivered ICI immunotherapy enhances local immunogenicity, releasing tumor antigen and inducing immunogenic cell death. Modulated immunity in HCC effectively unleash the suppressed anti-cancer immune responses with circulating educated CTLs. IO, interventional oncology; ICI, immune checkpoint inhibitor; HCC, hepatocellular carcinoma; CTL, cytotoxic lymphocyte; APC, antigen presenting cell; IL-2, interleukin-2; TRAE, treatment related-adverse effect.
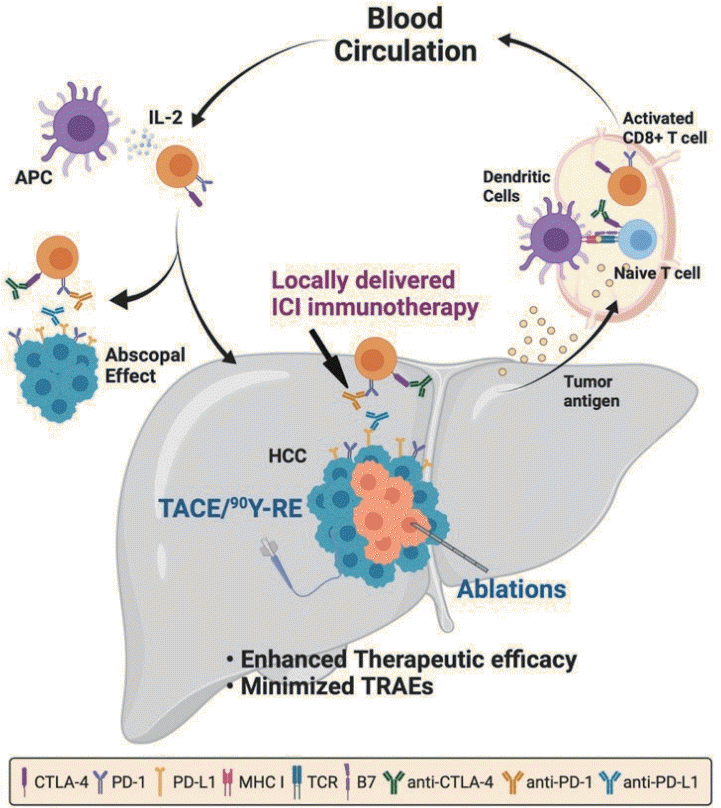
Table 1.
Food and Drug Administration approved immune checkpoint inhibitors and clinical trials on HCC
Table 2.
Clinical trials of combination interventional oncology local therapy and immune checkpoint inhibitor immunotherapy