Abstract
Background
The optimal dose of radioactive iodine (RAI) therapy for N1b papillary thyroid carcinoma (PTC) is controversial. We evaluated the clinical outcome of N1b PTC patients treated with either 100 or 150 mCi of RAI.
Methods
We retrospectively analyzed N1b PTC patients who underwent total thyroidectomy and postoperative RAI therapy at a tertiary referral center between 2012 and 2017. As the baseline characteristics differed between treatment groups, we performed exact matching for various pathological factors according to RAI dose. We evaluated the response to therapy and recurrence-free survival (RFS) in the matched patients. Structural recurrent/persistent disease was defined as new structural disease detected after initial therapy, which was confirmed by cytology or pathology.
Results
Of the total 436 patients, 37 (8.5%) received 100 mCi of RAI and 399 (91.5%) received 150 mCi of RAI. After an exact 1:3 matching, 34 patients in the 100 mCi group and 100 patients in the 150 mCi group remained. There was no significant difference in response to therapy between the groups in the matched population (P=0.63). An excellent response was achieved in 70.6% (n=24) of patients in the 100 mCi group and 76.0% (n=76) in the 150 mCi group. Two (5.9%) patients in the 100 mCi group and four (4.0%) in the 150 mCi group had recurrence and there was no significant difference in RFS between the groups in the matched population (P=0.351).
Postoperative radioactive iodine (RAI) therapy has been used for patients with differentiated thyroid carcinoma (DTC) to remove residual normal thyroid tissue after thyroidectomy or to treat potential metastatic disease [1,2]. DTC patients presenting with lymph node (LN) metastasis have higher risks of distant metastasis and locoregional recurrence and lower remission rates compared with intrathyroidal tumors without clinical LN involvement [3]. Patients with LN metastasis were categorized as intermediate-risk group in the 2015 American Thyroid Association (ATA) guideline [1]. Furthermore, disease-specific mortality is higher in patients presenting with clinically significant LN metastasis [4–6].
The presence of lateral neck LN metastasis (N1b) is associated with a high rate of recurrence in papillary thyroid carcinoma (PTC) [7–9]. Furthermore, PTC patients with N1b disease showed inferior responses to surgery and RAI therapy compared to patients with only central neck LN metastasis (N1a) [10]. Thus, a high dose of RAI ablation/adjuvant therapy is routinely administered in PTC patients with N1b disease [11]. Recommendations for these patients often range from 100 to 250 mCi of RAI depending on the clinical situation, and most physicians treat N1b disease patients with 150 mCi of RAI [3,11]. However, the optimal dose of RAI therapy remains controversial and there is no sufficient evidence that a higher dose of RAI therapy is associated with improvement in clinical outcomes for patients with N1b disease. A recent study reported that administering more than 100 mCi of RAI as adjuvant therapy in N1b disease is unlikely to improve the initial response to therapy, especially in young patients [3].
Therefore, the aim of this study was to evaluate the clinical outcome of patients with N1b disease who were treated with either 100 or 150 mCi of RAI.
This study was a retrospective analysis of N1b PTC patients who underwent total thyroidectomy and postoperative RAI therapy at the Asan Medical Center (Seoul, South Korea) between 2012 and 2017. We included adult PTC patients with N1b disease confirmed at final pathology and who underwent either 100 or 150 mCi of RAI. Exclusion criteria were as follows: patients with distant metastasis initially; pathological diagnosis of follicular, medullary, poorly DTC, or anaplastic thyroid cancer; and patients without adequate follow-up data. Finally, 436 patients were included for analysis (Fig. 1). The study protocol was approved by the Institutional Review Board of the Asan Medical Center (study number: 2019-0676). Written informed consent by the patients was waived due to a retrospective nature of our study.
All patients underwent total thyroidectomy with central and lateral neck dissection. For RAI therapy, patients received ablative doses of 131I after thyroxine withdrawal or after two consecutive injections of recombinant human thyrotropin alpha (rhTSH). A post-therapy whole body scan (WBS) was obtained 5 to 7 days after administration of 131I. After RAI therapy, patients were regularly followed up with levothyroxine supplementation. Physical examinations, including neck palpation, thyroid function test, serum thyroglobulin (Tg), and anti-Tg antibody (TgAb) measurement, were done in all patients at each visit. To assess the response to therapy, a diagnostic WBS, stimulated Tg (sTg) with TgAb measurement, and neck ultrasonography (US) were done 6 to 12 months after RAI therapy [12–14]. The level of sTg was determined after thyroxine withdrawal or injection of rhTSH. After the response to therapy was assessed, neck US was performed every 12 to 24 months. If there were abnormal findings on neck US, US-guided fine-needle aspiration cytology was done. Other imaging studies, including neck/chest computed tomography or 18F-fluorodeoxyglucose-positron emission tomography, were performed when needed.
The risk of recurrence was determined by the ATA risk stratification system [1,15]. Intermediate-risk of recurrence was defined as microscopic tumor invasion into perithyroidal soft tissue, RAI-avid metastatic foci in the neck on the first posttreatment whole body RAI scan, aggressive histology, PTC with vascular invasion, clinical N1 or >5 pathologic N1 with all involved LNs <3 cm in largest dimension, and multifocal papillary microcarcinoma with extrathyroidal extension (ETE) and BRAFV600E mutated if known. High-risk of recurrence was defined as the macroscopic invasion of the tumor into the perithyroidal soft tissue (gross ETE), incomplete tumor resection, distant metastasis, postoperative serum Tg suggestive of distant metastasis, pathological N1 with any metastatic LNs ≥3 cm in the largest dimension or with extranodal extension (ENE) [1,15].
The response to initial therapy was classified according to the 2015 ATA management guidelines [1]: (1) excellent response if patients achieved sTg <1 ng/mL with negative TgAb and had no abnormal finding in neck US and diagnostic WBS; (2) indeterminate response if patients achieved sTg between 1 to 10 ng/mL or sTg was stable or declining in the absence of structural disease; (3) biochemical incomplete response if patients had sTg >10 ng/mL or rising TgAb levels in the absence of structural disease; or (4) structural incomplete response if patients had abnormal findings on imaging.
Structural recurrent/persistent disease was defined as the detection of new structural disease after initial therapy, which was confirmed by cytology or pathology. Recurrence-free survival (RFS) was defined as the time from total thyroidectomy to the detection of structural recurrent/persistent disease or the end of follow-up.
Statistical analyses were conducted using R version 3.5.1 (R Foundation for Statistical Computing, Vienna, Austria; http://www.R.project.org) and survival curves were drawn using GraphPad Prism version 5.0 (GraphPad Software, San Diego, CA, USA; http://www.graphpad.com). Continuous variables are presented as mean±standard deviation, and categorical variables are presented as number (percentage). We used the t test to compare continuous variables and the chi-square test to compare categorical variables. Survival curves were plotted using the Kaplan-Meier method, and the log-rank test was used to determine significance. We performed exact matching of patients according to RAI dose for further analysis. An exact 1:3 matching was performed for factors, the number and size of LNs, presence of ETE, and presence of ENE. LN numbers were matched using ±4 as an acceptable boundary, and the sizes of LNs were matched using ±1 cm as an acceptable boundary. The presence of ETE and ENE was exactly matched in both treatment groups. Differences were considered statistically significant at P<0.05.
Among the 436 patients included in this study, 37 (8.5%) received 100 mCi of RAI and 399 (91.5%) received 150 mCi of RAI. Baseline characteristics of these 436 patients are listed in Table 1. The mean age of the patients was 43.8 years and 272 patients (62%) were female. The mean tumor size was 2.1 cm and most had ETE: 24% with microscopic ETE and 56% with gross ETE. The mean number and size of metastatic LNs was 13.6 and 1.3 cm, respectively, and 57% showed ENE. Patients who received 150 mCi of RAI had more ETE (P=0.005), larger metastatic LNs (P<0.001), and more frequent ENE (P<0.001). There were more patients with T3 and T4 disease in the 150 mCi group (P=0.001), but there was no significant difference in TNM stage according to RAI dose. Among the total patients, 95 (21.8%) patients were classified as the intermediate-risk group by the ATA risk stratification, and 341 (78.2%) were classified as the high-risk group. There were more patients with a high-risk of recurrence in the 150 mCi group (P<0.001). In terms of surgical extent, 347 (79.6%) were underwent unilateral modified radical neck dissection (MRND) and others underwent bilateral MRND and there was no significant difference in surgical extent between two treatment groups (P=0.65). There was no significant difference between the extent of lateral LN metastasis in two groups (P=0.87). Five patients (1.1%) had residual tumor after surgery. All of the patients who received 150 mCi of RAI were prepared with levothyroxine withdrawal and 30 (81.1%) patients who treated with 100 mCi were prepared with rhTSH. At the time of RAI therapy, 15.9% of patients were positive for TgAb (reference <60 U/mL) and the median sTg among patients with negative TgAb was 2.10 ng/mL (range, 0.08 to 1,790.00). The proportion of patients with positive TgAb and median sTg levels was not significantly different between two groups (P=0.99 and P=0.40, respectively).
As the baseline characteristics were different between groups according to RAI dose, we performed exact matching for the pathologic characteristics as described in the methods. After matching, 34 patients in the 100 mCi group and 100 patients in the 150 mCi group remained (Fig. 1). The baseline characteristics of the matched 134 patients are shown in Table 2. After matching, the presence of ETE, number of LNs, size of LNs, and presence of ENE, which were all well-known risk factors for the recurrence of thyroid cancer, were not significantly different between the two groups. There was also no significant difference in T stage, ATA risk stratification and surgical extent between the two groups (Table 2).
The responses to initial therapy according to RAI dose in the total and matched populations are shown in Table 3. There were no significant differences in the response to therapy classification between the treatment groups in either total or matched patients (P=0.37 and P=0.63, respectively). In the matched population, 71% of patients (n=24) in the 100 mCi group and 76% (n=76) in the 150 mCi group achieved excellent response.
During a median follow-up period of 4.8 years (range, 0.5 to 8.1), 48 (11.0%) of the total 436 patients had structural recurrent/persistent disease: three patients (8.1%) in the 100 mCi group and 45 (11.3%) in the 150 mCi group. Of total 48 patients who had structural recurrent/persistent disease, 41 patients (9.4%) had locoregional metastasis, two patients (0.5%) had distant metastasis, and four (0.9%) had both locoregional and distant metastasis. The RFS curves are shown in Fig. 2A. RFS did not differ significantly according to RAI dose (P=0.76). The results were consistent in the matched population. Among the 134 matched patients, six (4.5%) had disease recurrence: two patients (5.9%) in the 100 mCi group and four (4.0%) in the 150 mCi group. The RFS curves of the matched patients are shown in Fig. 2B. There was no significant difference in RFS between the groups (P=0.35).
This was a retrospective study evaluating two different doses of RAI in N1b PTC patients. In the current study, 48 (11.0%) of the total 436 patients had structural recurrent/persistent disease after initial therapy, and the rate was similar with previous studies [1,3,16]. Because of differences in pathological characteristics between the groups, we evaluated the clinical outcomes of these patients after exact matching of various pathological risk factors. In both the total and matched cohorts, response to therapy classification and RFS was not different between 100 and 150 mCi of RAI therapy.
Recent studies demonstrated that low-dose RAI is sufficient for remnant ablation for low- to intermediate-risk DTC patients, and many experts advocate the use of lower doses of RAI [2,17–20]. In 2012, Schlumberger et al. [2] and Mallick et al. [17] compared 1.1 GBq (30 mCi) and 3.7 GBq (100 mCi) in DTC patients and reported similar rates of effectiveness and fewer adverse events in the lower RAI group than in the higher RAI group. The presence of N1b is an important prognostic factor for DTC patients, and a high dose of RAI for adjuvant therapy might be helpful [4,21]. However, patients undergoing high-dose RAI tend to have more adverse effects, such as sialadenitis, xerostomia, decreased gonadal function, and secondary neoplasm [22–25]. Considering the effectiveness and adverse effects, we need more accurate evidence for the efficacy of higher doses of RAI as a treatment option.
To our knowledge, there are no randomized controlled studies comparing the efficacy and safety of lower and higher doses of RAI in N1b PTC patients, and only a few retrospective studies have been reported [3,16]. A previous study involving 181 PTC patients with N1b disease who were treated with 100, 150 mCi, or high activity (>200 mCi) RAI therapy demonstrated that administering more than 100 mCi of RAI as adjuvant therapy is unlikely to improve the initial response to therapy, especially in young patients [3]. Another study involving 253 DTC patients with T4 or N1b disease demonstrated that adjuvant RAI therapy with rhTSH plus 3.7 GBq (100 mCi) had a comparable ablation success rate and RFS compared to thyroid hormone withdrawal plus 5.5 GBq (150 mCi) [16]. Our current study also showed similar results to previous studies. In the 134 matched patients with N1b disease in the current study, the response to therapy and RFS did not differ significantly between 100 mCi and 150 mCi of RAI therapy. This suggests that 100 mCi of RAI for N1b PTC patients is sufficient for remnant ablation. However, patients with more favorable pathological characteristics tended to remain after exact matching in our present study. The proportion of patients with ATA high-risk was 81.2% in 150 mCi group, and it was decreased to 51.0% after matching. Consequently, the proportion of patients with structural recurrent/persistent disease in 150 mCi group decreased from 11.3% to 4.0% after matching. Therefore, the result may be limited for the patients with more favorable pathologic findings and further studies for higher risk N1b disease are needed.
There were several limitations in the current study. First, it was a retrospectively designed study involving a small number of patients. Second, we did not investigate the adverse effects of different RAI administration strategies. Third, because the follow-up period was relatively short, there was no data about disease-specific or overall survival. Fourth, as we described above, the result of this study may be limited for the patients with more favorable pathologic findings. However, a strength of the current study is that it evaluated the effect of two different doses of RAI in N1b disease patients who were well-matched for tumor and LN factors.
In conclusion, there was no significant difference in response to therapy or RFS in PTC patients with N1b disease between 100 and 150 mCi of RAI therapy after exact matching of various pathological factors. These findings may help clinicians determine the optimal dose of RAI therapy in patients with N1b PTC.
Notes
AUTHOR CONTRIBUTIONS
Conception or design: M.J., J.A., Y.M.L., T.Y.S., W.G.K., T.Y.K., J.S.R., W.B.K., Y.K.S., M.J.J. Acquisition, analysis, or interpretation of data: M.J., J.A., Y.M.L., T.Y.S., W.G.K., T.Y.K., J.S.R., W.B.K., Y.K.S., M.J.J. Drafting the work or revising: M.J., M.J.J. Final approval of the manuscript: M.J., M.J.J.
ACKNOWLEDGMENTS
This study was supported by a grant (No. 2020IL0003) from the Asan Institute for Life Sciences, Asan Medical Center, Seoul, Korea.
REFERENCES
1. Haugen BR, Alexander EK, Bible KC, Doherty GM, Mandel SJ, Nikiforov YE, et al. 2015 American Thyroid Association management guidelines for adult patients with thyroid nodules and differentiated thyroid cancer: the American Thyroid Association guidelines task force on thyroid nodules and differentiated thyroid cancer. Thyroid. 2016; 26:1–133.


2. Schlumberger M, Catargi B, Borget I, Deandreis D, Zerdoud S, Bridji B, et al. Strategies of radioiodine ablation in patients with low-risk thyroid cancer. N Engl J Med. 2012; 366:1663–73.


3. Sabra MM, Grewal RK, Ghossein RA, Tuttle RM. Higher administered activities of radioactive iodine are associated with less structural persistent response in older, but not younger, papillary thyroid cancer patients with lateral neck lymph node metastases. Thyroid. 2014; 24:1088–95.


4. Podnos YD, Smith D, Wagman LD, Ellenhorn JD. The implication of lymph node metastasis on survival in patients with well-differentiated thyroid cancer. Am Surg. 2005; 71:731–4.


5. Zaydfudim V, Feurer ID, Griffin MR, Phay JE. The impact of lymph node involvement on survival in patients with papillary and follicular thyroid carcinoma. Surgery. 2008; 144:1070–7.


6. Leboulleux S, Rubino C, Baudin E, Caillou B, Hartl DM, Bidart JM, et al. Prognostic factors for persistent or recurrent disease of papillary thyroid carcinoma with neck lymph node metastases and/or tumor extension beyond the thyroid capsule at initial diagnosis. J Clin Endocrinol Metab. 2005; 90:5723–9.


7. Ito Y, Kudo T, Takamura Y, Kobayashi K, Miya A, Miyauchi A. Lymph node recurrence in patients with N1b papillary thyroid carcinoma who underwent unilateral therapeutic modified radical neck dissection. World J Surg. 2012; 36:593–7.


8. Baek SK, Jung KY, Kang SM, Kwon SY, Woo JS, Cho SH, et al. Clinical risk factors associated with cervical lymph node recurrence in papillary thyroid carcinoma. Thyroid. 2010; 20:147–52.


9. Lee SH, Roh JL, Gong G, Cho KJ, Choi SH, Nam SY, et al. Risk factors for recurrence after treatment of N1b papillary thyroid carcinoma. Ann Surg. 2019; 269:966–71.


10. Liu YQ, Li H, Liu JR, Lin YS. Unfavorable responses to radioiodine therapy in N1b papillary thyroid cancer: a propensity score matching study. Endocr Pract. 2019; 25:1286–94.


11. Beierwaltes WH, Rabbani R, Dmuchowski C, Lloyd RV, Eyre P, Mallette S. An analysis of “ablation of thyroid remnants” with I-131 in 511 patients from 1947–1984: experience at University of Michigan. J Nucl Med. 1984; 25:1287–93.
12. Jeon MJ, Kim WG, Park WR, Han JM, Kim TY, Song DE, et al. Modified dynamic risk stratification for predicting recurrence using the response to initial therapy in patients with differentiated thyroid carcinoma. Eur J Endocrinol. 2013; 170:23–30.


14. Yi KH. The revised 2016 Korean Thyroid Association guidelines for thyroid nodules and cancers: differences from the 2015 American Thyroid Association guidelines. Endocrinol Metab (Seoul). 2016; 31:373–8.


15. Lamartina L, Grani G, Durante C, Borget I, Filetti S, Schlumberger M. Follow-up of differentiated thyroid cancer: what should (and what should not) be done. Nat Rev Endocrinol. 2018; 14:538–51.


16. Jeong SY, Lee SW, Kim WW, Jung JH, Lee WK, Ahn BC, et al. Clinical outcomes of patients with T4 or N1b well-differentiated thyroid cancer after different strategies of adjuvant radioiodine therapy. Sci Rep. 2019; 9:5570.


17. Mallick U, Harmer C, Yap B, Wadsley J, Clarke S, Moss L, et al. Ablation with low-dose radioiodine and thyrotropin alfa in thyroid cancer. N Engl J Med. 2012; 366:1674–85.


18. Han JM, Kim WG, Kim TY, Jeon MJ, Ryu JS, Song DE, et al. Effects of low-dose and high-dose postoperative radioiodine therapy on the clinical outcome in patients with small differentiated thyroid cancer having microscopic extrathyroidal extension. Thyroid. 2014; 24:820–5.


19. Caglar M, Bozkurt FM, Akca CK, Vargol SE, Bayraktar M, Ugur O, et al. Comparison of 800 and 3700 MBq iodine-131 for the postoperative ablation of thyroid remnant in patients with low-risk differentiated thyroid cancer. Nucl Med Commun. 2012; 33:268–74.


20. Castagna MG, Cevenini G, Theodoropoulou A, Maino F, Memmo S, Claudia C, et al. Post-surgical thyroid ablation with low or high radioiodine activities results in similar outcomes in intermediate risk differentiated thyroid cancer patients. Eur J Endocrinol. 2013; 169:23–9.


21. Sellers M, Beenken S, Blankenship A, Soong SJ, Turbat-Herrera E, Urist M, et al. Prognostic significance of cervical lymph node metastases in differentiated thyroid cancer. Am J Surg. 1992; 164:578–81.


22. Rosario PW, Borges MA, Purisch S. Preparation with recombinant human thyroid-stimulating hormone for thyroid remnant ablation with 131I is associated with lowered radiotoxicity. J Nucl Med. 2008; 49:1776–82.
23. Kloos RT, Duvuuri V, Jhiang SM, Cahill KV, Foster JA, Burns JA. Nasolacrimal drainage system obstruction from radioactive iodine therapy for thyroid carcinoma. J Clin Endocrinol Metab. 2002; 87:5817–20.


Fig. 2
Kaplan-Meier analysis of recurrence-free survival according to different doses of radioactive iodine (RAI) therapy. (A) Recurrence-free survival in total 436 patients. (B) Recurrence-free survival in the matched 134 patients according to different doses of RAI.
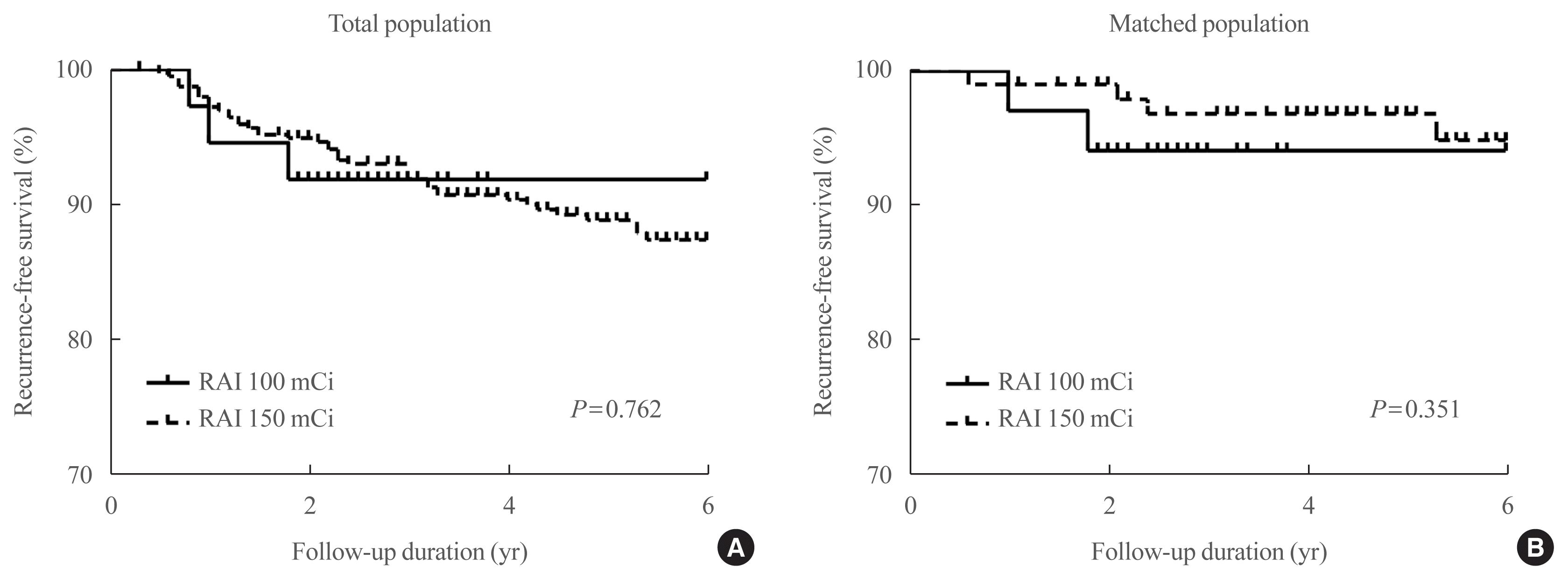
Table 1
Baseline Characteristics of Total 436 Patients
Characteristic | Total (n=436) | RAI 100 mCi (n=37) | RAI 150 mCi (n=399) | P value |
---|---|---|---|---|
Age, yr | 43.8±13.3 | 46.5±15.5 | 43.6±13.1 | 0.19 |
<55 | 337 (77.3) | 25 (67.6) | 312 (78.2) | 0.20 |
≥55 | 99 (22.7) | 12 (32.4) | 87 (21.8) | |
|
||||
Sex | 0.84 | |||
Male | 164 (37.6) | 15 (40.5) | 149 (37.3) | |
Female | 272 (62.4) | 22 (59.5) | 250 (62.7) | |
|
||||
Tumor size, cm | 2.1±1.2 | 2.1±0.7 | 2.1±1.2 | 0.91 |
≤2 | 281 (64.4) | 20 (54.1) | 261 (65.4) | 0.23 |
>2 | 155 (35.6) | 17 (45.9) | 138 (34.6) | |
|
||||
Presence of ETE | 0.005 | |||
No ETE | 88 (20.2) | 15 (40.5) | 73 (18.3) | |
Microscopic | 105 (24.1) | 8 (21.6) | 97 (24.3) | |
Gross | 243 (55.7) | 14 (37.8) | 229 (57.4) | |
|
||||
No. of metastatic LN | 13.6±8.6 | 13.4±7.9 | 13.6±8.6 | 0.93 |
|
||||
Size of metastatic LN, cm | 1.3±0.8 | 0.9±0.5 | 1.3±0.9 | <0.001 |
|
||||
Extent of lateral LN metastasis | 0.87 | |||
Unilateral | 355 (81.4) | 31 (83.8) | 324 (81.2) | |
Bilateral | 81 (18.6) | 6 (16.2) | 75 (18.8) | |
|
||||
Presence of ENE (yes) | 249 (57.1) | 6 (16.2) | 243 (60.9) | <0.001 |
|
||||
T stage | 0.001 | |||
T1 | 138 (31.7) | 13 (35.1) | 125 (31.3) | |
T2 | 44 (10.1) | 10 (27.0) | 34 (8.5) | |
T3 | 203 (46.6) | 13 (35.1) | 190 (47.6) | |
T4 | 51 (11.7) | 1 (2.7) | 50 (12.5) | |
|
||||
TNM stagea | 0.50 | |||
Stage I | 336 (77.2) | 25 (67.6) | 311 (78.1). | |
Stage II | 82 (18.9) | 11 (29.7) | 71 (17.8) | |
Stage III | 14 (3.2) | 1 (2.7) | 13 (3.3) | |
Stage IV | 3 (0.7) | 0 | 3 (0.8) | |
|
||||
ATA risk stratification | <0.001 | |||
Intermediate-risk of recurrence | 95 (21.8) | 20 (54.1) | 75 (18.8) | |
High-risk of recurrence | 341 (78.2) | 17 (45.9) | 324 (81.2) |
Table 2
Baseline Characteristics of the Matched 134 Patientsa
Characteristic | RAI 100 mCi (n=34) | RAI 150 mCi (n=100) | P value |
---|---|---|---|
Age, yr | 47.9±15.3 | 45.5±12.7 | 0.37 |
<55 | 22 (64.7) | 76 (76.0) | 0.29 |
≥55 | 12 (35.3) | 24 (24.0) | |
|
|||
Sex | 0.43 | ||
Male | 13 (38.2) | 29 (29.0) | |
Female | 21 (61.8) | 71 (71.0) | |
|
|||
Tumor size, cm | 2.1±0.7 | 2.0±1.1 | 0.59 |
≤2 | 17 (50.0) | 62 (62.0) | 0.30 |
>2 | 17 (50.0) | 38 (38.0) | |
|
|||
Presence of ETE | 0.98 | ||
No ETE | 14 (41.2) | 42 (42.0) | |
Microscopic | 6 (17.6) | 18 (18.0) | |
Gross | 14 (41.2) | 42 (42.0) | |
|
|||
No. of metastatic LN | 12.2±6.8 | 10.9±5.6 | 0.28 |
|
|||
Size of metastatic LN, cm | 0.9±0.5 | 0.9±0.5 | 0.96 |
|
|||
Extent of lateral LN metastasis | 0.79 | ||
Unilateral | 29 (85.3) | 89 (89.0) | |
Bilateral | 5 (14.7) | 11 (11.0) | |
|
|||
Presence of ENE (yes) | 6 (17.6) | 18 (18.0) | 0.99 |
|
|||
T stage | 0.31 | ||
T1 | 11 (32.4) | 38 (38.0) | |
T2 | 10 (29.4) | 15 (15.0) | |
T3 | 12 (35.3) | 42 (42.0) | |
T4 | 1 (2.9) | 5 (5.0) | |
|
|||
TNM stageb | 0.52 | ||
Stage I | 22 (64.7) | 76 (76.0) | |
Stage II | 11 (32.4) | 21 (21.0) | |
Stage III | 1 (2.9) | 2 (2.0) | |
Stage IV | 0 | 1 (1.0) | |
|
|||
ATA risk stratification | 0.84 | ||
Intermediate-risk of recurrence | 18 (52.9) | 49 (49.0) | |
High-risk of recurrence | 16 (47.1) | 51 (51.0) |
Table 3
Response to Therapy Classification in Total and Matched Patients