Abstract
Parathyroidectomy is the treatment of choice for primary hyperparathyroidism when the clinical criteria are met. Although bilateral neck exploration is traditionally the standard method for surgery, minimally invasive parathyroidectomy (MIP), or focused parathyroidectomy, has been widely accepted with comparable curative outcomes. For successful MIP, accurate preoperative localization of parathyroid lesions is essential. However, no consensus exists on the optimal approach for localization. Currently, ultrasonography and technetium-99m-sestamibi–single photon emission computed tomography/computed tomography are widely accepted in most cases. However, exact localization cannot always be achieved, especially in cases with multiglandular disease, ectopic glands, recurrent disease, and normocalcemic primary hyperparathyroidism. Therefore, new modalities for preoperative localization have been developed and evaluated. Positron emission tomography/computed tomography and parathyroid venous sampling have demonstrated improvements in sensitivity and accuracy. Both anatomical and functional information can be obtained by combining these methods. As each approach has its advantages and disadvantages, the localization study should be deliberately chosen based on each patient’s clinical profile, costs, radiation exposure, and the availability of experienced experts. In this review, we summarize various methods for the localization of hyperfunctioning parathyroid tissues in primary hyperparathyroidism.
Primary hyperparathyroidism (PHPT) is a status of normal or elevated parathyroid hormone (PTH) levels despite hypercalcemia [1]. The diagnosis is mostly confirmed by biochemical tests, and the clinical indications for surgery are relatively well established [1]. Surgical resection of the parathyroid lesion is indicated when serum calcium is >1 mg/dL above the upper limit of normal, skeletal or renal involvement is present, or the patient’s age is under 50 years [1]. Imaging findings of the parathyroid glands are not required to diagnose PHPT or to decide on a treatment plan. Negative imaging does not indicate that there is no need for surgery. However, once a patient is selected as a surgical candidate, imaging is necessary for performing minimally invasive parathyroidectomy (MIP).
Detecting hyperfunctioning parathyroid tissues is not always easy, and there was a dictum that the only localizing study necessary is to locate an experienced parathyroid surgeon [2]. The conventional surgical exploration involves the surgeon exploring the bilateral neck area, finding the pathologic parathyroid glands, and removing them [3]. However, MIP has been widely accepted as most patients with PHPT have a single parathyroid adenoma [4,5]. MIP has a lower risk of complications, shorter operation time, more rapid recovery, and more favorable cosmetic results than conventional bilateral neck exploration [3,5,6]. Preoperative localization is an integral part of performing MIP because surgeons need a direct approach to the pathologic parathyroid gland. Moreover, when the disease is persistent or recurrent and reoperation is planned, positive preoperative imaging is essential in planning reoperative parathyroidectomy, as the abnormal glands may be in ectopic locations [7,8].
Over the past few decades, advanced modalities for parathyroid localization have been developed. However, no consensus exists regarding the optimal localization procedure and imaging protocol, and the clinical approaches vary depending on local expertise and institutional factors [9]. In this review article, we summarize the modalities for parathyroid localization and clinical considerations for their employment.
Ultrasonography is widely used to detect pathologic parathyroid tissue [8,10,11]. Normal parathyroid glands are about 4 mm in size and are usually not visualized on ultrasonography [11]. However, parathyroid adenomas are larger than normal parathyroid glands and appear as round or oval well-defined hypoechogenic structures [10,11]. Larger parathyroid adenomas may show cystic changes, calcifications, and lobulations [10,11]. Ultrasonography is useful for detecting parathyroid adenomas located near the thyroid gland or the upper cervical portion of the thymus. However, ultrasonography often cannot detect parathyroid adenomas located behind the trachea or esophagus, or ectopic lesions [10]. Ectopic parathyroid regions, ranging from the carotid bifurcation to the sternal notch and the carotid artery, are strongly recommended to be included in the ultrasound field [12,13]. The sensitivity of ultrasonography varies depending on the location of the parathyroid lesion; its overall sensitivity was reported to be 55% to 87%, and it is especially low in cases with ectopic parathyroid tissues or normocalcemic PHPT [11,14,15]. The positive predictive value of ultrasonography ranges from 93% to 97%, and its specificity ranges from 40% to 98% [14].
The advantages of ultrasonography include a lack of radiation exposure, low cost, convenience, and the ability to screen for concomitant thyroid gland pathology. However, operator-dependent results and its low sensitivity for ectopic parathyroid tissue, small parathyroid adenomas, and intra-thyroidal masses are limitations of this modality. False-positive results due to thyroid nodules and enlarged lymph nodes also should be taken into account [11].
Ultrasound-guided fine needle aspiration biopsy (FNAB) with intracystic PTH measurement might be considered in difficult cases. This is a highly specific method for parathyroid localization [16-18]. However, the risk of parathyromatosis, hematoma, abscess, and inflammation is a barrier to its widespread use [19]. In particular, parathyroid FNAB should not be performed when parathyroid cancer is suspected because of the risk of seeding or dissemination of parathyroid tissue [8]. In addition, parathyroid FNAB cannot reliably distinguish between benign and malignant parathyroid lesions [8]. Given the aforementioned risks, parathyroid FNAB should be limited to carefully selected patients [8,16].
Parathyroid scintigraphy has been employed to detect parathyroid lesions since 57Co-cyanocobalamine and 75Se-selenomethionine were first used [20,21]. However, due to their poor image quality, high radiation dose, and low sensitivity, the clinical use of those radiotracers was abandoned with suboptimal results [22]. Several new radioisotopes, such as technetium-99m (99mTc), were proposed and drew attention because radionuclide imaging has the advantage of being able to identify the functional status of parathyroid tissue [23,24]. When combined with single photon emission computed tomography/computed tomography (SPECT/CT), radionuclide imaging can provide information on both anatomical structures and functional activity [25].
Positron emission tomography/CT (PET/CT) is a molecular imaging technique that has high sensitivity and spatial resolution [26]. Several PET tracers have been utilized in parathyroid imaging, including 18F-fluorodeoxyglucose (18F-FDG), 11C-methionine, 11C-choline, and 18F-fluorocholine. In this section, we describe several radionuclide imaging methods that are currently used to localize parathyroid lesions.
In 1989, 99mTc-sestamibi scintigraphy was first described as a radiopharmaceutical for detecting parathyroid lesions by Coakley et al. [24], and now it is a dominant isotope used for parathyroid scintigraphy [8]. 99mTc-sestamibi is a lipophilic cationic radiotracer, and its uptake depends on plasma and mitochondrial membrane potentials. Since adenomatous and hyperplastic parathyroid tissue has a large number of mitochondria in oxyphil cells, 99mTc-sestamibi uptake is avid and the washout is slow [25]. In contrast, uptake clears more rapidly in thyroid tissue than in parathyroid tissue [27]. Additionally, P-glycoprotein expression is associated with the transport of 99mTc-sestamibi across the cell membrane [28,29]. A transmembrane protein, P-glycoprotein is an energy-dependent influx and efflux pump [30]. Abnormal parathyroid tissues have lower levels of P-glycoprotein expression than normal parathyroid glands, leading to greater sestamibi retention [30].
Sestamibi scintigraphy is widely available and relatively inexpensive. Moreover, it has a wide imaging field which allows the detection of ectopic glands [22]. However, thyroid nodules, inflammatory thyroiditis, and lymphadenopathy might result in false-positive scans [31,32], and false-negative imaging results can occur for parathyroid adenomas weighing less than 600 to 800 mg [33]. The sensitivity of 99mTc-sestamibi scans was reported to range widely from 60% to 90% due to variations in study protocols and disease characteristics [34,35]. The sensitivity markedly decreased (30% to 45%) in patients with parathyroid hyperplasia or multiple adenomas [36].
To improve the sensitivity and obtain additional anatomical information, a protocol that combined 99mTc-sestamibi and SPECT/CT was developed, and its diagnostic value was validated [37-39]. SPECT/CT is a combination of SPECT with CT, and the addition of SPECT/CT to a 99mTc-sestamibi scan improves sensitivity [38]. It not only provides anatomical information but also makes it possible to differentiate parathyroid lesions from other sources of 99mTc-sestamibi uptake, including thyroid nodules and cervical lymph nodes [40]. In a meta-analysis by Wong et al. [41], 99mTc-sestamibi-SPECT/CT showed an estimated pooled sensitivity of 86% (95% confidence interval [CI], 81% to 90%), which was superior to that of planar imaging (70%; 95% CI, 61% to 80%) and SPECT (74%; 95% CI, 66% to 82%). However, its sensitivity is unsatisfactory in specific situations such as multiglandular disease and ectopic adenoma [42,43]. According to the study by Tay et al. [44], the sensitivity of 99mTc-sestamibi SPECT/CT was 78% in patients with single-glandular disease, whereas it was only 31% in multiglandular disease. Moreover, the detection rate of 99mTc-sestamibi might be limited in cases with low PTH levels or normocalcemic PHPT [15,45]. However, it is currently used as a first-line imaging modality despite the several limitations mentioned above [46].
11C-methionine accumulates in abnormal tissue of the parathyroid gland, making it a promising radiopharmaceutical in parathyroid imaging [47]. Although the exact mechanism of 11C-methionine uptake by parathyroid glands is not fully understood, 11C-methionine might be involved in the synthesis of pre-pro-PTH [48]. The clinical use of 11C-methionine-PET (MET-PET) for parathyroid disease was first described in 1994 [49], and the evolution from PET to PET-CT led to more accurate identification and localization of parathyroid lesions [47]. MET-PET/CT has been reported to show a comparable sensitivity to that of other traditional scintigraphy methods [50]. The per-patient sensitivity of MET-PET/CT and 99mTc-sestamibi-SPECT/CT was 65% and 61%, respectively [50]. The per-lesion sensitivity of MET-PET/CT and 99mTc-sestamibi-SPECT/CT was 91% and 73% for parathyroid adenomas, without a statistically significant difference [50]. However, Weber et al. [51] reported that the sensitivity of MET-PET/CT was markedly lower in multiglandular disease (67%) than in detecting a single parathyroid adenoma (83%). The lower sensitivity for multiglandular disease was probably because the hyperplastic glands had less PTH synthesis and lower uptake of 11C-methionine than parathyroid adenomas [52]. Another limitation is the short half-life of 11C, leading to limited availability of the 11C-methionine tracer, its high cost, and a substantial workload for preparation [47,53,54].
Radiolabeled choline (11C-choline or 18F-choline) has recently been explored and used as a promising PET tracer for detecting hyperfunctioning parathyroid tissue. Choline uptake is increased by choline kinase upregulation, and phospholipid-dependent choline kinase is upregulated where PTH is oversecreted. Based on this, radiolabeled choline PET can be used to detect parathyroid lesions [55,56]. 18F-choline PET has wider availability than 11C-choline PET because it has a long half-life; thus, it does not need an on-site cyclotron [55].
Compared to MET-PET/CT, 18F-choline PET/CT was reported to be more sensitive for parathyroid localization in patients with PHPT who had negative or inconclusive 99mTc-sestamibi-SPECT [57]. The per-patient sensitivity of 18F-choline PET/CT and MET-PET/CT was 96% and 60%, respectively [57]. Moreover, 18F-choline PET/CT showed comparable or superior sensitivity compared to 99mTc-sestamibi-SPECT/CT [42,58]. In a study by Araz et al. [58], the sensitivity of 18F-choline PET/CT and 99mTc-sestamibi SPECT/CT was 96% and 78%, respectively.
Other advantages of 18F-choline PET/CT include a shorter imaging time [42], higher spatial resolution [59], and a lower radiation dose (2.8 mSv) than 99mTc-sestamibi SPECT/CT (6.8 mSv) [60-62]. Despite its superior diagnostic performance compared to conventional imaging in detecting parathyroid lesions, its use is still limited due to its high cost and low availability [61].
18F-FDG is the most widely available PET tracer. It reflects metabolic activity by measuring the accumulation of FDG, which is an analog of glucose [63]. 18F-FDG PET is used to detect malignancy, monitor treatment response, and predict the disease prognosis [63]. As with other tumors, 18F-FDG PET can be employed for parathyroid cancer [64,65]. A case report by Neumann et al. [65] showed that 18F-FDG PET could localize recurrent postoperative parathyroid cancer cases that 99mTc-sestamibi scintigraphy and magnetic resonance imaging failed to detect. Several studies have investigated the role of 18F-FDG PET in parathyroid adenoma based on the hypothesis that FDG would accumulate in parathyroid adenoma in a sufficient amount to be visualized on PET images [66-68]. In the 1990s, the Neumann et al. [66] reported that 18F-FDG PET showed superior sensitivity compared to 99mTc-sestamibi-SPECT, and suggested 18F-FDG PET as a promising tool for preoperative localization in patients with PHPT [67]. In contrast, Melon et al. [68] concluded that the sensitivity of 18F-FDG-PET is too low for preoperative parathyroid detection in PHPT. At present, 18F-FDG-PET is not widely utilized in parathyroid adenoma patients. The possible false-positive results due to inflammation should be considered [69,70].
With the advent of radiolabeled peptides, somatostatin receptor PET imaging, such as gallium68 (68Ga)- 1,4,7,10-tetraazacyclododecane-1,4,7,10-tetraacetic acid (DOTA)-D-Phe1-Tyr3–octreotide (TOC) PET, 68Ga-DOTA-1-Nal3-octreotide PET, and 68Ga-DOTA-D-Phe1-Tyr3-octreotate PET has emerged for detecting neuroendocrine tumors [71]. In neuroendocrine tumors, the lesion identification rate of 68Ga-DOTA-TOC PET was higher than that of somatostatin receptor scintigraphy [72-74]. It was hypothesized that somatostatin receptor PET imaging might be utilized in parathyroid adenomas that express surface somatostatin receptors [75]. However, in a study by Froeling et al. [76], MEN-associated parathyroid adenomas were not detected by 68Ga-DOTA-TOC PET and were identified by only CT. To date, somatostatin receptor PET imaging is not generally recommended as an ideal imaging modality for parathyroid adenomas [75,76].
Conventional CT is usually not used for parathyroid localization due to its inferiority to other modalities [22]. Parathyroid four-dimensional-CT (4D-CT) was first recognized as a tool for localization in 2006 by Rodgers et al. [77]. The name of 4D-CT is derived from the fact that a dimension from the changes in contrast perfusion over time is added to three-dimensional CT [77]. A 4D-CT examination consists of pre-contrast, post-contrast, and delayed phases. In the pre-contrast image, parathyroid adenomas have similar attenuation as the surrounding muscles and are distinguished from the iodine-rich dense thyroid gland [11]. In early and delayed post-contrast images, parathyroid adenomas are seen as hypervascular tissue with variable enhancement and rapid washout.
In previous studies, 4D-CT demonstrated better sensitivity than 99mTc-sestamibi scans and ultrasonography [78,79]. In a study by Starker et al. [78], 4D-CT showed higher sensitivity (85.7%) than 99mTc-sestamibi-SPECT (40.4%) and ultrasonography (48%). It also showed superior sensitivity in reoperative cases [79]. In patients who had previous neck surgery, 4D-CT had a sensitivity of 88%, which was superior to 99mTc-sestamibi (54%) or ultrasonography (21%) [79]. Moreover, 4D-CT can be useful in patients with multiglandular disease or ectopic glands, although multiglandular disease remains a challenging clinical entity even with 4D-CT [79-81]. The main drawback of 4D-CT is the high radiation dose to the patient despite the use of dose reduction techniques [82,83]. A high false-positive rate, difficult interpretation, and low availability are also limitations of this modality [22]. Still, when ultrasonography and 99mTc-sestamibi scans are negative and SPECT/CT is not available, 4D-CT might be a beneficial method both in primary and reoperative cases.
Selective parathyroid venous sampling (PVS) can be a useful technique when localization is inconclusive with the noninvasive tests described above or when the disease recurs after the first operation [22,84]. Venous access is usually acquired via the femoral vein, and blood samples are obtained from the superior vena cava, bilateral brachiocephalic, internal jugular, vertebral, thymic, and superior, middle, and inferior thyroid veins [22]. Although a 2-fold higher PTH level than the peripheral level was conventionally used as a cutoff value [85,86], the optimal cutoff has not been fully validated. In a recent study, a 1.5-fold elevation of PTH was suggested as an optimal cutoff that improved discriminative performance [87]. The sensitivity of PVS has been reported to range from 71% to 90% due to the significant heterogeneity of enrolled patients and methodology [84,88-90]. In cases of persistent or recurrent PHPT, PVS showed significantly higher sensitivity than 99mTc-sestamibi-SPECT, which were 75% versus 30%, respectively [91]. PVS also demonstrated a high concordance rate (94.1%) with the pathological examination in cases with negative or inconsistent imaging tests [92].
One of the main concerns regarding PVS is its invasiveness. Bleeding, infection, arteriovenous fistulae, and pseudoaneurysms are possible complications of PVS, although they rarely occur [92]. Radiation exposure during PVS ranges from 1.26 to 5.3 mSv, which is approximately half the dose of 4D-CT (10.4 mSv) [93,94]. Other drawbacks of PVS include its relatively high cost and the need for experienced radiologists [10].
Parathyroid glands are small and their location varies widely. Therefore, distinguishing parathyroid glands from the surrounding tissue is often difficult during neck surgery. This task is particularly difficult when the pathogenic glands are smaller and multiglandular, as is often observed in normocalcemic PHPT [95,96]. Frozen section analysis and intraoperative PTH assays are traditional methods of parathyroid tissue confirmation [8]. However, the confirmation cannot be made before the gland is surgically resected. Moreover, these methods are invasive or require a longer operative time [97].
A gamma probe is a handheld gamma detector that can be utilized intraoperatively after radionuclide injection [98]. It was once speculated that using a gamma probe after preoperative 99mTc-sestamibi injection would not be beneficial in cases with a negative 99mTc-sestamibi scan [99]. However, Buicko et al. [99] reported that a gamma probe showed high sensitivity (90.5%) in identifying parathyroid adenoma in patients with negative preoperative 99mTc-sestamibi scans. Moreover, the gamma probe was effective in cases with multiple or ectopic parathyroid adenomas [99-101]. Jaskowiak et al. [102] reported two reoperative cases with dense scars and obscured anatomy in which a gamma probe provided crucial aid in localization. However, the utility of gamma probes remains limited to an adjunctive role, and it cannot replace preoperative imaging or localization modalities [103].
As another intraoperative localization technique, fluorescence imaging has been proposed, as it is a real-time, accurate and rapid technique that can be used to identify parathyroid glands before they are resected [97,104]. It can be employed both in parathyroidectomy and thyroidectomy, so that postsurgical hypoparathyroidism can be avoided [97]. The parathyroid gland shows a unique pattern of autofluorescence and is displayed in the blue color channel when exposed to near-infrared light [104]. The intensity of fluorescence appears greater in parathyroid tissue than in other surrounding tissues such as the thyroid, lymph nodes, and adipose tissue [104]. Methylene blue and aminolevulinic acid have been studied as exogenous contrast materials in near-infrared autofluorescence (NIRAF) imaging [105,106].
The use of NIRAF imaging in the localization of hyperfunctioning parathyroid tissue is still limited because the accuracy of NIRAF in distinguishing pathologic from normal parathyroid glands has not been fully validated, with inconsistent reports reported in the literature [107-110]. Some studies reported that adenomas had higher NIRAF intensity than normal parathyroid glands [108], but others reported contradictory findings [107, 109,110]. Pathologic parathyroid glands showed lower NIRAF intensity in other situations [107], or similar intensity compared to normal parathyroid tissue [109,110]. The first report on the NIRAF pattern of parathyroid carcinoma was recently published as a case series, and autofluorescence was absent in three patients with parathyroid cancer [111]. Further studies are warranted to determine whether NIRAF can be used to detect hyperfunctioning parathyroid tissue in patients with PHPT.
In cases when all glands should be exposed, such as multiglandular disease, recurrent PHPT, multiple endocrine neoplasia, and secondary hyperparathyroidism, these intraoperative localization techniques may offer a benefit for detecting the parathyroid glands [104,112]. The routine use of NIRAF or gamma probes is not currently widely accepted.
Although several parathyroid imaging modalities have been developed, no consensus exists regarding their indications and applications, leading to their less efficient usage or underutilization in clinical practice. Thus, we reviewed the current preoperative localization of parathyroid glands in patients with PHPT in this article. Table 1 summarizes the advantages and disadvantages of each preoperative modality, and Fig. 1 depicts the possible preoperative localization process for surgical candidates with PHPT.
A combination of ultrasonography and 99mTc-sestamibi SPECT/CT is currently a favored approach to localize pathologic parathyroid tissue at most institutions. These modalities have fair sensitivity when the etiology is a single adenoma containing an ample amount of mitochondria. However, PHPT may arise from multifocal lesions with various histology, including smaller adenoma, hyperplasia, or cancer. The sensitivity of conventional imaging modalities is low for pathologic parathyroid lesions that are multiglandular or ectopic [113,114]. Parathyroid localization is challenging in patients with previous neck surgery; recurrent, persistent, or normocalcemic hyperparathyroidism; ectopic parathyroid glands; multiglandular disease; or parathyroid hyperplasia [45,114,115].
Choline PET and 4D-CT are imaging tests that might provide superior localization in certain situations. Because previous studies have reported superior sensitivity of choline PET compared to MET-PET [57,116], choline PET could be preferred to MET-PET. PVS is an invasive technique, but it plays an important role in difficult cases such as remedial cases or those with negative imaging tests. However, modalities such as choline PET, MET-PET, 4D-CT, and PVS are not widely available. Therefore, it might be helpful to repeat 99mTc-sestamibi SPECT/CT at a high-volume center when the first scan is negative [117]. Unfortunately, appropriate localization is still clinically challenging in cases with multiglandular disease and parathyroid hyperplasia. New methods, including NIRAF, are being studied and are expected to enable better preoperative localization in the future.
REFERENCES
1. Bilezikian JP, Brandi ML, Eastell R, Silverberg SJ, Udelsman R, Marcocci C, et al. Guidelines for the management of asymptomatic primary hyperparathyroidism: summary statement from the Fourth International Workshop. J Clin Endocrinol Metab. 2014; 99:3561–9.


2. Doppman JL, Miller DL. Localization of parathyroid tumors in patients with asymptomatic hyperparathyroidism and no previous surgery. J Bone Miner Res. 1991; 6 Suppl 2:S153–9.


3. Udelsman R, Lin Z, Donovan P. The superiority of minimally invasive parathyroidectomy based on 1650 consecutive patients with primary hyperparathyroidism. Ann Surg. 2011; 253:585–91.


4. Venkat R, Kouniavsky G, Tufano RP, Schneider EB, Dackiw AP, Zeiger MA. Long-term outcome in patients with primary hyperparathyroidism who underwent minimally invasive parathyroidectomy. World J Surg. 2012; 36:55–60.


5. Kunstman JW, Udelsman R. Superiority of minimally invasive parathyroidectomy. Adv Surg. 2012; 46:171–89.


6. Greene AB, Butler RS, McIntyre S, Barbosa GF, Mitchell J, Berber E, et al. National trends in parathyroid surgery from 1998 to 2008: a decade of change. J Am Coll Surg. 2009; 209:332–43.


8. Wilhelm SM, Wang TS, Ruan DT, Lee JA, Asa SL, Duh QY, et al. The American Association of Endocrine Surgeons guidelines for definitive management of primary hyperparathyroidism. JAMA Surg. 2016; 151:959–68.


9. Silva BC, Cusano NE, Bilezikian JP. Primary hyperparathyroidism. Best Pract Res Clin Endocrinol Metab. 2018; 32:593–607.


10. Khan AA, Hanley DA, Rizzoli R, Bollerslev J, Young JE, Rejnmark L, et al. Primary hyperparathyroidism: review and recommendations on evaluation, diagnosis, and management: a Canadian and international consensus. Osteoporos Int. 2017; 28:1–19.


11. Siraj QH. Radionuclide parathyroid imaging. Cham: Springer;2020. Chapter 4, Diagnostic imaging: structural modalities. 31–40.


12. Leupe PK, Delaere PR, Vander Poorten VL, Debruyne F. Pre-operative imaging in primary hyperparathyroidism with ultrasonography and sestamibi scintigraphy. B-ENT. 2011; 7:173–80.
14. Cheung K, Wang TS, Farrokhyar F, Roman SA, Sosa JA. A meta-analysis of preoperative localization techniques for patients with primary hyperparathyroidism. Ann Surg Oncol. 2012; 19:577–83.


15. Cunha-Bezerra P, Vieira R, Amaral F, Cartaxo H, Lima T, Montarroyos U, et al. Better performance of four-dimension computed tomography as a localization procedure in normocalcemic primary hyperparathyroidism. J Med Imaging Radiat Oncol. 2018; 62:493–8.


16. Bancos I, Grant CS, Nadeem S, Stan MN, Reading CC, Sebo TJ, et al. Risks and benefits of parathyroid fine-needle aspiration with parathyroid hormone washout. Endocr Pract. 2012; 18:441–9.


17. MacFarlane MP, Fraker DL, Shawker TH, Norton JA, Doppman JL, Chang RA, et al. Use of preoperative fine-needle aspiration in patients undergoing reoperation for primary hyperparathyroidism. Surgery. 1994; 116:959–65.
18. Stephen AE, Milas M, Garner CN, Wagner KE, Siperstein AE. Use of surgeon-performed office ultrasound and parathyroid fine needle aspiration for complex parathyroid localization. Surgery. 2005; 138:1143–51.


19. Alwaheeb S, Rambaldini G, Boerner S, Coire C, Fiser J, Asa SL. Worrisome histologic alterations following fine-needle aspiration of the parathyroid. J Clin Pathol. 2006; 59:1094–6.


20. Sisson J, Beierwaltes W. Radiocyanocobalamine (Co-57-B-12) concentration in the parathyroid glands. J Nucl Med. 1962; 3:160–6.
21. Potchen EJ, Adelstein SJ, Dealy JB Jr. Radioisotope localization of the overactive human parathyroid. Am J Roentgenol Radium Ther Nucl Med. 1965; 93:955–61.
22. Kunstman JW, Kirsch JD, Mahajan A, Udelsman R. Clinical review: parathyroid localization and implications for clinical management. J Clin Endocrinol Metab. 2013; 98:902–12.
23. Fukunaga M, Fujita T, Yonekura Y, Dokoh S, Yamamoto I, Morita R, et al. Visualization of parathyroid tumor with 201Tl-chloride (author’s transl). Kaku Igaku. 1979; 16:327–31.
24. Coakley AJ, Kettle AG, Wells CP, O’Doherty MJ, Collins RE. 99Tcm sestamibi: a new agent for parathyroid imaging. Nucl Med Commun. 1989; 10:791–4.
25. Siraj QH. Radionuclide parathyroid imaging. Cham: Springer;2020. Chapter 5, Parathyroid Scintigraphy. p. 41–60.


26. Siraj QH. Radionuclide parathyroid imaging. Cham: Springer;2020. Chapter 6, Parathyroid PET. p. 61–5.


27. Kettle AG, O’Doherty MJ. Parathyroid imaging: how good is it and how should it be done? Semin Nucl Med. 2006; 36:206–11.


28. Hendrikse NH, Franssen EJ, van der Graaf WT, Meijer C, Piers DA, Vaalburg W, et al. 99mTc-sestamibi is a substrate for P-glycoprotein and the multidrug resistance-associated protein. Br J Cancer. 1998; 77:353–8.


29. Gupta Y, Ahmed R, Happerfield L, Pinder SE, Balan KK, Wishart GC. P-glycoprotein expression is associated with sestamibi washout in primary hyperparathyroidism. Br J Surg. 2007; 94:1491–5.


30. Mitchell BK, Cornelius EA, Zoghbi S, Murren JR, Ghoussoub R, Flynn SD, et al. Mechanism of technetium 99m sestamibi parathyroid imaging and the possible role of p-glycoprotein. Surgery. 1996; 120:1039–45.


31. Erbil Y, Barbaros U, Yanik BT, Salmaslioglu A, Tunaci M, Adalet I, et al. Impact of gland morphology and concomitant thyroid nodules on preoperative localization of parathyroid adenomas. Laryngoscope. 2006; 116:580–5.


32. Vattimo A, Bertelli P, Cintorino M, Burroni L, Volterrani D, Vella A, et al. Hurthle cell tumor dwelling in hot thyroid nodules: preoperative detection with technetium-99m-MIBI dual-phase scintigraphy. J Nucl Med. 1998; 39:822–5.
33. Erbil Y, Barbaros U, Tukenmez M, Issever H, Salmaslioglu A, Adalet I, et al. Impact of adenoma weight and ectopic location of parathyroid adenoma on localization study results. World J Surg. 2008; 32:566–71.


34. Lavely WC, Goetze S, Friedman KP, Leal JP, Zhang Z, Garret-Mayer E, et al. Comparison of SPECT/CT, SPECT, and planar imaging with single- and dual-phase (99m)Tc-sestamibi parathyroid scintigraphy. J Nucl Med. 2007; 48:1084–9.


36. Ruda JM, Hollenbeak CS, Stack BC Jr. A systematic review of the diagnosis and treatment of primary hyperparathyroidism from 1995 to 2003. Otolaryngol Head Neck Surg. 2005; 132:359–72.


37. Krausz Y, Bettman L, Guralnik L, Yosilevsky G, Keidar Z, Bar-Shalom R, et al. Technetium-99m-MIBI SPECT/CT in primary hyperparathyroidism. World J Surg. 2006; 30:76–83.


38. Neumann DR, Obuchowski NA, Difilippo FP. Preoperative 123I/99mTc-sestamibi subtraction SPECT and SPECT/CT in primary hyperparathyroidism. J Nucl Med. 2008; 49:2012–7.


39. Kim YI, Jung YH, Hwang KT, Lee HY. Efficacy of 99mTc-sestamibi SPECT/CT for minimally invasive parathyroidectomy: comparative study with 99mTc-sestamibi scintigraphy, SPECT, US and CT. Ann Nucl Med. 2012; 26:804–10.


40. Akram K, Parker JA, Donohoe K, Kolodny G. Role of single photon emission computed tomography/computed tomography in localization of ectopic parathyroid adenoma: a pictorial case series and review of the current literature. Clin Nucl Med. 2009; 34:500–2.
41. Wong KK, Fig LM, Gross MD, Dwamena BA. Parathyroid adenoma localization with 99mTc-sestamibi SPECT/CT: a meta-analysis. Nucl Med Commun. 2015; 36:363–75.
42. Beheshti M, Hehenwarter L, Paymani Z, Rendl G, Imamovic L, Rettenbacher R, et al. 18F-fluorocholine PET/CT in the assessment of primary hyperparathyroidism compared with 99mTc-MIBI or 99mTc-tetrofosmin SPECT/CT: a prospective dual-centre study in 100 patients. Eur J Nucl Med Mol Imaging. 2018; 45:1762–71.


43. Cuderman A, Senica K, Rep S, Hocevar M, Kocjan T, Sever MJ, et al. 18F-fluorocholine PET/CT in primary hyperparathyroidism: superior diagnostic performance to conventional scintigraphic imaging for localization of hyperfunctioning parathyroid glands. J Nucl Med. 2020; 61:577–83.


44. Tay D, Das JP, Yeh R. Preoperative localization for primary hyperparathyroidism: a clinical review. Biomedicines. 2021; 9:390.


45. Gomez-Ramirez J, Gomez-Valdazo A, Luengo P, Porrero B, Osorio I, Rivas S. Comparative prospective study on the presentation of normocalcemic primary hyperparathyroidism: is it more aggressive than the hypercalcemic form? Am J Surg. 2020; 219:150–3.


46. Boccalatte LA, Higuera F, Gomez NL, de la Torre AY, Mazzaro EL, Galich AM, et al. Usefulness of 18F-fluorocholine positron emission tomography-computed tomography in locating lesions in hyperparathyroidism: a systematic review. JAMA Otolaryngol Head Neck Surg. 2019; 145:743–50.


47. Saerens J, Velkeniers B, Keyaerts M, Raeymaeckers S, Vanhoeij M, Blotwijk S, et al. Value of [11C]-methionine PET/CT in preoperative localization of parathyroid adenomas. Horm Metab Res. 2021; 53:444–52.


48. Yuan L, Liu J, Kan Y, Yang J, Wang X. The diagnostic value of 11C-methionine PET in hyperparathyroidism with negative 99mTc-MIBI SPECT: a meta-analysis. Acta Radiol. 2017; 58:558–64.


49. Hellman P, Ahlstrom H, Bergstrom M, Sundin A, Langstrom B, Westerberg G, et al. Positron emission tomography with 11C-methionine in hyperparathyroidism. Surgery. 1994; 116:974–81.
50. Hayakawa N, Nakamoto Y, Kurihara K, Yasoda A, Kanamoto N, Miura M, et al. A comparison between 11C-methionine PET/CT and MIBI SPECT/CT for localization of parathyroid adenomas/hyperplasia. Nucl Med Commun. 2015; 36:53–9.


51. Weber T, Cammerer G, Schick C, Solbach C, Hillenbrand A, Barth TF, et al. C-11 methionine positron emission tomography/computed tomography localizes parathyroid adenomas in primary hyperparathyroidism. Horm Metab Res. 2010; 42:209–14.


52. Tang BN, Moreno-Reyes R, Blocklet D, Corvilain B, Cappello M, Delpierre I, et al. Accurate pre-operative localization of pathological parathyroid glands using 11C-methionine PET/CT. Contrast Media Mol Imaging. 2008; 3:157–63.


53. Rubello D, Fanti S, Nanni C, Farsad M, Castellucci P, Boschi S, et al. 11C-methionine PET/CT in 99mTc-sestamibi-negative hyperparathyroidism in patients with renal failure on chronic haemodialysis. Eur J Nucl Med Mol Imaging. 2006; 33:453–9.


54. Caldarella C, Treglia G, Isgro MA, Giordano A. Diagnostic performance of positron emission tomography using 11C-methionine in patients with suspected parathyroid adenoma: a meta-analysis. Endocrine. 2013; 43:78–83.


55. Treglia G, Giovannini E, Di Franco D, Calcagni ML, Rufini V, Picchio M, et al. The role of positron emission tomography using carbon-11 and fluorine-18 choline in tumors other than prostate cancer: a systematic review. Ann Nucl Med. 2012; 26:451–61.


56. Kluijfhout WP, Pasternak JD, Drake FT, Beninato T, Gosnell JE, Shen WT, et al. Use of PET tracers for parathyroid localization: a systematic review and meta-analysis. Langenbecks Arch Surg. 2016; 401:925–35.


57. Mathey C, Keyzer C, Blocklet D, Van Simaeys G, Trotta N, Lacroix S, et al. 18F-fluorocholine PET/CT is more sensitive than 11C-methionine PET/CT for the localization of hyperfunctioning parathyroid tissue in primary hyperparathyroidism. J Nucl Med. 2022; 63:785–91.
58. Araz M, Soydal C, Ozkan E, Kir MK, Ibis E, Gullu S, et al. The efficacy of fluorine-18-choline PET/CT in comparison with 99mTc-MIBI SPECT/CT in the localization of a hyperfunctioning parathyroid gland in primary hyperparathyroidism. Nucl Med Commun. 2018; 39:989–94.


59. Ferrari C, Santo G, Mammucci P, Pisani AR, Sardaro A, Rubini G. Diagnostic value of choline PET in the preoperative localization of hyperfunctioning parathyroid gland(s): a comprehensive overview. Biomedicines. 2021; 9:231.


60. Broos WA, Wondergem M, Knol RJ, van der Zant FM. Parathyroid imaging with 18F-fluorocholine PET/CT as a first-line imaging modality in primary hyperparathyroidism: a retrospective cohort study. EJNMMI Res. 2019; 9:72.


61. Treglia G, Piccardo A, Imperiale A, Strobel K, Kaufmann PA, Prior JO, et al. Diagnostic performance of choline PET for detection of hyperfunctioning parathyroid glands in hyperparathyroidism: a systematic review and meta-analysis. Eur J Nucl Med Mol Imaging. 2019; 46:751–65.


62. Rep S, Hocevar M, Vaupotic J, Zdesar U, Zaletel K, Lezaic L. 18F-choline PET/CT for parathyroid scintigraphy: significantly lower radiation exposure of patients in comparison to conventional nuclear medicine imaging approaches. J Radiol Prot. 2018; 38:343–56.
63. Kawada K, Iwamoto M, Sakai Y. Mechanisms underlying 18F-fluorodeoxyglucose accumulation in colorectal cancer. World J Radiol. 2016; 8:880–6.


64. Evangelista L, Sorgato N, Torresan F, Boschin IM, Pennelli G, Saladini G, et al. FDG-PET/CT and parathyroid carcinoma: review of literature and illustrative case series. World J Clin Oncol. 2011; 2:348–54.


65. Neumann DR, Esselstyn CB, Kim EY. Recurrent postoperative parathyroid carcinoma: FDG-PET and sestamibi-SPECT findings. J Nucl Med. 1996; 37:2000–1.
66. Neumann DR, Esselstyn CB, Maclntyre WJ, Go RT, Obuchowski NA, Chen EQ, et al. Comparison of FDG-PET and sestamibi-SPECT in primary hyperparathyroidism. J Nucl Med. 1996; 37:1809–15.
67. Neumann DR, Esselstyn CB Jr, MacIntyre WJ, Chen EQ, Go RT, Kohse LM, et al. Primary hyperparathyroidism: preoperative parathyroid imaging with regional body FDG PET. Radiology. 1994; 192:509–12.


68. Melon P, Luxen A, Hamoir E, Meurisse M. Fluorine-18-fluorodeoxyglucose positron emission tomography for preoperative parathyroid imaging in primary hyperparathyroidism. Eur J Nucl Med. 1995; 22:556–8.


69. Strauss LG. Positron emission tomography: current role for diagnosis and therapy monitoring in oncology. Oncologist. 1997; 2:381–8.


70. Strauss LG. Sensitivity and specificity of positron emission tomography (PET) for the diagnosis of lymph node metastases. Recent Results Cancer Res. 2000; 157:12–9.


71. Morgat C, Velayoudom-Cephise FL, Schwartz P, Guyot M, Gaye D, Vimont D, et al. Evaluation of (68)Ga-DOTA-TOC PET/CT for the detection of duodenopancreatic neuroendocrine tumors in patients with MEN1. Eur J Nucl Med Mol Imaging. 2016; 43:1258–66.


72. Buchmann I, Henze M, Engelbrecht S, Eisenhut M, Runz A, Schafer M, et al. Comparison of 68Ga-DOTATOC PET and 111In-DTPAOC (Octreoscan) SPECT in patients with neuroendocrine tumours. Eur J Nucl Med Mol Imaging. 2007; 34:1617–26.


73. Gabriel M, Decristoforo C, Kendler D, Dobrozemsky G, Heute D, Uprimny C, et al. 68Ga-DOTA-Tyr3-octreotide PET in neuroendocrine tumors: comparison with somatostatin receptor scintigraphy and CT. J Nucl Med. 2007; 48:508–18.


74. Schreiter NF, Bartels AM, Froeling V, Steffen I, Pape UF, Beck A, et al. Searching for primaries in patients with neuroendocrine tumors (NET) of unknown primary and clinically suspected NET: evaluation of Ga-68 DOTATOC PET/CT and In-111 DTPA octreotide SPECT/CT. Radiol Oncol. 2014; 48:339–47.


75. Sharma P, Mukherjee A, Karunanithi S, Naswa N, Kumar R, Ammini AC, et al. Accuracy of 68Ga DOTANOC PET/CT imaging in patients with multiple endocrine neoplasia syndromes. Clin Nucl Med. 2015; 40:e351–6.


76. Froeling V, Elgeti F, Maurer MH, Scheurig-Muenkler C, Beck A, Kroencke TJ, et al. Impact of Ga-68 DOTATOC PET/CT on the diagnosis and treatment of patients with multiple endocrine neoplasia. Ann Nucl Med. 2012; 26:738–43.


77. Rodgers SE, Hunter GJ, Hamberg LM, Schellingerhout D, Doherty DB, Ayers GD, et al. Improved preoperative planning for directed parathyroidectomy with 4-dimensional computed tomography. Surgery. 2006; 140:932–41.


78. Starker LF, Mahajan A, Bjorklund P, Sze G, Udelsman R, Carling T. 4D parathyroid CT as the initial localization study for patients with de novo primary hyperparathyroidism. Ann Surg Oncol. 2011; 18:1723–8.


79. Mortenson MM, Evans DB, Lee JE, Hunter GJ, Shellingerhout D, Vu T, et al. Parathyroid exploration in the reoperative neck: improved preoperative localization with 4D-computed tomography. J Am Coll Surg. 2008; 206:888–96.


80. Lubitz CC, Hunter GJ, Hamberg LM, Parangi S, Ruan D, Gawande A, et al. Accuracy of 4-dimensional computed to mography in poorly localized patients with primary hyperparathyroidism. Surgery. 2010; 148:1129–38.


81. Eichhorn-Wharry LI, Carlin AM, Talpos GB. Mild hypercalcemia: an indication to select 4-dimensional computed tomography scan for preoperative localization of parathyroid adenomas. Am J Surg. 2011; 201:334–8.


82. Welling RD, Olson JA Jr, Kranz PG, Eastwood JD, Hoang JK. Bilateral retropharyngeal parathyroid hyperplasia detected with 4D multidetector row CT. AJNR Am J Neuroradiol. 2011; 32:E80–2.


83. Russell MT, Fink JR, Rebeles F, Kanal K, Ramos M, Anzai Y. Balancing radiation dose and image quality: clinical applications of neck volume CT. AJNR Am J Neuroradiol. 2008; 29:727–31.


84. Ibraheem K, Toraih EA, Haddad AB, Farag M, Randolph GW, Kandil E. Selective parathyroid venous sampling in primary hyperparathyroidism: a systematic review and meta-analysis. Laryngoscope. 2018; 128:2662–7.


85. Taslakian B, Trerotola SO, Sacks B, Oklu R, Deipolyi A. The essentials of parathyroid hormone venous sampling. Cardiovasc Intervent Radiol. 2017; 40:9–21.


86. Ogilvie CM, Brown PL, Matson M, Dacie J, Reznek RH, Britton K, et al. Selective parathyroid venous sampling in patients with complicated hyperparathyroidism. Eur J Endocrinol. 2006; 155:813–21.


87. Lee J, Hong N, Kim BM, Kim DJ, Yun M, Jeong JJ, et al. Evaluation of an optimal cutoff of parathyroid venous sampling gradient for localizing primary hyperparathyroidism. J Bone Miner Metab. 2020; 38:570–80.


88. Seehofer D, Steinmuller T, Rayes N, Podrabsky P, Riethmuller J, Klupp J, et al. Parathyroid hormone venous sampling before reoperative surgery in renal hyperparathyroidism: comparison with noninvasive localization procedures and review of the literature. Arch Surg. 2004; 139:1331–8.


89. Jones JJ, Brunaud L, Dowd CF, Duh QY, Morita E, Clark OH. Accuracy of selective venous sampling for intact parathyroid hormone in difficult patients with recurrent or persistent hyperparathyroidism. Surgery. 2002; 132:944–51.


90. Jaskowiak N, Norton JA, Alexander HR, Doppman JL, Shawker T, Skarulis M, et al. A prospective trial evaluating a standard approach to reoperation for missed parathyroid adenoma. Ann Surg. 1996; 224:308–21.


91. Witteveen JE, Kievit J, van Erkel AR, Morreau H, Romijn JA, Hamdy NA. The role of selective venous sampling in the management of persistent hyperparathyroidism revisited. Eur J Endocrinol. 2010; 163:945–52.


92. Ho J, Kim D, Lee JE, Hong N, Kim BM, Kim DJ, et al. Parathyroid venous sampling for the preoperative localisation of parathyroid adenoma in patients with primary hyperparathyroidism. Sci Rep. 2022; 12:7058.


93. Mahajan A, Starker LF, Ghita M, Udelsman R, Brink JA, Carling T. Parathyroid four-dimensional computed tomography: evaluation of radiation dose exposure during preoperative localization of parathyroid tumors in primary hyperparathyroidism. World J Surg. 2012; 36:1335–9.


94. Mettler FA Jr, Huda W, Yoshizumi TT, Mahesh M. Effective doses in radiology and diagnostic nuclear medicine: a catalog. Radiology. 2008; 248:254–63.


95. Akerstrom G, Malmaeus J, Bergstrom R. Surgical anatomy of human parathyroid glands. Surgery. 1984; 95:14–21.
96. Bilezikian JP, Silverberg SJ, Bandeira F, Cetani F, Chandran M, Cusano NE, et al. Task Force #8: management of primary hyperparathyroidism. J Bone Miner Res. 2022; Aug. 28. [Epub]. https://doi.org/10.1002/jbmr.4682.


97. Solorzano CC, Thomas G, Baregamian N, Mahadevan-Jansen A. Detecting the near infrared autofluorescence of the human parathyroid: hype or opportunity? Ann Surg. 2020; 272:973–85.
98. Alazraki N, Glass EC, Castronovo F, Olmos RA, Podoloff D; Society of Nuclear Medicine. Procedure guideline for lymphoscintigraphy and the use of intraoperative gamma probe for sentinel lymph node localization in melanoma of intermediate thickness 1.0. J Nucl Med. 2002; 43:1414–8.
99. Buicko JL, Kichler KM, Amundson JR, Scurci S, Kozol RA. The sestamibi paradox: improving intraoperative localization of parathyroid adenomas. Am Surg. 2017; 83:832–5.


100. Chen H, Sippel RS, Schaefer S. The effectiveness of radioguided parathyroidectomy in patients with negative technetium tc 99m-sestamibi scans. Arch Surg. 2009; 144:643–8.


101. Daliakopoulos SI, Chatzoulis G, Lampridis S, Pantelidou V, Zografos O, Ioannidis K, et al. Gamma probe-assisted excision of an ectopic parathyroid adenoma located within the thymus: case report and review of the literature. J Cardiothorac Surg. 2014; 9:62.


102. Jaskowiak NT, Sugg SL, Helke J, Koka MR, Kaplan EL. Pitfalls of intraoperative quick parathyroid hormone monitoring and gamma probe localization in surgery for primary hyperparathyroidism. Arch Surg. 2002; 137:659–69.


103. Tobin K, Ayers RR, Rajaei M, Sippel RS, Balentine CJ, Elfenbein D, et al. Use of the gamma probe to identify multigland disease in primary hyperparathyroidism. Int J Endocr Oncol. 2016; 3:13–9.


104. Ladurner R, Sommerey S, Arabi NA, Hallfeldt KK, Stepp H, Gallwas JK. Intraoperative near-infrared autofluorescence imaging of parathyroid glands. Surg Endosc. 2017; 31:3140–5.


105. Han N, Bumpous JM, Goldstein RE, Fleming MM, Flynn MB. Intra-operative parathyroid identification using methylene blue in parathyroid surgery. Am Surg. 2007; 73:820–3.


106. Suzuki T, Numata T, Shibuya M. Intraoperative photodynamic detection of normal parathyroid glands using 5-aminolevulinic acid. Laryngoscope. 2011; 121:1462–6.


107. Kose E, Kahramangil B, Aydin H, Donmez M, Berber E. Heterogeneous and low-intensity parathyroid autofluorescence: patterns suggesting hyperfunction at parathyroid exploration. Surgery. 2019; 165:431–7.


108. Falco J, Dip F, Quadri P, de la Fuente M, Prunello M, Rosenthal RJ. Increased identification of parathyroid glands using near infrared light during thyroid and parathyroid surgery. Surg Endosc. 2017; 31:3737–42.


109. Ladurner R, Al Arabi N, Guendogar U, Hallfeldt K, Stepp H, Gallwas J. Near-infrared autofluorescence imaging to detect parathyroid glands in thyroid surgery. Ann R Coll Surg Engl. 2018; 100:33–6.


110. Squires MH, Jarvis R, Shirley LA, Phay JE. Intraoperative parathyroid autofluorescence detection in patients with primary hyperparathyroidism. Ann Surg Oncol. 2019; 26:1142–8.


111. Merrill AL, Sims SS, Dedhia PH, Rossfeld K, Lott Limbach A, Duh QY, et al. Near-infrared autofluorescence features of parathyroid carcinoma. J Endocr Soc. 2022; 6:bvac090.


112. Morris MA, Saboury B, Ahlman M, Malayeri AA, Jones EC, Chen CC, et al. Parathyroid imaging: past, present, and future. Front Endocrinol (Lausanne). 2022; 12:760419.


113. Katz SC, Wang GJ, Kramer EL, Roses DF. Limitations of technetium 99m sestamibi scintigraphic localization for primary hyperparathyroidism associated with multiglandular disease. Am Surg. 2003; 69:170–5.
114. Parikh AM, Suliburk JW, Moron FE. Imaging localization and surgical approach in the management of ectopic parathyroid adenomas. Endocr Pract. 2018; 24:589–98.


115. Udelsman R. Approach to the patient with persistent or recurrent primary hyperparathyroidism. J Clin Endocrinol Metab. 2011; 96:2950–8.


116. Bioletto F, Barale M, Parasiliti-Caprino M, Prencipe N, Berton AM, Procopio M, et al. Comparison of the diagnostic accuracy of 18F-fluorocholine PET and 11C-methionine PET for parathyroid localization in primary hyperparathyroidism: a systematic review and meta-analysis. Eur J Endocrinol. 2021; 185:109–20.


Fig. 1.
Possible preoperative localization process for primary hyperparathyroidism. Dashed lines (---): Choline-positron emission tomography (PET) could be preferred to 11C-methionine (MET)-PET. Dot-dashed lines (─·─·): This process could be chosen with an experienced parathyroid surgeon. PHPT, primary hyperparathyroidism; SPECT/CT, single photon emission computed tomography/computed tomography; PVS, parathyroid venous sampling; 4D-CT, four-dimensional computed tomography; PET/CT, positron emission tomography/computed tomography. aWhen a patient is referred to a high-volume center, this process might be followed.
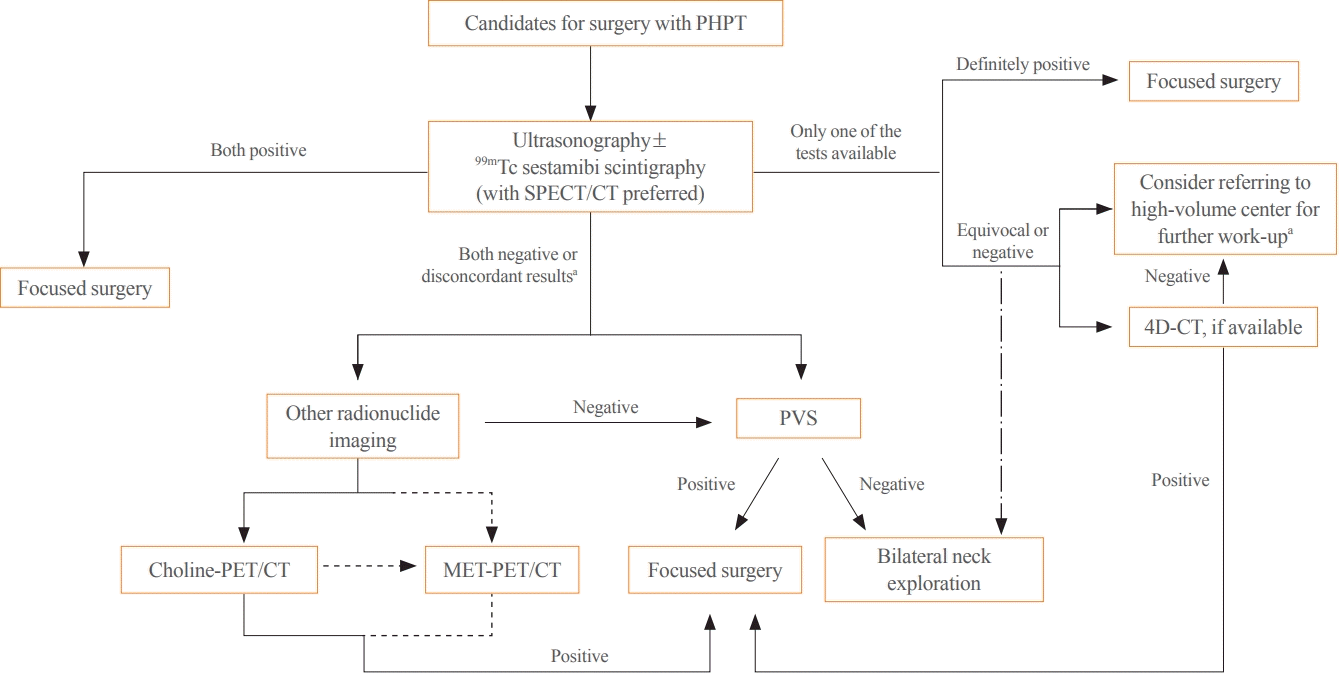
Table 1.
Advantages and Disadvantages of Localization Modalities