Abstract
Background
The clinical outcomes of delayed radioiodine remnant ablation (RRA) therapy in patients with low-risk papillary thyroid carcinoma (PTC) are unclear. We aimed to evaluate the clinical impact of the interval between total thyroidectomy (TT) and RRA therapy in patients with low-risk PTC.
Methods
We included 526 patients who underwent TT and RRA for low-risk PTC with a primary tumor size of >1 cm between 2000 and 2012. Patients were divided into the early (<90 days) and the delayed (≥90 days) RRA groups based on the interval between TT and RRA. The results of diagnostic whole-body scan (DxWBS), ongoing risk stratification (ORS; response to therapy), and disease-free survival (DFS) were evaluated before and after propensity score matching (PSM).
Results
Among the 526 patients, 75 (14.3%) patients underwent delayed RRA; they had more cervical lymph node metastasis and received a higher RRA dose than those who underwent early RRA. The median follow-up period was 9.1 years after initial therapy, and the structural recurrence rate was 1.9%. In DxWBS, 60 patients had focal iodine uptake limited in operative bed, with no significant difference between groups. According to ORS, 78%, 20%, 1%, and 1% patients were classified into excellent, indeterminate, biochemical incomplete, and structural incomplete response groups, respectively. There was no significant difference in ORS or DFS between groups before and after PSM.
Radioiodine remnant ablation (RRA) is a traditional therapeutic option for patients with differentiated thyroid carcinoma (DTC). The goal of RRA is to ablate residual thyroid and microscopic cancer tissues including distant metastasis for improving disease-free survival (DFS) and reducing disease specific mortality [1–3]. Previous studies have reported on the efficacy of RRA for improving DFS and overall survival in patients with intermediate- or high-risk DTC [4–6]. However, the efficacy of RRA in patients with low-risk DTC remains unclear [6–10]. In general, therapeutic doses of RRA are determined by clinicopathological prognostic factors or initial risk stratification; however, the doses vary among different countries and hospitals [1].
The optimal timing of RRA and the risk of delayed RRA have not been substantiated [2,11–13]. The interval between total thyroidectomy (TT) and initial RRA varied by country and is affected by facility availability, socioeconomic status of the patients, and disease-related factors [11,12]. A previous study has suggested that a delay in initial RRA of >180 days is associated with poor survival outcome after TT in patients with advanced DTC [13]. However, the clinical outcomes after delayed RRA remain controversial, and it is unclear whether immediate RRA therapy offers any clinical benefits compared to delayed RRA therapy [14].
The recent increase in the incidence of small papillary thyroid carcinoma (PTC) and better clinical outcomes in patients with low-risk PTC have prompted a discussion regarding the necessity of RRA for low-risk PTC patients [6,7,10,15,16]. Although the role of RRA is controversial, the usefulness of RRA cannot be completely denied since patients with low-risk PTC also have some clinicopathological factors such as high postoperative thyroglobulin (Tg) level, large tumor size, and cervical lymph node (LN) metastasis [1,9]. Therefore, it is important to understand whether RRA therapy can be delayed during the follow-up of patients with low-risk PTC.
To the best of our knowledge, no clinical study has evaluated the optimal timing of RRA or the effect of delayed RRA in patients with low-risk PTC according to the American Thyroid Association (ATA) risk stratification. Therefore, we compared the clinical outcomes in patients with low-risk PTC treated with early and delayed RRA before and after propensity score matching (PSM).
Patients with PTC who underwent TT followed by RRA between 2000 and 2012 were eligible for enrollment in the current study. The definition of low-risk PTC was determined according to the ATA guideline as follows: without local or distant metastasis; no extrathyroidal extension; negative residual margin; no radioactive iodine (RAI) avid metastatic foci outside the thyroid bed on the first post-surgical RAI scan; no lymphovascular invasion; and clinical N0 or <5 pathologic N1 micrometastases (<0.2 cm in the largest dimension) [1]. Of the 1,446 patients with low-risk PTC, those with papillary microcarcinoma measuring <1 cm were excluded. Finally, 526 patients were enrolled in this study. The patients were divided into the early RRA group (<90 days; n=451) and the delayed RRA group (≥90 days; n=75) based on the interval between TT and the first RRA [2]. Written informed consent by the patients was waived due to a retrospective nature of our study and the study protocol was approved by the Institutional Review Board of Asan Medical Center (study number: 2019-1562), Seoul, South Korea.
All patients underwent TT with or without central neck dissection, followed by RRA. The RRA therapy and I-131 therapeutic doses were decided according to the protocol of Asan Medical Center [2]. All patients underwent the thyroid hormone withdrawal method for RRA. The I-131 doses were determined based on clinicopathological parameters. In brief, PTCs with a primary tumor size of <4 cm that were pathologically defined as N0 were treated with 30 mCi of I-131. PTCs with a primary tumor size of <4 cm that were pathologically defined as N1a were treated with 80 mCi of I-131. All PTCs with a primary tumor size of >4 cm with or without cervical LN metastasis were treated with 150 mCi of I-131.
After RRA, all patients were administered levothyroxine for suppression of thyroid-stimulating hormones. Patients were followed up regularly (every 6 to 24 months) with physical examination, neck ultrasonography (US), and laboratory tests including thyroid function, serum Tg, and anti-thyroglobulin antibody (TgAb) tests. During the first 12 to 24 months of initial therapy, neck US and/or diagnostic whole-body scan (DxWBS) were performed with measurement of stimulated Tg (sTg) and TgAb, and the response to the initial therapy was assessed [17–20]. If suspected signs for recurrence were present in routine follow-up, further evaluation, such as additional imaging or biopsy, was performed. Additional diagnostic imaging studies, such as computed tomography (CT) or whole-body fluorodeoxyglucose positron emission tomography/CT, were performed in some patients, as required.
The study outcomes were ongoing risk stratification (ORS; response to therapy) and DFS between the two groups based on the interval of RRA. ORS was categorized into excellent, indeterminate, biochemical incomplete, structural incomplete response based on the response to the initial therapy within the first 2 years of follow-up [1,2,21,22]. DFS was calculated from the date of the initial operation to the date of confirmation of structural recurrence by cytopathology. Disease-specific survival was not included in the study outcome because the mortality rate was very low in the low-risk PTC cases.
Rstudio version 1.1.463 (RStudio: Integrated Development for R, RStudio Inc., Boston, MA, USA; www.rstudio.com) was used to perform statistical analyses. Continuous variables are presented as median with interquartile range (IQR), and categorical variables are presented as frequencies with percentages. PSM was used to adjust baseline characteristics related to disease recurrence. The propensity score was defined as the probability of being assigned to the early and delayed RRA group based on age, sex, tumor size, T stage, LN metastasis, initial ablation dose, and RAI avidity. After PSM, a standardized mean difference was used to measure balancing covariates between the groups; if a variable was balanced, then the standardized mean difference was <0.1. The chi-square test was used to determine associations between categorical variables. DFS curves were plotted using the Kaplan-Meier method, and the log-rank test was used to determine their significance. The Cox proportional hazards model was used to evaluate the risk factors for recurrent disease and was presented as hazard ratio and 95% confidence interval. P values of <0.05 were considered statistically significant.
The baseline clinical and pathological characteristics of the 526 patients with low-risk PTC is shown in Table 1 (left panel). The median interval between RRA and the initial surgery was 45 days (IQR, 40 to 56) and 127 days (IQR, 110 to 137.5) in the early RRA and delayed RRA groups, respectively (P<0.001). The median age of the patients was 47.2 years, and of the 526 patients, 93 (17.7%) were males. The median primary tumor size was 1.4 cm, and approximately 85% patients had T1 staging according to the 8th edition of the American Joint Committee on Cancer (AJCC) tumor node metastasis (TNM) staging system. Details of AJCC TNM staging system in each group are shown in Supplemental Table S1. There was no significant difference in age, sex, primary tumor size, and T staging between the early and delayed RRA groups. Cervical LN metastasis was confirmed in 80 patients (12%), and patients in the delayed RRA group had significantly more metastasis than those in the early RRA group (64% vs. 7%). In 80 patients with LN metastasis, the number of metastatic LN was as below; 1 (n=46, 57.5%), 2 (n=24, 30.0%), 3 (n=5, 6.2%), and 4 (n=5, 6.2%). The median size of metastatic LN was 0.1 cm (minimum to maximum range, 0.01 to 0.15). Further, patients in the delayed RRA group received a significantly higher RRA dose than those in the early RRA group (P<0.001). Therefore, we evaluated these two groups after PSM, and 57 patients were included in each group. There was no significant difference in LN metastasis and RRA dose between the two groups after PSM (Table 1, right panel).
In the first 12 to 24 months after initial therapy, DxWBS was performed in 431 (95.6%) and 72 (96%) patients in the early RRA and delayed RRA groups, respectively. There was no abnormal iodine uptake outside the operative bed and no evidence of cervical LN metastasis or distant metastasis. In total, 56 (13%) and four patients (5.6%) had focal iodine uptake limited in the operative bed in the early and delayed RRA groups, respectively. There was no significant difference in the proportion of patients with iodine uptake between the early and delayed RRA groups before and after PSM.
We evaluated the initial treatment response by comparing ORS between the early and delayed RRA groups (Table 2). A majority of the patients (77.6%) showed an excellent response, and approximately 20% showed an indeterminate response. Approximately 1% patients showed a biochemical or structural incomplete response. There was no significant difference in the initial treatment response between the early and delayed RRA groups before and after PSM.
The median follow-up period was 9.1 years (IQR, 6.8 to 11.8) after initial therapy, and the structural recurrence rate was 1.9% (10/ 526). The recurrence rate was 1.6% (7/451) and 4% (3/75) in the early and delayed RRA groups, respectively, but there was no significant difference (P=0.242). There was no significant difference in DFS between the two groups before and after PSM (P=0.181 and P=0.094, respectively) (Fig. 1). All cases of recurrent disease were locoregional recurrence, and all patients with recurrent disease underwent additional surgery, such as lateral neck dissection or selective LN excision (Table 3). After additional surgical treatment, all patients were disease free during the follow-up period, and there were no cases of disease-specific death.
Table 4 shows the clinical and pathological parameters associated with recurrent disease in patients with low-risk PTC. In multivariate analyses using the Cox-proportional hazard model, male sex and primary tumor size (>2 cm) were independent risk factors of recurrent PTC. Delayed RRA was not a significant risk factor in the univariate and multivariate analyses. High RRA dose (5.55 GBq, 150 mCi) was a significant risk factor in the univariate analysis, but was not significant in the multivariate analysis.
In this study, we evaluated the clinical outcomes of patients with low-risk PTC who underwent early and delayed RRA therapy after TT. In this cohort, only 1.9% patients had recurrent disease during a median follow-up of 9.1 years after the initial treatment. We compared DFS and ORS categories based on the initial therapy response before and after PSM because there was a significant difference in cervical LN metastasis and I-131 doses for RRA. There was no significant difference in DFS and ORS categories between early and delay RRA groups. These findings suggest that the decision regarding adjuvant RRA therapy can be delayed in patients with low-risk PTC after TT.
Currently, there is no specific consensus regarding delayed RRA therapy in patients with DTC [1,23]. Previous studies have evaluated the clinical implications of the timing of RRA therapy owing to the concerns of limitation to number of special rooms for isolation needed to RRA [2,11–14]. Scheffel et al. [12] evaluated the clinical outcomes in 545 patients with DTC according to the timing of RRA (early RRA ≤6 months vs. late RRA >6 months) irrespective of the initial risk factors. There was no significant difference in recurrent DTC between the early and delayed RRA groups. However, their study included patients with low- (42%), intermediate- (45%), and high-risk DTC (13%) according to the ATA initial risk stratification, and there was a significant difference in the baseline characteristics, such as cervical LN metastasis and age, between the two groups. In a study by Li et al. [11], delayed RRA (≥3 months) was significantly associated with incomplete treatment response and reduced excellent response in 235 patients with low- and intermediate-risk DTC. However, the study did not evaluate recurrent disease and included both patients with low- and intermediate-risk DTC. Thus, the abovementioned studies could not provide evidence of clinical outcomes after delayed RRA in patients with low-risk DTC.
A study by Tsirona et al. [14] evaluated the clinical impact of delayed RRA in 107 patients with AJCC TNM stage I (T1N0M0 or T2N0M0) DTC. The study did not find any significant difference in the remission rate and recurrent disease between early (≤4.7 months) and delayed (>4.7 months) RRA groups during a median 7 years of follow-up; however, a majority of patients without cervical LN metastasis were not administered RRA because of a very-low risk of recurrence. In our study, we included 50% patients with cervical LN metastasis and approximately 20% patients with TNM stage II and III after PSM. Moreover, we excluded patients with microPTC to reduce the bias considering the very low risk of recurrence in patients with microPTC [24–26]. Nevertheless, the clinical outcomes of delayed RRA therapy were comparable with those of early RRA in patients with low-risk DTC in our study. These results suggest that RRA can be delayed, and a decision can be made after considering the results of postoperative Tg levels or DxWBS.
Owing to the nature of retrospective study, all patients in this study underwent RRA according to the previous consensus and guidelines [23,27,28]. Many clinical studies have evaluated the efficacy of RRA in patients with low-risk DTCs, and a majority of these studies have suggested that there is no significant benefit of RRA in terms of clinical outcomes in patients with low-risk DTCs [6,10,15]. These results have prompted a discussion regarding the necessity of RRA for the treatment of low-risk PTC. RRA is not routinely recommended, and the decision of RRA should be determined considering additional risk factors such as postoperative serum Tg levels and DxWBS results [1,9]. In our multivariate analysis, large tumor size (>2 cm) and male sex were significant risk factors for the disease recurrence in patients with low-risk PTC. Further studies should consider appropriate indications and doses of RRA, including these two risk factors, to provide firm evidence for the optimal timing of RRA.
There are several limitations in our study. First, the selection bias cannot be excluded in this retrospective single center study. Second, given the generally excellent clinical outcome of low-risk PTC, we could not to evaluate the disease-specific mortality. Third, there were more patients with cervical LN metastasis in the delayed RRA group than in the early RRA before PSM, which explains the higher I-131 doses for RRA in the delayed group. Because the high dose of RAI requires hospitalization for isolation, the delayed RRA was due to limitation of special rooms for isolation. After PSM, the number of patients in each group were relatively small, which could have affected the statistical power. However, to our knowledge, this study is the first to evaluate the clinical outcomes of delayed RRA in patients with low-risk PTC according to the ATA risk stratification. Further prospective studies are warranted to confirm the optimal timing of RRA.
In conclusion, there was no significant difference in clinical outcomes between early and delayed RRA in patients with low-risk PTC. Our findings suggest that the clinical decision for RRA therapy and the dose of RRA can be determined >3 months after thyroid surgery and considering postoperative changes in serum Tg levels or DxWBS results.
Supplementary Data
Supplemental Table S1
Tumor and Node Stage of the Patients with Low-Risk Differentiated Thyroid Carcinoma before and after Propensity Score Matching
ACKNOWLEDGMENTS
This study was supported by the National Research Foundation (NRF) of Korea Research Grant (NRF-2018R1D1A1A02085365).
REFERENCES
1. Haugen BR, Alexander EK, Bible KC, Doherty GM, Mandel SJ, Nikiforov YE, et al. 2015 American Thyroid Association management guidelines for adult patients with thyroid nodules and differentiated thyroid cancer: the American Thyroid Association guidelines task force on thyroid nodules and differentiated thyroid cancer. Thyroid. 2016; 26:1–133.


2. Kim M, Han M, Jeon MJ, Kim WG, Kim IJ, Ryu JS, et al. Impact of delayed radioiodine therapy in intermediate-/high-risk papillary thyroid carcinoma. Clin Endocrinol (Oxf). 2019; 91:449–55.


3. Taieb D, Sebag F, Cherenko M, Baumstarck-Barrau K, Fortanier C, Farman-Ara B, et al. Quality of life changes and clinical outcomes in thyroid cancer patients undergoing radioiodine remnant ablation (RRA) with recombinant human TSH (rhTSH): a randomized controlled study. Clin Endocrinol (Oxf). 2009; 71:115–23.


4. Ruel E, Thomas S, Dinan M, Perkins JM, Roman SA, Sosa JA. Adjuvant radioactive iodine therapy is associated with improved survival for patients with intermediate-risk papillary thyroid cancer. J Clin Endocrinol Metab. 2015; 100:1529–36.


5. Orosco RK, Hussain T, Noel JE, Chang DC, Dosiou C, Mittra E, et al. Radioactive iodine in differentiated thyroid cancer: a national database perspective. Endocr Relat Cancer. 2019; 26:795–802.


6. Sacks W, Fung CH, Chang JT, Waxman A, Braunstein GD. The effectiveness of radioactive iodine for treatment of low-risk thyroid cancer: a systematic analysis of the peer-reviewed literature from 1966 to April 2008. Thyroid. 2010; 20:1235–45.


7. Schvartz C, Bonnetain F, Dabakuyo S, Gauthier M, Cueff A, Fieffe S, et al. Impact on overall survival of radioactive iodine in low-risk differentiated thyroid cancer patients. J Clin Endocrinol Metab. 2012; 97:1526–35.


8. Ibrahimpasic T, Nixon IJ, Palmer FL, Whitcher MM, Tuttle RM, Shaha A, et al. Undetectable thyroglobulin after total thyroidectomy in patients with low- and intermediate-risk papillary thyroid cancer: is there a need for radioactive iodine therapy? Surgery. 2012; 152:1096–105.


9. Marti JL, Morris LG, Ho AS. Selective use of radioactive iodine (RAI) in thyroid cancer: no longer “one size fits all”. Eur J Surg Oncol. 2018; 44:348–56.


10. Schlumberger M, Catargi B, Borget I, Deandreis D, Zerdoud S, Bridji B, et al. Strategies of radioiodine ablation in patients with low-risk thyroid cancer. N Engl J Med. 2012; 366:1663–73.


11. Li H, Zhang YQ, Wang C, Zhang X, Li X, Lin YS. Delayed initial radioiodine therapy related to incomplete response in low- to intermediate-risk differentiated thyroid cancer. Clin Endocrinol (Oxf). 2018; 88:601–6.


12. Scheffel RS, Zanella AB, Dora JM, Maia AL. Timing of radioactive iodine administration does not influence outcomes in patients with differentiated thyroid carcinoma. Thyroid. 2016; 26:1623–9.


13. Higashi T, Nishii R, Yamada S, Nakamoto Y, Ishizu K, Kawase S, et al. Delayed initial radioactive iodine therapy resulted in poor survival in patients with metastatic differentiated thyroid carcinoma: a retrospective statistical analysis of 198 cases. J Nucl Med. 2011; 52:683–9.


14. Tsirona S, Vlassopoulou V, Tzanela M, Rondogianni P, Ioannidis G, Vassilopoulos C, et al. Impact of early vs late postoperative radioiodine remnant ablation on final outcome in patients with low-risk well-differentiated thyroid cancer. Clin Endocrinol (Oxf). 2014; 80:459–63.
15. Sawka AM, Thephamongkhol K, Brouwers M, Thabane L, Browman G, Gerstein HC. Clinical review 170: a systematic review and metaanalysis of the effectiveness of radioactive iodine remnant ablation for well-differentiated thyroid cancer. J Clin Endocrinol Metab. 2004; 89:3668–76.
16. Iniguez-Ariza NM, Brito JP. Management of low-risk papillary thyroid cancer. Endocrinol Metab (Seoul). 2018; 33:185–94.


17. Ahn J, Song E, Kim WG, Kim TY, Kim WB, Shong YK, et al. Long-term clinical outcomes of papillary thyroid carcinoma patients with biochemical incomplete response. Endocrine. 2020; 67:623–9.


18. Jeon MJ, Kim WG, Park WR, Han JM, Kim TY, Song DE, et al. Modified dynamic risk stratification for predicting recurrence using the response to initial therapy in patients with differentiated thyroid carcinoma. Eur J Endocrinol. 2013; 170:23–30.


19. Jeon MJ, Kim M, Park S, Oh HS, Kim TY, Kim WB, et al. A follow-up strategy for patients with an excellent response to initial therapy for differentiated thyroid carcinoma: less is better. Thyroid. 2018; 28:187–92.


20. Oh HS, Ahn JH, Song E, Han JM, Kim WG, Kim TY, et al. Individualized follow-up strategy for patients with an indeterminate response to initial therapy for papillary thyroid carcinoma. Thyroid. 2019; 29:209–15.


21. Park S, Kim WG, Song E, Oh HS, Kim M, Kwon H, et al. Dynamic risk stratification for predicting recurrence in patients with differentiated thyroid cancer treated without radioactive iodine remnant ablation therapy. Thyroid. 2017; 27:524–30.


22. Tuttle RM, Tala H, Shah J, Leboeuf R, Ghossein R, Gonen M, et al. Estimating risk of recurrence in differentiated thyroid cancer after total thyroidectomy and radioactive iodine remnant ablation: using response to therapy variables to modify the initial risk estimates predicted by the new American Thyroid Association staging system. Thyroid. 2010; 20:1341–9.


23. American Thyroid Association (ATA) Guidelines Taskforce on Thyroid Nodules and Differentiated Thyroid Cancer, Cooper DS, Doherty GM, Haugen BR, Kloos RT, Lee SL, et al. Revised American Thyroid Association management guidelines for patients with thyroid nodules and differentiated thyroid cancer. Thyroid. 2009; 19:1167–214.


24. Miyauchi A, Ito Y, Oda H. Insights into the management of papillary microcarcinoma of the thyroid. Thyroid. 2018; 28:23–31.


25. Leboulleux S, Tuttle RM, Pacini F, Schlumberger M. Papillary thyroid microcarcinoma: time to shift from surgery to active surveillance? Lancet Diabetes Endocrinol. 2016; 4:933–42.


26. Kim TY, Shong YK. Active surveillance of papillary thyroid microcarcinoma: a mini-review from Korea. Endocrinol Metab (Seoul). 2017; 32:399–406.


Fig. 1
The disease-free survival of patient with low-risk papillary thyroid carcinoma based on the interval between total thyroidectomy and radioiodine remnant ablation (RRA). (A) It represents total population and (B) represents patients after propensity score matching.
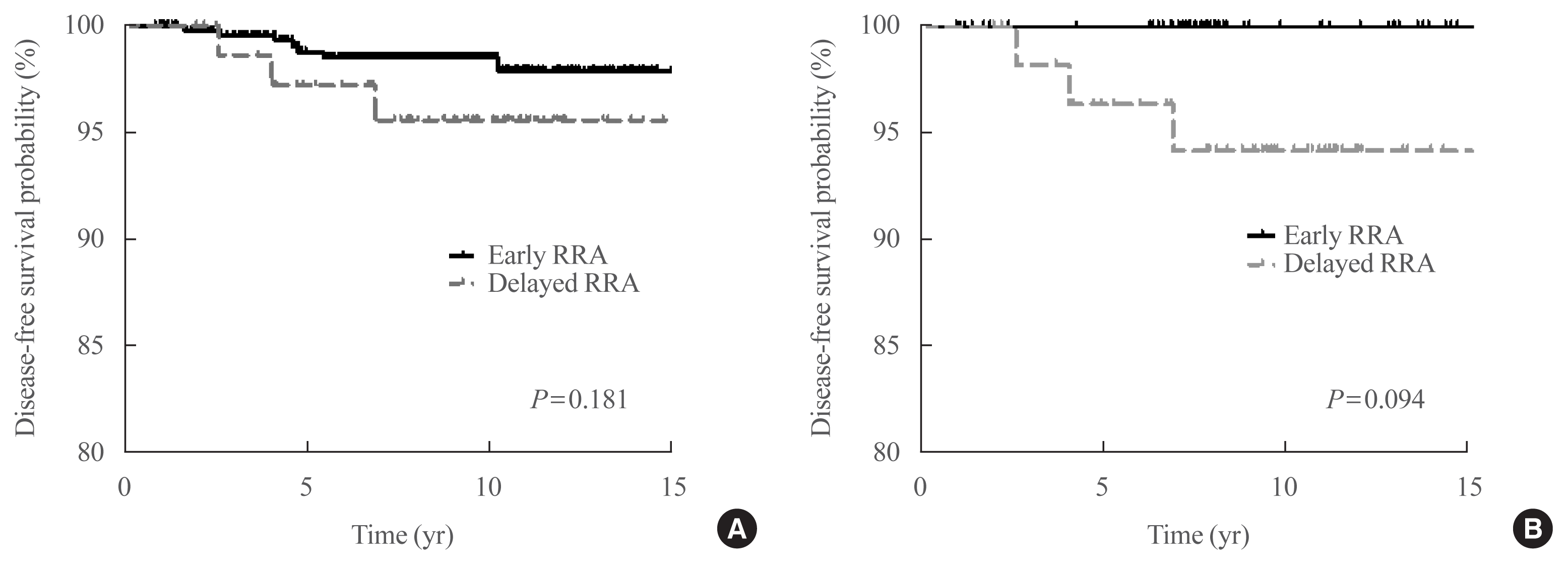
Table 1
Baseline Characteristics of Patients with Low-Risk Differentiated Thyroid Carcinoma before and after Propensity Score Matching
Characteristic | Total patients | Propensity score matching | ||||||
---|---|---|---|---|---|---|---|---|
|
|
|||||||
Total (n=526) | Early RRA (n=451) | Delayed RRA (n=75) | P value | Total (n=114) | Early RRA (n=57) | Delayed RRA (n=57) | P value | |
Age, yr | 47.2 (39.3–55.0) | 47.9 (39.9–55.0) | 45.0 (38.0–55.5) | 0.533 | 45.0 (37.9–57.0) | 45.9 (38.0–55.9) | 45.0 (37.9–57.0) | 0.777 |
|
||||||||
Male sex | 93 (17.7) | 79 (17.5) | 14 (18.7) | 21 (18.4) | 12 (21.1) | 9 (15.8) | 0.629 | |
|
||||||||
Tumor size, cm | 1.4 (1.1–1.8) | 1.4 (1.1–1.8) | 1.5 (1.1–2.0) | 0.163 | 1.5 (1.2–2.0) | 1.5 (1.2–2.0) | 1.5 (1.2–2.0) | 0.841 |
|
||||||||
T stagea | 0.645 | 0.761 | ||||||
T1 | 445 (84.6) | 383 (84.9) | 62 (82.7) | 93 (81.6) | 48 (84.2) | 45 (78.9) | ||
T2 | 68 (12.9) | 58 (12.9) | 10 (13.3) | 16 (14.0) | 7 (12.3) | 9 (15.8) | ||
T3a | 13 (2.5) | 10 (2.2) | 3 (4.0) | 5 (4.4) | 2 (3.5) | 3 (5.3) | ||
|
||||||||
LN metastasis | 80 (15.2) | 32 (7.1) | 48 (64.0) | <0.001 | 57 (50.0) | 28 (49.1) | 29 (50.9) | 0.999 |
|
||||||||
RRA dose, GBq | <0.001 | 0.999 | ||||||
1.11 | 380 (72.2) | 366 (81.2) | 14 (18.7) | 28 (24.6) | 14 (24.6) | 14 (24.6) | ||
2.96 | 119 (22.6) | 69 (15.3) | 50 (66.7) | 68 (59.6) | 34 (59.6) | 34 (59.6) | ||
5.55 | 27 (5.1) | 16 (3.5) | 11 (14.7) | 18 (15.8) | 9 (15.8) | 9 (15.8) |
Table 2
Ongoing Risk Stratification after Initial Treatment of Patients with Low-Risk Papillary Thyroid Carcinoma in the Early and Delayed RRA Groups
Table 3
Description of 10 Patients with Low-Risk Papillary Thyroid Carcinoma Who Had Local Recurrence during Follow-up
Table 4
Clinicopathological Factors Associated with Recurrent Disease in Patients with Low-Risk Papillary Thyroid Carcinoma