Abstract
Increased inflammation and insulin resistance are commonly observed in obesity and diabetes. Inflammatory mediators secreted by the adipose tissue contribute to the pathogenesis of diabetes and cardiovascular diseases. Free fatty acids and pro-inflammatory cytokines from adipose tissue inhibit the intracellular insulin signaling pathway, further contributing to the progression of diabetes. Meta-analysis studies show that high sensitivity C-reactive protein can be used as a predictor of future all-cause mortality, including cardiovascular and cancer mortality. In addition to the discovery of novel therapeutic methods targeting inflammatory mediators, basic lifestyle interventions, such as regular exercise, healthy eating, and proper weight control, are absolutely crucial for reducing inflammation and preventing mortality.
Free fatty acids and pro-inflammatory cytokines from the adipose tissue have a negative effect on the intracellular insulin signaling pathway. Free fatty acids activate atypical protein kinase C (PKC), IκB kinase (IKK-β), c-jun N-terminal kinase, leading to the serine/threonine phosphorylation of insulin receptor substrate (IRS) 1 and the inhibition of its signaling.1) As a result, free fatty acids contribute to the insulin resistance in target tissues. Free fatty acids can also promote the secretion of pro-inflammatory cytokines by binding to Toll-like receptors.
The very first experimental evidence connecting inflammation to insulin resistance was the report showing that the overexpression of tumor necrosis factor alpha (TNFα) induced insulin resistance.2),3) Since that original report, numerous proteins secreted by adipose tissues were found to be associated with insulin resistance, including monocyte chemoattractant protein-1 (MCP-1), plasminogen activator inhibitor-1, interleukin (IL)-6, resistin, leptin, visfatin, angiotensinogen, retinol binding protein 4 (RBP4), serum amyloid A, and many others. TNFα secreted by the adipose tissue promotes the lipolysis of adipose tissue and inhibits insulin signaling. Furthermore, the neutralization of TNFα reverses insulin resistance in mouse models; however, this phenomenon has not been observed in humans. IL-6 increases the expression of suppressor of cytokine signaling 3 (SOCS-3) and inhibits IRS signaling through the induction of SOCS-3.4)
TNFα receptors, IL-1 receptors, Toll-like receptors, and advanced glycation end-product receptors mediate the intracellular inflammatory process by stimulating IKK-β/nuclear factor kappa-light-chain-enhancer of activated B cells (NF-κB) and JNK signaling. Furthermore, reactive oxygen species, endoplasmic reticulum (ER) stress, ceramide, and PKC isoforms have been implicated in the regulation of intracellular inflammation.1)
In addition to insulin target tissues, pancreatic islet inflammation could be detrimental to the pancreatic function, resulting in diabetes progression and insulin secretory dysfunction. In the pancreatic islets of type 2 diabetes patients, inflammatory cell infiltration, amyloid deposition, and the expression of high cytokine levels can be detected, eventually leading to the development of fibrosis. This type of pancreatic inflammation is commonly observed as a result of obesity, with cytokines, hyperglycemia, and dyslipidemia further contributing to this process. Moreover, high expression levels of IL-1β promote insulin secretory dysfunction and beta-cell apoptosis; however, in obese type 2 diabetes patients, IL-1β antagonists have been shown to decrease islet inflammation, as well as blood glucose levels.5) This increased IL-1β expression has also been observed in a gene array study of human islets from type 2 diabetes patients.6)
Recently, the prevalence of non-alcoholic fatty liver disease (NAFLD) has been increasing and it is commonly detected in obese patients. Inflammation also plays an important role in the progression of NAFLD; furthermore, the expression of intra-hepatic inflammatory markers increases proportionally relative to the degree of obesity. Since inflammation can be induced by increased lipid deposits in the adipose tissue, similar processes occur in the liver tissue. In obese individuals, free fatty acids and pro-inflammatory cytokines enter through the portal vein, promoting inflammation in the liver and inducing selective hepatic insulin resistance. In the liver, these cytokines are known to activate Kupffer cells, cells responsible for the induction of the inflammatory processes. In addition to Kupffer cells, other immune cells, such as natural killer cells, B cells, T cells, dendritic cells, and hepatic stellate cells, can also mediate inflammation, resulting in the progression of NAFLD to steatohepatitis and liver cirrhosis.
Several studies described the connection between inflammatory cytokines and the development of NAFLD. For example, adipocyte-specific fatty acid-binding protein (A-FABP) is expressed in adipocytes and is associated with insulin resistance, metabolic syndrome, and type 2 diabetes. It has been reported that the levels of A-FABP are positively associated with the NAFLD stage, the levels of TNFα, insulin resistance index, and metabolic syndrome.7) In another study, Park et al.8) investigated whether insulin resistance and systemic inflammatory markers could be used as independent predictors of NAFLD in non-obese men. In this cross-sectional study, multivariate analysis indicated that an increase in C-reactive protein (CRP) per 1 standard deviation (SD; 1.48 mg/L) and homeostatic model assessment-insulin resistance per 1 SD (0.63) were independent risk factors for NAFLD.8)
We investigated the connection between silent mating type information regulation 2 homolog (sirtuin, SIRT), NAFLD, and insulin resistance.9) The SIRT family consists of nicotinamide adenine dinucleotide-dependent enzymes that modify proteins via de-acetylation. For example, SIRT1 promotes fatty acid oxidation in the liver by activating adenosine monophosphateactivated protein kinase; however, the connection between exendin-4 and SIRT1 expression has not been previously reported. Therefore, we used high-fat diet-induced obesity mouse model (C57BL/6J), as well as cell culture models, to investigate whether the protective effects of exendin-4 on fatty liver involved SIRT. We observed that exendin-4 increased SIRT1 expression in mice with high-fat diet-induced NAFLD by stimulating fatty acid oxidation.10) Subsequently, we also showed that exendin-4 treatment elevated SIRT1 expression, leading to the reduction of ER stress parameters in hepatocytes11)
The adipose tissue secrets numerous factors that are involved in metabolism, inflammation, and immunity. Inflammatory mediators produced by the adipose tissue are known to contribute to the pathogenesis of diabetes and cardiovascular diseases. For example, serum levels of inflammatory cytokines are different between healthy and obese subjects, suggesting the indirect role of these mediators. Blood levels of pro-inflammatory cytokines are also associated with strokes and cardiovascular mortality.12) For example, MCP-1 is highly expressed in the adipose tissues of obese subjects; however, the blood levels of MCP-1, IL-6, RBP4, and visfatin are decreased if these obese subjects were to lose their body weight. When morbidly obese subjects lose weight, the reduction of both adipose tissues and adipose tissue macrophages is observed.13) Therefore, the expansion of adipose tissues can induce inflammatory processes in the body and contribute to the progression of metabolic and cardiovascular diseases.
Clinical studies clearly demonstrate the connection between subclinical inflammation and the development of diabetes and cardiovascular diseases. For example, in the crosssectional and longitudinal studies, the blood levels of high sensitivity CRP (hsCRP), an inflammatory marker, were closely correlated with the metabolic syndrome components.14),15) It was reported that there was significant correlation between hsCRP and the blood glucose levels even in the euglycemic state, with the glucose levels and hsCRP positively correlated in 10,059 healthy Korean individuals.16) In Rancho-Bernardo study, the baseline levels of IL-6 and hsCRP were significantly correlated with the severity of carotid intima-media thickness,17) while baseline blood TNFα levels were associated with the later progression of glycemic status and NAFLD.18),19) Furthermore, weight gain for several years was correlated with increased inflammation, and the elevated levels of inflammatory markers, such as haptoglobin, ceruloplasmin, alpha1-antitrypsin, orosomucoid, and fibrinogen, at baseline could be used as the predictors of future weight gain.20)
We also investigated the impact of inflammation on the correlation between insulin resistance and mortality in 165,849 apparently healthy Korean adults in a longitudinal study. During the follow-up period of 1,417,325.6 person-years, there was a correlation between the levels of hsCRP and all-cause and cancer-related mortality risks, irrespective of insulin resistance status. This correlation was more evident in the case of cancer-related mortality.21) The Da Qing Diabetes Study showed that there was a significant association between the baseline hsCRP levels and 10-year all-cause mortality.22) Furthermore, the meta-analysis demonstrated that elevated hsCRP levels could predict the risk of all-cause and cardiovascular mortality in the general population.23)
Thiazolidinediones, anti-diabetic drugs, has anti-inflammatory effects in addition to its anti-hyperglycemic properties. It can inhibit NF-κB activity and reduce inflammatory signaling. Furthermore, peroxisome proliferator-activated receptor alpha (PPARα), PPARδ, liver X receptor are nuclear receptors that also have anti-inflammatory effects. We previously reported that pioglitazone, a PPARγ agonist, significantly reduced inflammation and ER stress in a mouse insulinoma cell line (Figure 1).24) The anti-inflammatory effect of pioglitazone was also confirmed in vivo using pancreatic islets of high-fat-fed KK-Ay mice. In these experiments, pioglitazone prevented β-cell apoptosis by reducing ER stress and inflammation.24) Statins also inhibit the activation of NF-κB, activator protein-1, hypoxiainducible factor 1-alpha, and reduce the expression of cytokines and CRP. In 1876, Ebstein25) reported that high dose salicylate had glucose-lowering effects; these glucose-reducing effects of salicylate were due to the inhibition of NF-κB signaling. High doses of aspirin (up to 7 g/day) have been shown to lower the blood levels of free fatty acids, triglycerides, glucose, and induce numerous metabolic effects, such as the inhibition of hepatic glucose production and the stimulation of insulin-dependent glucose transport. However, high doses of aspirin also increase bleeding, limiting its use. In randomized controlled trials, salsalate, a non-acetylated salicylate, was reported to have glucose-lowering effect, although renal safety issues may limit its clinical application.26) Additional evidence is needed to further assess the effect of salsalate on metabolic diseases.
Lifestyle intervention plays a very important role in the reduction of low-grade inflammation. The Action for Health in Diabetes trial, which examined the effect of intensive lifestyle intervention (ILI) on cardiovascular mortality in overweight/obese type 2 diabetic subjects, found that CRP was significantly reduced in the ILI group.27)
Inflammation and insulin resistance observed in obesity lead to metabolic diseases. Current research is focused on targeting inflammation, since the reduction of inflammation would improve metabolic diseases. Basic lifestyle interventions, such as regular exercise and healthy eating, are very important even in the absence of weight reduction and should be regarded as essential treatment methods.
REFERENCES
2. Hotamisligil GS, Shargill NS, Spiegelman BM. Adipose expression of tumor necrosis factor-α: direct role in obesity-linked insulin resistance. Science. 1993; 259:87–91.


3. Feinstein R, Kanety H, Papa MZ, Lunenfeld B, Karasik A. Tumor necrosis factor-α suppresses insulininduced tyrosine phosphorylation of insulin receptor and its substrates. J Biol Chem. 1993; 268:26055–8.
4. Senn JJ, Klover PJ, Nowak IA, Zimmers TA, Koniaris LG, Furlanetto RW, Mooney RA. Suppressor of cytokine signaling-3 (SOCS-3), a potential mediator of interleukin-6-dependent insulin resistance in hepatocytes. J Biol Chem. 2003; 278:13740–6.


5. Larsen CM, Faulenbach M, Vaag A, Vølund A, Ehses JA, Seifert B, Mandrup-Poulsen T, Donath MY. Interleukin-1-receptor antagonist in type 2 diabetes mellitus. N Engl J Med. 2007; 356:1517–26.
6. Böni-Schnetzler M, Thorne J, Parnaud G, Marselli L, Ehses JA, Kerr-Conte J, Pattou F, Halban PA, Weir GC, Donath MY. Increased interleukin (IL)-1beta messenger ribonucleic acid expression in beta -cells of individuals with type 2 diabetes and regulation of IL-1beta in human islets by glucose and autostimulation. J Clin Endocrinol Metab. 2008; 93:4065–74.
7. Kim YC, Cho YK, Lee WY, Kim HJ, Park JH, Park DI, Sohn CI, Jeon WK, Kim BI, Park SE, Rhee EJ, Park CY, Oh KW, Park SW, Kim SW, Ryu SH. Serum adipocyte-specific fatty acid-binding protein is associated with nonalcoholic fatty liver disease in apparently healthy subjects. J Nutr Biochem. 2011; 22:289–92.


8. Park SH, Kim BI, Yun JW, Kim JW, Park DI, Cho YK, Sung IK, Park CY, Sohn CI, Jeon WK, Kim H, Rhee EJ, Lee WY, Kim SW. Insulin resistance and C-reactive protein as independent risk factors for non-alcoholic fatty liver disease in non-obese Asian men. J Gastroenterol Hepatol. 2004; 19:694–8.


9. Purushotham A, Schug TT, Xu Q, Surapureddi S, Guo X, Li X. Hepatocyte-specific deletion of SIRT1 alters fatty acid metabolism and results in hepatic steatosis and inflammation. Cell Metab. 2009; 9:327–38.


10. Lee J, Hong SW, Chae SW, Kim DH, Choi JH, Bae JC, Park SE, Rhee EJ, Park CY, Oh KW, Park SW, Kim SW, Lee WY. Exendin-4 improves steatohepatitis by increasing Sirt1 expression in high-fat diet-induced obese C57BL/6J mice. PLoS One. 2012; 7:e31394.


11. Lee J, Hong SW, Park SE, Rhee EJ, Park CY, Oh KW, Park SW, Lee WY. Exendin-4 attenuates endoplasmic reticulum stress through a SIRT1-dependent mechanism. Cell Stress Chaperones. 2014; 19:649–56.


12. Rosenson RS, Koenig W. Utility of inflammatory markers in the management of coronary artery disease. Am J Cardiol. 2003; 92:10i–8i.


13. Cancello R, Henegar C, Viguerie N, Taleb S, Poitou C, Rouault C, Coupaye M, Pelloux V, Hugol D, Bouillot JL, Bouloumié A, Barbatelli G, Cinti S, Svensson PA, Barsh GS, Zucker JD, Basdevant A, Langin D, Clément K. Reduction of macrophage infiltration and chemoattractant gene expression changes in white adipose tissue of morbidly obese subjects after surgery-induced weight loss. Diabetes. 2005; 54:2277–86.


14. Lee WY, Park JS, Noh SY, Rhee EJ, Sung KC, Kim BS, Kang JH, Kim SW, Lee MH, Park JR. C-reactive protein concentrations are related to insulin resistance and metabolic syndrome as defined by the ATP III report. Int J Cardiol. 2004; 97:101–6.


15. Jung CH, Lee WY, Kim SY, Shin HS, Kim HD, Rhee EJ, Park CY, Oh KW, Park SW, Kim SW. The risk of metabolic syndrome according to the high-sensitivity C-reactive protein in apparently healthy Koreans. Int J Cardiol. 2008; 129:266–71.


16. Rhee EJ, Kim YC, Lee WY, Jung CH, Sung KC, Ryu SH, Oh KW, Kim SW. Comparison of insulin resistance and serum high-sensitivity C-reactive protein levels according to the fasting blood glucose subgroups divided by the newly recommended criteria for fasting hyperglycemia in 10059 healthy Koreans. Metabolism. 2006; 55:183–7.


17. Lee WY, Allison MA, Kim DJ, Song CH, Barrett-Connor E. Association of interleukin-6 and C-reactive protein with subclinical carotid atherosclerosis (the Rancho Bernardo Study). Am J Cardiol. 2007; 99:99–102.


18. Rhee EJ, Seo MH, Jeon WS, Won HK, Choi JH, Park SE, Park CY, Kwag HJ, Oh KW, Park SW, Kim SW, Lee WY. The association of baseline adipocytokine levels with glycemic progression in nondiabetic Korean adults in 4 years of follow-up. Diabetes Res Clin Pract. 2012; 98:501–7.


19. Seo YY, Cho YK, Bae JC, Seo MH, Park SE, Rhee EJ, Park CY, Oh KW, Park SW, Lee WY. Tumor necrosis factor-α as a predictor for the development of nonalcoholic fatty liver disease: a 4-year follow-up study. Endocrinol Metab (Seoul). 2013; 28:41–5.


20. Engström G, Hedblad B, Stavenow L, Lind P, Janzon L, Lindgärde F. Inflammation-sensitive plasma proteins are associated with future weight gain. Diabetes. 2003; 52:2097–101.


21. Lee DY, Rhee EJ, Chang Y, Sohn CI, Shin HC, Ryu S, Lee WY. Impact of systemic inflammation on the relationship between insulin resistance and all-cause and cancer-related mortality. Metabolism. 2018; 81:52–62.


22. Qian X, He S, Wang J, Gong Q, An Y, Li H, Chen Y, Li G. Prediction of 10-year mortality using hs-CRP in Chinese people with hyperglycemia: findings from the Da Qing diabetes prevention outcomes study. Diabetes Res Clin Pract. 2021; 173:108668.


23. Li Y, Zhong X, Cheng G, Zhao C, Zhang L, Hong Y, Wan Q, He R, Wang Z. Hs-CRP and all-cause, cardiovascular, and cancer mortality risk: a meta-analysis. Atherosclerosis. 2017; 259:75–82.


24. Hong SW, Lee J, Cho JH, Kwon H, Park SE, Rhee EJ, Park CY, Oh KW, Park SW, Lee WY. Pioglitazone attenuates palmitate-induced inflammation and endoplasmic reticulum stress in pancreatic β-cells. Endocrinol Metab (Seoul). 2018; 33:105–13.


25. Ebstein W. Zur therapie des diabetes mellitus, insbesondere über die anwendung des salicylsauren natron bei demselben. Berl Klin Wochenschr. 1876; 13:337–40.
26. Salastekar N, Desai T, Hauser T, Schaefer EJ, Fowler K, Joseph S, Shoelson SE; Goldfine ABTINSAL-CVD study team. Salsalate improves glycaemia in overweight persons with diabetes risk factors of stable statintreated cardiovascular disease: a 30-month randomized placebo-controlled trial. Diabetes Obes Metab. 2017; 19:1458–62.


27. Belalcazar LM, Haffner SM, Lang W, Hoogeveen RC, Rushing J, Schwenke DC, Tracy RP, Pi-Sunyer FX, Kriska AM; Ballantyne CMLook AHEAD (Action for Health in Diabetes) Research Group. Lifestyle intervention and/or statins for the reduction of C-reactive protein in type 2 diabetes: from the look AHEAD study. Obesity (Silver Spring). 2013; 21:944–50.


Figure 1.
Pio improves PA-induced β-cell impairment by suppressing the inflammatory response and ER stress. (A) MIN6 cells were incubated with 0.5 mM PA in the presence or absence of 10 µM Pio for 24 hours, and the glucose-stimulated (1 or 4.5 g/L) insulin secretion of MIN6 cells was evaluated. Secreted insulin was measured using a mouse insulin enzyme-linked immunosorbent assay kit. The values are representative of 6 independent experiments. (B) The expression of c-casp3, an apoptotic protein, was measured by Western blot analysis. (C) PARP activity is presented as the percentage of absorbance relative to the veh group. (D) Expression of TNFα, IL-6, and IL-1β was measured by real time reverse-transcription polymerase chain reaction, and normalized to ACTB. (E) ER stress proteins, including p-eIF2α, GRP78, c-ATF6, and CHOP, were measured by Western blot analysis. (F) Quantification of p-eIF2α, GRP78, and CHOP protein levels; the results were normalized to β-actin. Each value represents the mean of 3 experiments.
ACTB = β-actin; c-ATF6 = cleaved-activating transcription factor 6; c-casp3 = cleaved caspase-3; CHOP = C/EBP homologous protein; ER = endoplasmic reticulum; GRP78 = glucose-regulated protein 78; IL = interleukin; MIN6 = mouse insulinoma 6; mRNA = messenger RNA; PA = palmitate; PARP = poly (adenosine diphosphate [ADP]-ribose) polymerase; p-eIF2α = phospho-eukaryotic translation initiation factor 2α; Pio = pioglitazone; t-casp3 = total caspase-3; TNFα = tumor necrosis factor alpha; Veh = vehicle.
*p<0.01, †p<0.001 compared to the vehicle group; ‡p<0.05, §p<0.001 compared to the PA group (adapted from Hong SW, et al.24)).
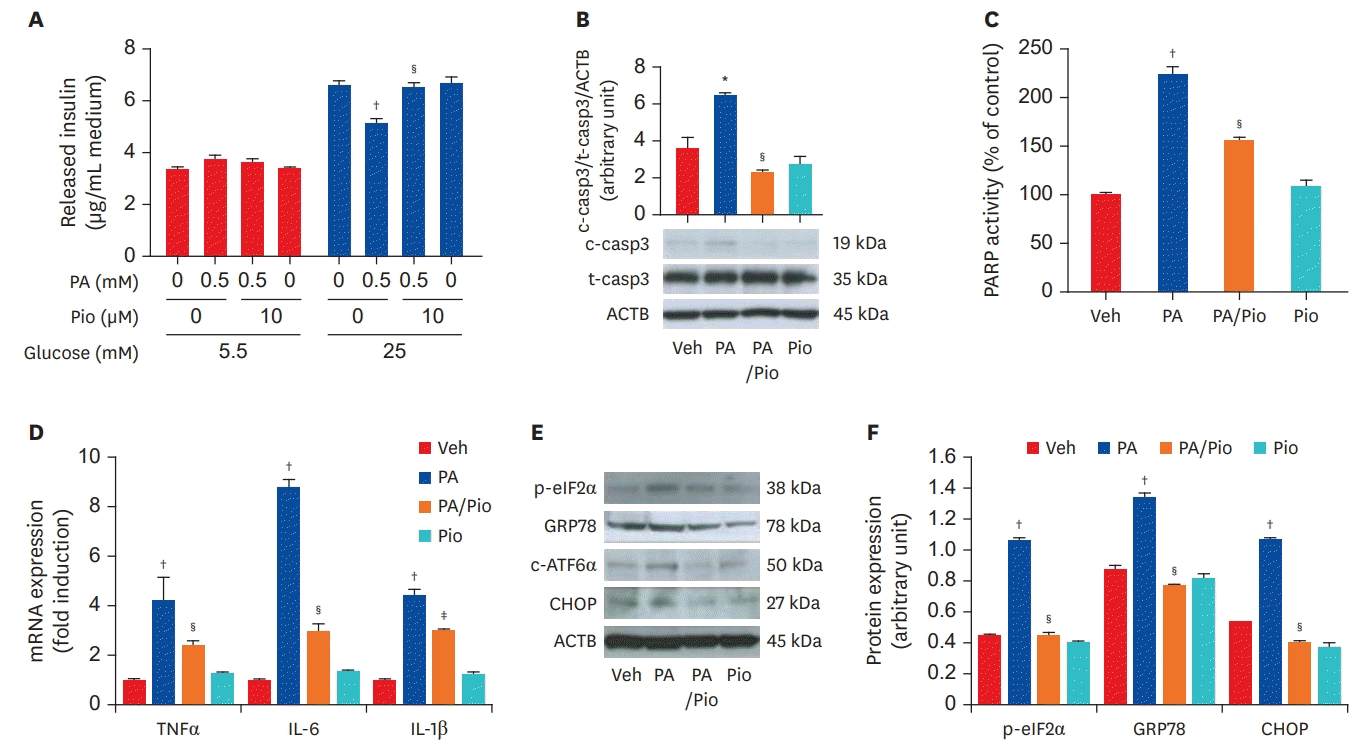