Abstract
Background
Copeptin is the carboxyl-terminal part of the vasopressin precursor protein, and its concentration is an independent predictor of the onset of chronic kidney disease and a rapid decline in the glomerular filtration rate. The glomerular filtration rate is regarded as the best indicator of kidney transplant function and is a predictor of graft and patient survival. We investigated the clinical significance of copeptin as an early predictor of renal graft dysfunction in renal transplant recipients.
Methods
We measured serum creatinine, cystatin C, and copeptin concentrations in renal transplant recipients on the day of their operation, as well as on postoperative days 3, 7, 30, and 365. Acute rejection was defined as a sudden decrease in renal function accompanied by histological changes.
Results
Eight renal transplant recipients were enrolled in the study from July 2018 to December 2019. Four patients experienced histologically confirmed transplant rejection. All four cases involved acute T-cell rejection. No significant correlation was found between the copeptin level and the presence or absence of rejection at any time point. In subgroup analyses, changes in creatinine, the estimated glomerular filtration rate, cystatin, and copeptin did not show statistical significance.
Renal transplant patients can experience graft dysfunction and rejection. Predicting which patients are at higher risk and why for this outcome may enable early and effective interventions. Copeptin, the C-terminal fragment of pro-arginine vasopressin precursor, was first discovered by Holwerda and his colleagues in 1972 as a Leucine-rich glycopeptide of relatively low molecular mass (about 3,248 Da) in the posterior pituitary of pigs, and its 39 amino acids sequence was determined in 1981 [1,2]. In a study using rats, continuous infusion of desmopressin, a vasopressin V2 receptor agonist, was associated with proteinuria and decreased renal function [3,4]. It has also been confirmed that renal function improves when water absorption is increased or when vasopressin levels are lowered using V1a/V2-receptor antagonists [5,6]. This is because it suppresses tubuloglomerular feedback by enhancing urea recycling and sodium chloride reabsorption. Vasopressin might be a suitable tool for predicting renal graft dysfunction and rejection. However, the study using desmopressin in kidney transplantation was mainly conducted on donors before transplantation. To the best of our knowledge, no studies have been reported on the association between desmopressin or copeptin and acute kidney transplant rejection in post-transplant recipients. Because vasopressin is difficult to measure due to technical complications, copeptin might be a better candidate. Copeptin is the carboxyl-terminal portion of the vasopressin precursor protein and it has been correlated with plasma vasopressin concentration [7]. Furthermore, copeptin independently predicts the onset of chronic kidney disease and a rapid decline in estimated glomerular filtration rate (eGFR) [8]. eGFR is generally regarded as the best indicator of renal graft function and is a predictor of both transplant and patient survival. Based on the results of previous studies, we theorize copeptin may be useful for predicting which patients are at higher risk of renal graft dysfunction and rejection.
This study assessed the correlation between changes in copeptin level with the function and prognosis of renal transplants by serially measuring the concentration of copeptin after transplantation.
Ethical statements: Our research protocol received the Institutional Review Board of Inje University Haeundae Paik Hospital's approval and was compliant with the Helsinki Declaration (IRB number: 2019-08-026-004). All study participants provided informed consent.
This study was conducted on patients undergoing renal transplantation at the Haeundae Paik Hospital from July 2018 to December 2019. Surgery time, amount of bleeding during surgery, cold ischemic time, warm ischemic time, human leukocyte antigen matching results, panel-reactive antibody screening, and immunosuppressants administered after surgery were all confirmed. In cases of renal function change in which clinically acute rejection was suspected during a 1-year follow-up period, confirmatory examination was performed via biopsy of the transplanted kidney.
Acute rejection was defined as a case diagnosed pathologically through renal biopsy. Warm ischemic time was the time from clamping of the aorta to cold perfusion. Cold ischemic time was the time from cold perfusion of the kidney to the venous anastomosis.
Examinations were performed the day before renal transplantation as well as on postoperative days 3, 7, 30, and 365. Examinations included serum copeptin, creatinine, sodium, and cystatin C. Serum osmolality was added in a calculated way. eGFR was calculated using the Chronic Kidney Disease Epidemiology (CKD-EPI) equation.
Copeptin testing was performed with a commercial enzyme-linked immunosorbent assay kit (CSB-E12130h; Cusabio Biotech Co., Ltd., Houston, TX, USA) using a serum sample stored at −20°C. The lower limit of detection was 19.53 pg/mL; the detection range was 78–5,000 pg/mL. Creatinine and cystatin C were measured on a Cobas c702 (Roche Diagnostics, Basel, Switzerland) using the following Roche reagents: CREJ2 (Creatinine Jaffe Gen.2) and CYSC2 (Tina-quant Cystatin C Gen.2).
The serum osmolality excluding ethanol was calculated by using the following formula for calculated osmolality excluding ethanol: 2 Na (mEq/L)+(urea [mg/dL])/2.8+(glucose [mg/dL])/18.
The study data were presented as frequencies with percentages for categorical variables and means±standard deviations for continuous variables. Mann-Whitney U test was performed to verify whether there was a significant difference in baseline, postoperative days 3, 7, 30, and 365. copeptin according to the presence or absence of kidney rejection. The correlation between changes in eGFR or cystatin C and copeptin was analyzed by Spearman correlation test. Spearman correlation analysis was performed using the median value of each test result from baseline to postoperative days 365. All statistical analyses were carried out using SPSS 25.0 version software (IBM Corp., Armonk, NY, USA); p-values less than 0.05 were considered significant.
Patient characteristics are presented in Table 1. Eight patients who underwent living or deceased donor kidney transplantation were enrolled in the study. Two of them were lost during follow-up. One patient stopped collecting samples after the baseline examination, and the other failed to perform additional examinations other than the baseline examination due to a thrombus and re-anastomosis that occurred after transplantation. Seven patients received a deceased donor kidney transplant and one had a living donor kidney transplant. There were five male and three female patients with an average age of 50.6 years. The average operation time was 239.4 minutes and the average amount of bleeding during surgery was 525.0 mL. All eight patients received tacrolimus, mycophenolate mofetil, prednisolone, and basiliximab as immunosuppressants after surgery. Cold ischemic time was 264.8 minutes, and warm ischemic time was 48.8 minutes.
Four patients underwent histological examination and transplantation rejection was confirmed. All were acute T-cell rejection. Of the six patients who had been followed for 1 year, three experienced renal rejection. The timing of occurrence of acute rejection in three patients is as follows; one patient 10 months after surgery, the other 8 months after surgery, and the other 2 months after surgery. Additional results were summarized for these six patients. Median copeptin concentration for the whole group at baseline was 122.25 pg/mL. Median copeptin concentration was 102.2 pg/mL for men and 138.35 pg/mL for women. The distribution of copeptin values ranged from 85.3 to 322.8 pg/mL. The median copeptin concentration in patients with rejection was 191.4 pg/mL at baseline and the median copeptin concentration in patients without rejection was 102.2 pg/mL at baseline. In both groups, copeptin level increased during the first 30 days, and decreased after 1 year of follow-up (Fig. 1). The baseline copeptin did not show any significant difference according to the presence or absence of rejection (p=0.686). There was no significant correlation in copeptin level for each period according to the presence or absence of rejection (day 3 copeptin, p=1.000, day 7 copeptin, p=0.800, day 30 copeptin, p=0.667, and day 365 copeptin, p=0.200).
Fig. 2 shows the results of clinical examinations during 1 year of follow-up. Patients 1 to 3 were patients with rejection and 4 to 6 were patients with no rejection. Fig. 2A shows the change in eGFR over time for each patient, Fig. 2B shows the change in copeptin, Fig. 2C shows the change in creatinine, and Fig. 2D shows the change in cystatin C. Most patients showed improvement of renal function compared to baseline, and renal function was maintained during 1-year follow-up. After transplantation, all six patients remained without dialysis.
As the baseline creatinine level increased, baseline copeptin concentration tended to increase, but there was no statistically significant correlation (rho=0.405, p=0.320). As the baseline eGFR level increases, the baseline copeptin concentration tends to increase, but there was no statistical significance (rho=0.405, p=0.320). The correlation between eGFR change and copeptin was calculated to be 0.300, but it did not show statistical significance (p=0.624). The correlation between cystatin C and copeptin was calculated as a correlation coefficient of –0.700 and did not show statistical significance (p=0.188). Subgroup analysis was performed as a group with rejection. The correlation coefficient between eGFR change and copeptin was calculated to be –0.200, but it did not show statistical significance (p=0.747). The correlation between cystatin C and copeptin was calculated as a correlation coefficient of –0.400 and did not show statistical significance (p=0.600).
Our study investigated whether changes in the concentration of copeptin after transplantation could predict transplant function decline. Other well-known tests for predicting renal function include creatinine and cystatin C. However, due to many factors such as chronic illness, malnutrition, and muscle wasting, serum creatinine is not reliable as a marker of renal function in transplant patients. There is also a method for measuring renal function using inulin, 99mTc-DTPA, or iohexol, but these processes are complicated and expensive to use in routine clinical practice. Previous studies of renal function measurement that have been useful in kidney transplant patients have been conducted. According to White et al. [9], the cystatin C-based equation was found to be more accurate in predicting eGFR in kidney transplant recipients than the existing creatinine-based equation. Meanwhile, according to a study by Luis-Lima et al. [10], both creatinine-based formulas and cystatin C-based formulas show low precision and accuracy to reflect actual renal function in kidney transplant recipients. As such, the test results for estimating renal function in transplant patients are considered to be different compared to the general population, and these results are related to events such as medications taken by patients, physiologic changes, infection, or rejection of the transplant. We believe this study is meaningful because accurate renal function tracking in kidney transplant patients is very important in predicting which patients are at higher risk of renal graft dysfunction and rejection.
Many risk factors for rejection are known, such as age, human leukocyte antigen mismatching, and the number of transplants. According to a study by Han et al. [11], hyponatremia after kidney transplantation was associated with graft failure and mortality. After confirming that copeptin is related to osmoregulation, the study was expected to predict graft failure after kidney transplantation. However, in our study, copeptin level was not significantly different depending on the presence or absence of rejection. It should be considered that previous studies have shown hyponatremia is associated with graft failure, but the mechanism and direct causality have not been verified. If hyponatremia was a companion event to the outcome of the transplant, there was no significant difference in our study considering that all patients were in a dialysis-free state.
According to a study by Mazloum et al. [12], osmoregulation affects transplant results. Chronically elevated vasopressin is associated with worsening renal function. Copeptin, a vasopressin precursor, has been identified in previous cohort studies as an independent predictor of the development of new chronic kidney disease and rapid decrease in eGFR [8]. eGFR is generally regarded as the best indicator of graft function and a predictor of patient survival. Meanwhile, according to a study by Meijer et al. [13], since baseline copeptin level correlated with changes in the eGFR during the follow-up of 3.2 years, vasopressin may play a role in renal function changes in kidney transplant patients. However, this study confirmed the relationship with subsequent changes in renal function by measuring baseline copeptin. Therefore, considering that copeptin is associated with kidney and urinary tract diseases, infections of the upper respiratory tract, and some physiological states such as fasting or thirsting, it is difficult to control all other patient conditions at the time of copeptin measurement, which limits the study [7,14,15]. In addition, since copeptin levels are also associated with diabetic macrovascular and microvascular complications, it should be considered that renal function deteriorated due to complications from diabetes, which may affect the outcome of transplanted patients [16-19]. With these limitations in mind, we tried to confirm the association between serial changes in copeptin and renal function, but our study did not provide meaningful results. In addition, although there was improvement in eGFR and all patients were stable without dialysis, the copeptin concentration did not show a statistically significant relationship with changes in eGFR, creatinine, or cystatin C. The reason for not finding statistically meaningful results is considered to be the limitation of our research. No statistical significance was found, but similar trends in copeptin concentration and renal function were confirmed. It is expected that statistical significance can be secured by collecting a large number of patients and clinical results over a longer period. This is a study conducted by a single institution and the relatively small sample size is a limitation. Since they were all T-cell rejection, it may have been more difficult to reveal the correlation, so it would be a limitation. It is also expected that a longer follow-up period will be required. Previous studies have shown that copeptin in the heart but not the kidney transplant population was independently associated with kidney and heart function. It may also predict the outcome of orthotopic heart transplants. It may be worthwhile to establish copeptin ranges in relation to kidney function among heart and kidney allograft recipients [20]. In our study, the left ventricular ejection fraction was presented as data reflecting the patient's heart function. We did not find an association between copeptin and renal function changes. Therefore, follow-up studies including additional data such as New York Heart Association Class or ProBNP or Soluble ST2 are expected. Copeptin levels can be affected by a variety of different physiologic and pathologic conditions, and additional corrections may be needed [7,14,15]. It is unlikely that the copeptin level will provide more information to predict renal function changes compared with creatinine and cystatin C, which are already in use. However, if we consider the relationship between copeptin and vasopressin, we should consider the possibility of benefiting from treatment with V2-receptor antagonists in patients with high copeptin levels or those at high risk of decreased renal function. To study these new treatments, it will be necessary to confirm the correlation between changes in copeptin levels and changes in renal function in certain patient groups, such as patients with renal transplantation.
Copeptin level was expected to identify individuals with a high risk of declining graft function or with a high likelihood of rejection; however, we found no statistically significant association. Further research is needed to overcome the limitations of our study, and it is expected that this will ensure a longer graft survival and increase the patient's survival.
Notes
Author contributions
Conceptualization: YJL, YWK. Data curation: SP, IHK. Formal analysis: SP, IHK. Investigation: JHP, JK. Methodology: JHP, JK. Project administration: BSP. Resources: SP, IHK. Software: SP, IHK. Supervision: YWK. Validation: BSP. Visualization: BSP. Writing - original draft: YJL, CMH. Writing - review & editing: YJL, CMH. Approval of final manuscript: all authors.
References
1. Holwerda DA. A glycopeptide from the posterior lobe of pig pituitaries. I. Isolation and characterization. Eur J Biochem. 1972; 28:334–9.
2. Seidah NG, Benjannet S, Chretien M. The complete sequence of a novel human pituitary glycopeptide homologous to pig posterior pituitary glycopeptide. Biochem Biophys Res Commun. 1981; 100:901–7.
3. Bouby N, Hassler C, Bankir L. Contribution of vasopressin to progression of chronic renal failure: study in Brattleboro rats. Life Sci. 1999; 65:991–1004.
4. Bardoux P, Bichet DG, Martin H, Gallois Y, Marre M, Arthus MF, et al. Vasopressin increases urinary albumin excretion in rats and humans: involvement of V2 receptors and the renin-angiotensin system. Nephrol Dial Transplant. 2003; 18:497–506.
5. Bouby N, Bachmann S, Bichet D, Bankir L. Effect of water intake on the progression of chronic renal failure in the 5/6 nephrectomized rat. Am J Physiol. 1990; 258(4 Pt 2):F973–9.
6. Perico N, Zoja C, Corna D, Rottoli D, Gaspari F, Haskell L, et al. V1/V2 Vasopressin receptor antagonism potentiates the renoprotection of renin-angiotensin system inhibition in rats with renal mass reduction. Kidney Int. 2009; 76:960–7.
7. Morgenthaler NG, Struck J, Alonso C, Bergmann A. Assay for the measurement of copeptin, a stable peptide derived from the precursor of vasopressin. Clin Chem. 2006; 52:112–9.
8. Tasevska I, Enhorning S, Christensson A, Persson M, Nilsson PM, Melander O. Increased levels of copeptin, a surrogate marker of arginine vasopressin, are associated with an increased risk of chronic kidney disease in a general population. Am J Nephrol. 2016; 44:22–8.
9. White C, Akbari A, Hussain N, Dinh L, Filler G, Lepage N, et al. Estimating glomerular filtration rate in kidney transplantation: a comparison between serum creatinine and cystatin C-based methods. J Am Soc Nephrol. 2005; 16:3763–70.
10. Luis-Lima S, Marrero-Miranda D, Gonzalez-Rinne A, Torres A, Gonzalez-Posada JM, Rodriguez A, et al. Estimated glomerular filtration rate in renal transplantation: the nephrologist in the mist. Transplantation. 2015; 99:2625–33.
11. Han SS, Han M, Park JY, An JN, Park S, Park SK, et al. Posttransplant hyponatremia predicts graft failure and mortality in kidney transplantation recipients: a multicenter cohort study in Korea. PLoS One. 2016; 11:e0156050.
12. Mazloum M, Jouffroy J, Brazier F, Legendre C, Neuraz A, Garcelon N, et al. Osmoregulation performance and kidney transplant outcome. J Am Soc Nephrol. 2019; 30:1282–93.
13. Meijer E, Bakker SJ, de Jong PE, Homan van der Heide JJ, van Son WJ, Struck J, et al. Copeptin, a surrogate marker of vasopressin, is associated with accelerated renal function decline in renal transplant recipients. Transplantation. 2009; 88:561–7.
14. Morgenthaler NG, Struck J, Jochberger S, Dunser MW. Copeptin: clinical use of a new biomarker. Trends Endocrinol Metab. 2008; 19:43–9.
15. Mockel M, Searle J, Hamm C, Slagman A, Blankenberg S, Huber K, et al. Early discharge using single cardiac troponin and copeptin testing in patients with suspected acute coronary syndrome (ACS): a randomized, controlled clinical process study. Eur Heart J. 2015; 36:369–76.
16. Potier L, Roussel R, Marre M, Bjornstad P, Cherney DZ, El Boustany R, et al. Plasma copeptin and risk of lower-extremity amputation in type 1 and type 2 diabetes. Diabetes Care. 2019; 42:2290–7.
17. Villela-Torres ML, Higareda-Mendoza AE, Gomez-Garcia A, Alvarez-Paredes AR, Garcia-Lopez E, Stenvikel P, et al. Copeptin plasma levels are associated with decline of renal function in patients with type 2 diabetes mellitus. Arch Med Res. 2018; 49:36–43.
18. Zhu FX, Wu HL, Tu KS, Chen JX, Zhang M, Shi C. Serum levels of copeptin are associated with type 2 diabetes and diabetic complications in Chinese population. J Diabetes Complications. 2016; 30:1566–70.
19. Enhorning S, Hedblad B, Nilsson PM, Engstrom G, Melander O. Copeptin is an independent predictor of diabetic heart disease and death. Am Heart J. 2015; 169:549–56.
20. Malyszko J, Przybylowski P, Koc-Zorawska E, Iaina-Levin N, Sadowski J, Mysliwiec M, et al. Copeptin in relation to New York Heart Association class in heart transplant recipients and kidney transplant recipients. Transplant Proc. 2010; 42:4259–62.
Fig. 2.
Changes in parameters over time for each patient. Changes in (A) the estimated glomerular filtration rate, (B) copeptin concentrations, (C) creatinine levels, and (D) cystatin C concentrations.
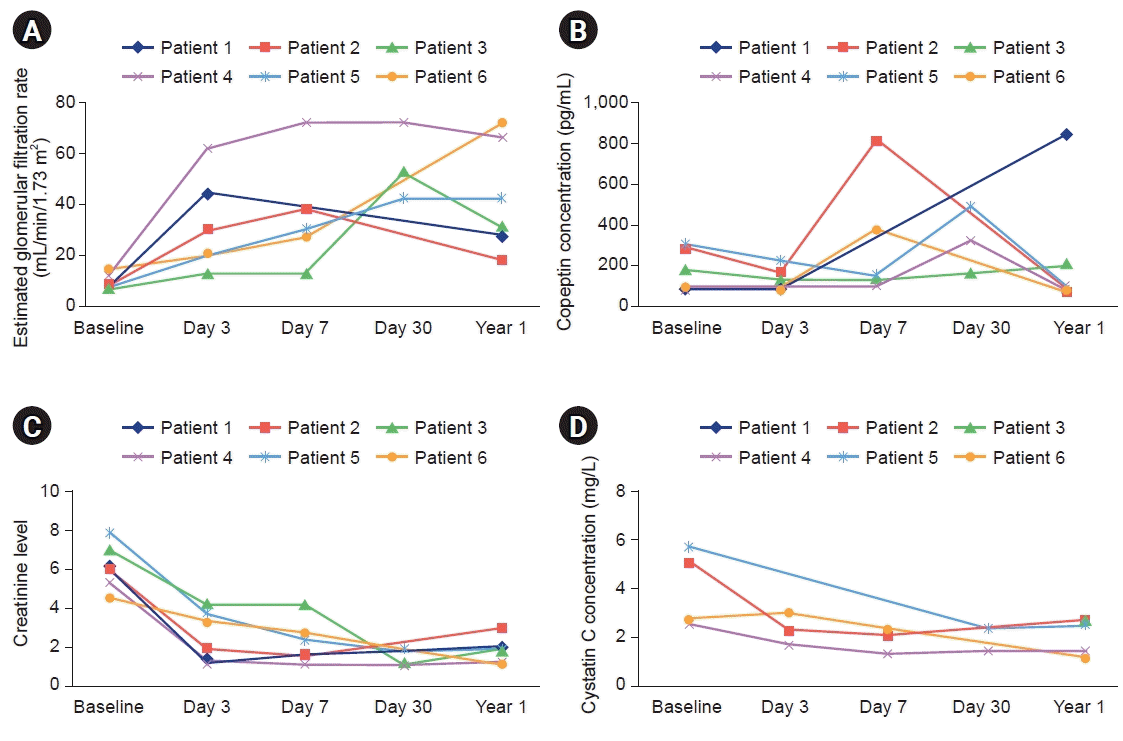
Table 1.
Characteristics of the study population