Abstract
Background
Methods
Results
Supplementary Information
Notes
Ethics Statement
This study was approved by the Institutional Review Board of Seoul National University Bundang Hospital (Seongnam, Korea) (approval No. B-2107-697-101), and the need for informed consent was waived.
Availability of Data and Material
The datasets generated or analyzed during the study are available from the corresponding author on reasonable request.
Author Contributions
Conceptualization: JL, YBH, JHC. Data curation: JL, SKL. Formal analysis: JL, YBH, JHC. Project administration: YBH, JHC. Supervision: HJK, HK, JHC. Writing—original draft: JL, YBH, JHC. Writing—review & editing: JL, YBH, HJK, HK, JHC. Approval of final manuscript: all authors.
References



























Fig. 1
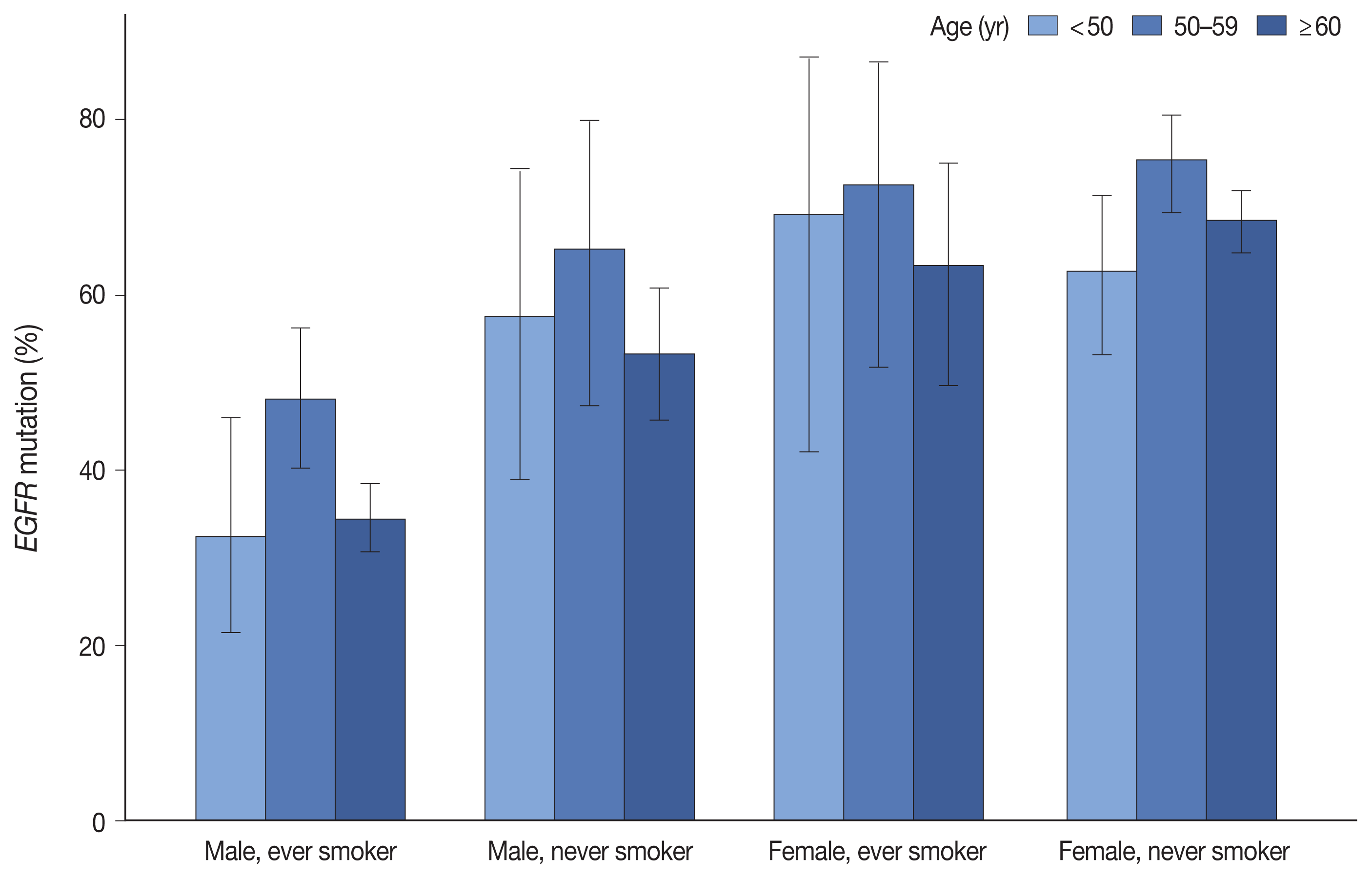
Fig. 2
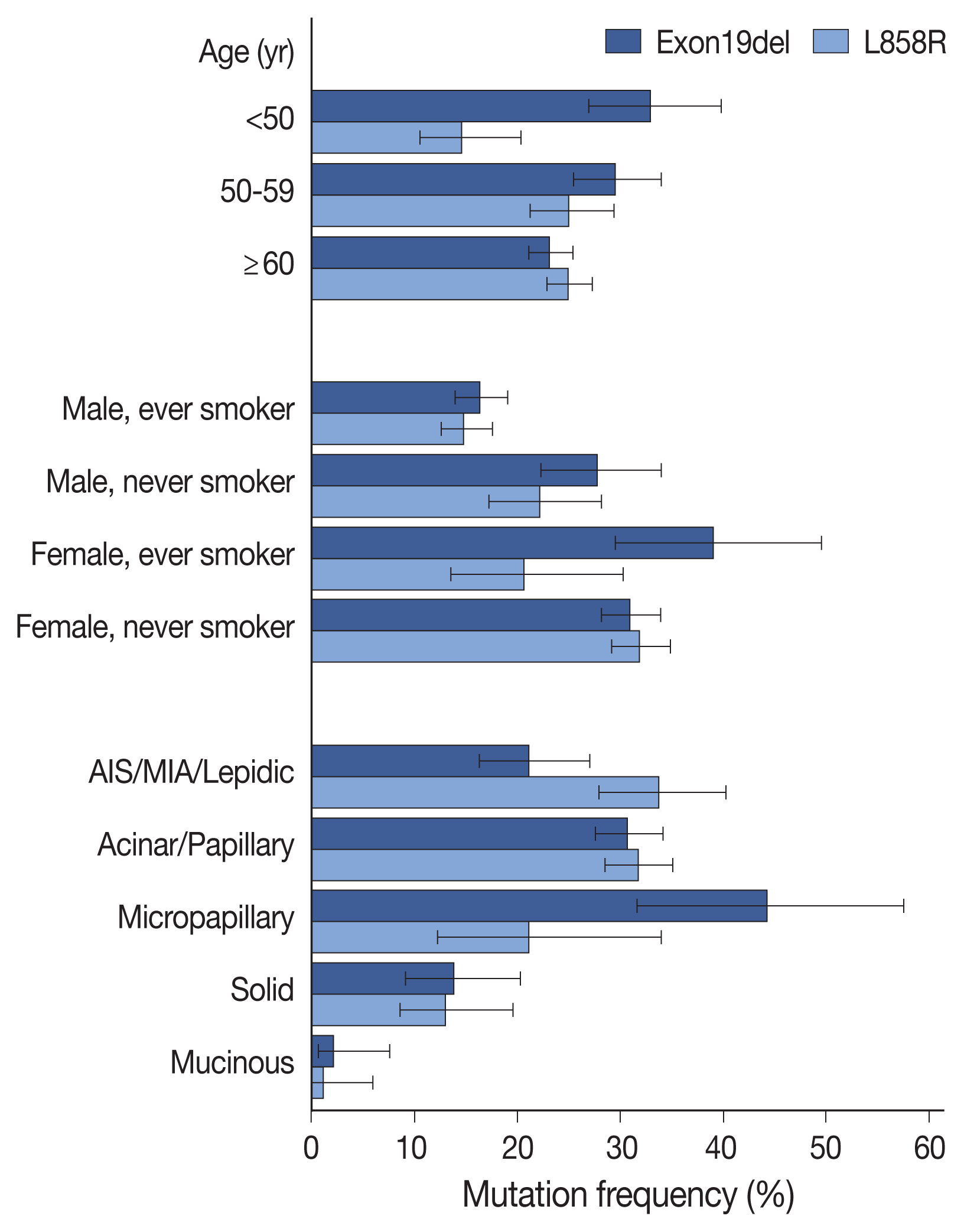
Fig. 3
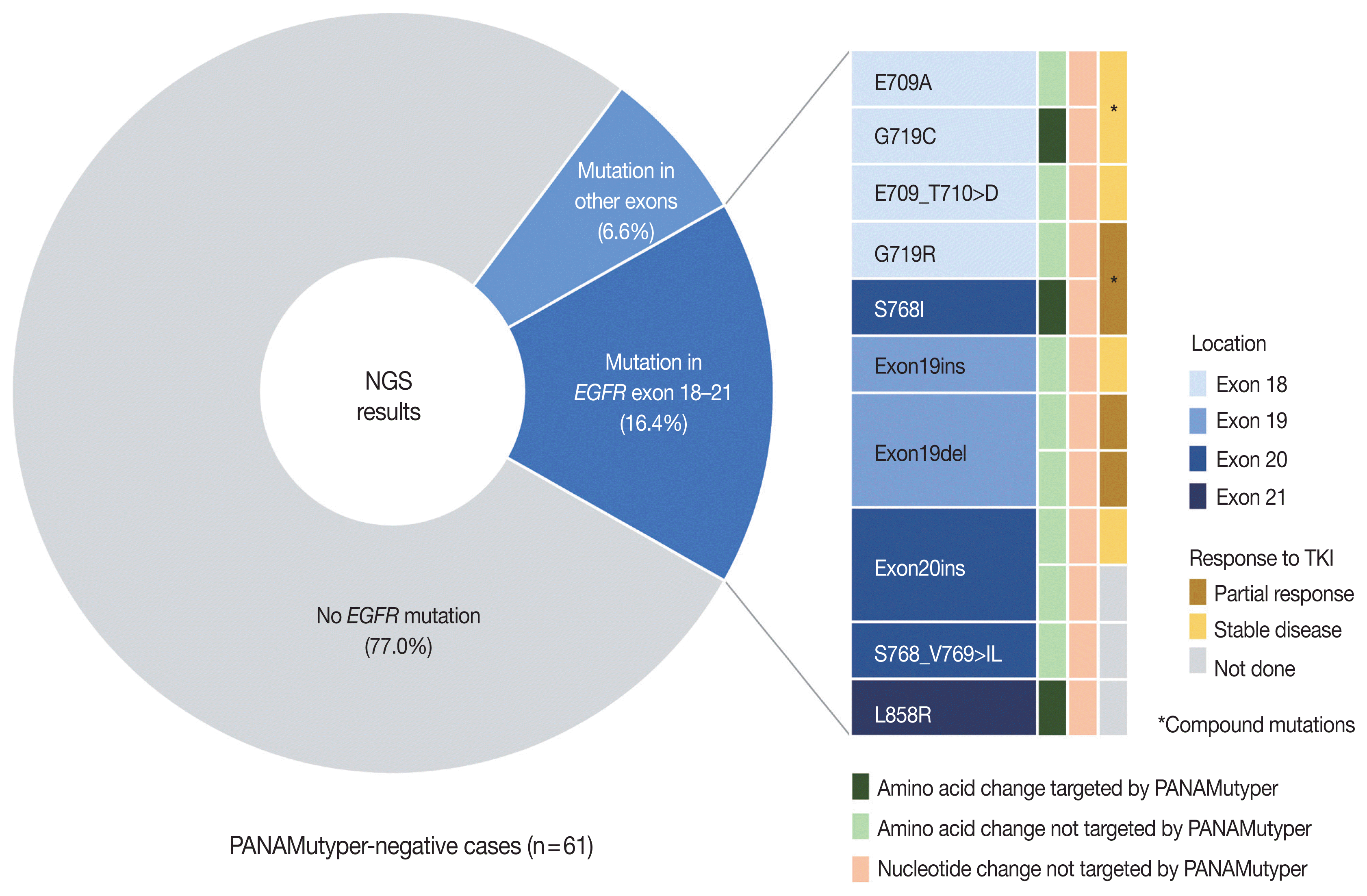
Table 1
Characteristic | Examined No. | EGFR mutation | p-value |
---|---|---|---|
Total | 2,088 (100) | 1,162 (55.7) | |
Age (yr) | |||
< 40 | 36 (1.7) | 17 (47.2) | .001 |
40–49 | 161 (7.7) | 90 (55.9) | |
50–59 | 427 (20.5) | 277 (64.9) | |
60–69 | 673 (32.2) | 370 (55.0) | |
70–79 | 638 (30.6) | 334 (52.4) | |
≥ 80 | 153 (7.3) | 74 (48.4) | |
Sex | |||
Male | 1,005 (48.1) | 412 (41.0) | < .001 |
Female | 1,083 (51.9) | 750 (69.3) | |
Smoking statusa | |||
Never | 1,205 (57.7) | 808 (67.1) | < .001 |
Ever | 873 (41.8) | 349 (40.0) | |
Sex and smoking statusa | |||
Male, never smoker | 216 (10.3) | 120 (55.6) | < .001 |
Male, ever smoker | 786 (37.6) | 291 (37.0) | |
Female, never smoker | 989 (47.4) | 688 (69.6) | |
Female, ever smoker | 87 (4.2) | 58 (66.7) | |
Specimen type | |||
Resection | 1,300 (62.3) | 773 (59.5) | < .001 |
Primary lesion | 1,261 (60.4) | 746 (59.2) | |
Metastatic lesion | 39 (1.9) | 27 (69.2) | |
Biopsy | 776 (37.2) | 385 (49.6) | |
Primary lesion | 463 (22.2) | 241 (52.1) | |
PCNB | 345 (16.5) | 179 (51.9) | |
Bronchoscopic biopsy | 117 (5.6) | 62 (53.0) | |
Thoracoscopic biopsy | 1 (0) | 0 | |
Metastatic lesion | 313 (15.0) | 144 (46.0) | |
PCNB (other than LN) | 52 (2.5) | 29 (55.8) | |
PCNB (LN) | 75 (3.6) | 32 (42.7) | |
EBUS-TBNA biopsy (mediastinal LN) | 154 (7.4) | 64 (41.6) | |
Thoracoscopic biopsy | 29 (1.4) | 18 (62.1) | |
Other biopsies | 3 (0.1) | 1 (33.3) | |
Cytology | 12 (0.6) | 4 (33.3) | |
EBUS-TBNA | 4 (0.2) | 0 | |
Pleural fluid | 8 (0.4) | 4 (50.0) | |
Histologic subtypeb | |||
Adenocarcinoma in situ | 16 (1.3) | 5 (31.3) | < .001 |
Minimally invasive adenocarcinoma | 145 (11.5) | 92 (63.4) | |
Lepidic adenocarcinoma | 55 (4.4) | 36 (65.5) | |
Acinar adenocarcinoma | 404 (32.0) | 279 (69.1) | |
Papillary adenocarcinoma | 348 (27.6) | 244 (70.1) | |
Micropapillary adenocarcinoma | 52 (4.1) | 37 (71.2) | |
Solid adenocarcinoma | 145 (11.5) | 48 (33.1) | |
Invasive mucinous adenocarcinomac | 91 (7.2) | 5 (5.5) | |
Colloid adenocarcinoma | 2 (0.2) | 0 | |
Enteric-type adenocarcinoma | 3 (0.2) | 0 |
Table 2
Total occurrence | Positive rate (%) | Relative proportion (%) | Form of mutation, n (%) | ||
---|---|---|---|---|---|
|
|||||
Singlet | Compound | ||||
G719X | 49 | 2.3 | 4.2 | 31 (63.3) | 18 (36.7) |
Exon19del | 530 | 25.4 | 45.7 | 521 (98.3) | 9 (1.7) |
T790Ma | 12 | 0.6 | 1.0 | 3 (25.0) | 9 (75.0) |
S768I | 12 | 0.6 | 1.0 | 3 (25.0) | 9 (75.0) |
Exon20ins | 70 | 3.4 | 6.0 | 64 (91.4) | 6 (8.6) |
L858R | 502 | 24.0 | 43.3 | 487 (97.0) | 15 (3.0) |
L861Q | 19 | 0.9 | 1.6 | 15 (78.9) | 4 (21.1) |
Table 3
Case | Sex | Age (yr) | PANA results | NGS results | PANA-NGS samples | Sample type (PANA vs. NGS) | TKI treatment | Best response (TTD, mo) | |||
---|---|---|---|---|---|---|---|---|---|---|---|
|
|||||||||||
Exon | Nucleotide change | Amino acid change | VAF (%) | ||||||||
N01 | M | 55 | Not identified |
18 18 |
c.2126A > C c.2154_2155delGGinsTT |
p.E709A p.G719C |
32.1 31.0 |
Same | Lung, PCNB | Afatinib | SD (32) |
N02 | M | 55 | Not identified | 18 | c.2127_2129delAAC | p.E709_T710delinsD | 68.5 | Different | Lung, lobectomy (different block) | Afatinib | SD (15) |
N03 | M | 54 | Not identified |
18 20 |
c.2155G > C c.2303_2304delGCinsTT |
p.G719R p.S768I |
27.5 33.7 |
Same | Lung, PCNB |
Erlotinib Afatinib Osimertinib |
PR (11) SD (7) PD (ND, 2) |
N04 | M | 68 | Not identified | 19 | c.2214_2231dupTAAAATTCCCGTCGCTAT | p.I744_K745insKIPVAI | 33.7 | Different | Lung, lobectomy (different block) | Erlotinib | SD (24) |
N05 | F | 34 | Not identified | 19 | c.2239_2253delTTAAGAGAAGCAACAinsAAC | p.L747_T751delinsN | 47.8 | Different | Lung, lobectomy (different block) | Erlotinib | PR (NR, 24+) |
N06 | F | 50 | Not identified |
19 26 |
c.2253_2276delATCTCCGAAAGCCAACAAGGAAATinsTTCCGC c.3143C > T |
p.P753_I759delinsA p.A1048V |
10.8 50.0 |
Same | LN, PCNB | Erlotinib | PR (4) |
N07 | F | 59 | Not identified | 20 | c.2284-5_2290dupTCCAGGAAGCCT | p.A763_Y764insFQEA | 20.2 | Same | LN, PCNB | Amivantamab | SD (NR, 9+) |
N08 | M | 67 | Not identified |
20 26 |
c.2303_2305delGCGinsTCT c.3143C > T |
p.S768_V769 > IL p.A1048V |
51.7 60.7 |
Same | Lung, PCNB | Erlotiniba | PR (22)a |
N09 | F | 71 | Not identified | 20 | c.2311_2319dupAACCCCCAC | p.N771_H773dup | 13.0 | Same | Lung, PCNB | Not done | - |
N10 | M | 54 | Not identified | 21 | c.2573_2574delTGinsGC | p.L858R | 16.5 | Different | Peritoneum, excision (different lesion) | Not done | - |
N11 | F | 75 | Not identified | 4 | c.500T > C | p.I167T | 3.8 | Same | Lung, PCNB | Not done | - |
N12 | M | 68 | Not identified | 11 | c.1282G > A | p.G428S | 2.3 | Same | Lung, PCNB | Not done | - |
N13 | M | 55 | Not identified | 26 | c.3143C > T | p.A1048V | 44.0 | Different | LN, EBUS-TBNA Bx (different node) | Not done | - |
N14 | F | 49 | Not identified | 26 | c.3143C > T | p.A1048V | 49.9 | Same | Lung, PCNB | Not done | - |
N15 | F | 58 | G719X |
18 18 |
c.2125G > A c.2156G > C |
p.E709K p.G719A |
42.2 41.0 |
Same | LN, PCNB |
Erlotiniba Afatinib |
SD (6)a N/A (ND, 4) |
N16 | F | 36 | Exon19del |
18 19 |
c.2170G > A c.2237_2255delAATTAAGAGAAGCAACATCinsT |
p.G724S p.E746_S752delinsV |
9.8 32.7 |
Same | Pleura, VATS biopsy |
Gefitiniba Erlotinib |
SD (12.5)a SD (9) |
N17 | M | 66 | Exon19del | 17 | c.1996C > T | p.L666F | 45.9 | Same | Lung, PCNB | Erlotinib | SD (ND, 2) |
N18 | F | 50 | Exon19del | Not identified | Different | LN, EBUS-TBNA Bx vs. pleural fluid |
Erlotiniba Erlotinib |
PD (1)a PD (1) |
EGFR, epidermal growth factor receptor; NGS, next-generation sequencing; PANA, PANAMutyper; VAF, variant allele frequency; TKI, tyrosine kinase inhibitor; TTD, time-to-treatment discontinuation; M, male; F, female; PCNB, percutaneous needle biopsy; SD, stable disease; PR, partial response; PD, progressive disease; ND, not detected; NR, not reached; LN, lymph node; EBUS-TBNA, endobronchial ultrasound-transbronchial needle aspiration; Bx, biopsy; N/A, not available; VATS, video-assisted thoracoscopic surgery.