초록
다양한 치과용 도재가 개발되고 있으나 현재 단일구조 보철물로 사용할 수 있는 수준의 기계적, 광학적 성질을 보이는 도재는 반투명 지르코니아와 리튬디실리케이트 결정화유리가 대표적이다. 기계적 성질의 경우 지르코니아가, 광학적 성질은 리튬디실리케이트가 상대적으로 더 우수하다고 알려져 있으나 물성개선을 통해 보다 다양한 증례에 보철물을 적용할 수 있게 되었다. 그 결과 반투명 지르코니아와 리튬디실리케이트 보철물의 적응증이 서로 중복되는 경우가 발생하고 있다. 그러나 두 도재는 유리질(glass matrix) 포함여부뿐만 아니라 강화기전, 탄성계수 등이 서로다르다. 이에 본 문헌고찰에서는 두 단일구조 치과용 도재의 물성을 평가한 다양한 연구결과들을 살펴보고 특히 가공치가 포함된 고정성 보철물의 디자인 시 고려사항에 대해 알아보고자 한다.
Abstract
Although low translucency 3 mol% yttria stabilized tetragonal zirconia polycrystal has excellent mechanical properties, it has limited application as a monolithic prosthesis. To improve these optical limitations, translucent zirconia has improved esthetics due to an increase in the cubic phase; however, it is accompanied by a decrease in mechanical properties simultaneously. Lithium disilicate has improved its mechanical properties through crystal size reduction and various heat treatment methods; therefore, its clinical application range is continuously increasing. Translucent zirconia shows a wide distribution of physical properties depending on the yttria content and lithium disilicate according to the size and density of crystal grains. As a result, the indications for translucent zirconia and lithium disilicate are increasing. Therefore, in this literature review, we intend to examine the rationale behind the material selection criteria in clinical situations and considerations for designing fixed dental prostheses including pontic, in particular, by summarizing recent studies.
반투명 지르코니아(translucent zirconia)는 알루미나 함량을 줄이고 이트리아 함량을 증가시켜 심미성이 개선되었으나 입방정상(cubic phase)의 증가로 인한 기계적 성질의 감소를 동반하였다. 한편 대표적인 결정화유리인 리튬디실리케이트는 결정 크기 감소 및 밀도 증가를 통한 기계적 성질이 개선되면서 임상적 적용범위가 증가하였다. 그 결과 각각 광학적, 기계적 성질에서 상대적으로 우위를 보였던 리튬디실리케이트와 반투명 지르코니아간의 경계가 불명확해지고 있다. 그러나 두 재료는 탄성계수, 파절에 대한 저항기전, 유리질(glass matrix)의 유무에 따른 보철물 접착방법의 차이 등 재료학적 및 임상적으로 상이한 특징을 갖고 있다. 본 문헌고찰에서는 이러한 두가지 치과용 도재의 물성 차이에 대한 연구결과들을 정리하고 특히 가공치가 포함된 고정성 보철물의 디자인 시 고려사항에 대해 살펴보고자 한다.
치과용으로 처음 소개된 지르코니아는 3 mol%의 이트리아 안정화 정방정 지르코니아(3 mol% yttria stabilized tetragonal zirconia polycrystal; 3Y-TZP)로서 90% 이상이 정방정 결정형태로 이루어져 있어 기계적 성질은 우수하나 결정화유리에 비해 낮은 반투명도를 가진다.1 지르코니아의 반투명도는 입사광의 파장보다 큰 입자크기, 정방정 결정에 의한 복굴절(birefringence), 내부의 결함 및 불순물, 알루미나 입자의 분리 등에 의해 영향을 받는다.2-4 알루미나를 지르코니아에 0.5 - 1 wt% 가량 첨가하면 저온열화현상(low-temperature degradation; LTD)을 막고, 정방정의 지르코니아를 안정화하는 역할을 하지만 불투명도(opacity)가 증가한다.5,6 지르코니아의 반투명도를 개선하기 위해 알루미나 함량을 0.05 wt%까지 낮추는 방법이 개발되었지만, 대신 저온열화현상에 취약해지고 안정화 효과가 약해져 결과적으로 굴곡강도의 감소를 초래하였다.7 이트리아 함량에 따른 지르코니아의 굴곡강도를 비교한 연구에서 3Y-TZP는 약 1200 MPa의 굴곡강도를 가지고 있어 단일치관 혹은 고정성 보철물의형태로 교합력이 강한 구치부에서도 사용될 수 있고, 5Y-PSZ의 경우 3Y-TZP에 비해 30 - 40% 감소한 500 - 800 MPa의 굴곡강도를 보였다(Table 1).8
한편, 지르코니아의 반투명도는 결정입자크기(입도)와도 관련이 있다. 입도가 증가하면 일반적으로 반투명도 역시 증가한다.9 이트리아 함량이 높은 일부 반투명 지르코니아의 경우, 직경 1.5 µm 정도로 큰 입도를 가지며 반투명도가 우수하다.7 그러나 직경 1 µm 이상의 입도에서는 저온열화현상이 쉽게 발생하게 된다는 연구결과가 있으며,10 직경 1.4 µm까지는 입도 증가에 따라 지르코니아의 파절강도가 증가했지만, 그 이상일 때는 오히려 파절강도가 감소했다는 보고도 있어,11-13 입도 증가에 따른 지르코니아 강도의 감소에 대해서는 현재까지 명확한 경향이 밝혀지지 않은 상태이다.
지르코니아는 유리질이 배제된 다결정구조로 인해 기존의 실란결합제(silane coupling agent)를 이용한 레진시멘트와의 접착이 불가능하다. 따라서 기계적, 화학적 다양한 전처리 방법이 소개되었으며 이 가운데 3Y-TZP의 접착방법으로 50 µm, 2 bar 이하의 압력으로 공기매개입자마모(airborne-particle abrasion; APA) 처리 후 10-methacryloyloxydecyl dihydrogen phosphate monomer (10-MDP)를 포함한 전처리제를 적용하는 방법이추천되고 있다.3 그러나 McLaren 등14은 5Y-PSZ의 경우공기매개입자마모 처리 후 오히려 물성저하 및 기공과정에 더 민감하게 반응할 수 있음을 주장하였다.
이상의 내용을 정리하면 5Y-PSZ의 경우 심미성은 개선되지만 결정화유리와 비슷한 수준의 기계적 성질이 나타날 수 있다. 지르코니아 입도와 기계적 성질과의 연관성은 이트리아 함량 외에도 여러 요인이 영향을 미칠 수있어 추가 연구가 필요하다. 또한 지르코니아는 기존 치과용 도재에 비해 상대적으로 접착방법에 대한 장기간임상결과가 부족하며 일부 반투명 지르코니아의 전처리효과에 대해 아직 논란의 여지가 있으므로 임상에 적용시 보다 신중할 필요가 있다. 더구나 5Y-PSZ의 물성은 리튬디실리케이트와 유사하므로 상대적으로 접착기전이잘 정립된 결정화유리가 상황에 따라 5Y-PSZ보다 예지성 높은 재료 선택지가 될 수 있겠다.
리튬디실리케이트 결정화유리는 1990년대 출시된 IPS-Empress 2 (Ivoclar Vivadent AG, Schaan, Liechtenstein)가 대표적인 상품으로 알려져 있다. IPS-Empress 2는 작은 바늘모양의 리튬디실리케이트와 리튬오르토인산염(lithium orthophosphate) 결정(65%)이 유리질 내에 분포되어 있으며, 약 350 MPa의 굴곡강도를 보인다. 이후 더 작고 균일한 리튬디실리케이트 분포를 가지며, 굴곡강도가 370 - 460 MPa로 개선된 IPS e.max Press (Ivoclar Vivadent AG)가 출시되었고, 이 후 밀링방식으로 제작이 가능한 IPS e.max CAD (Ivoclar Vivadent AG)가 개발되었다.15 리튬디실리케이트는 유사한 강도를 가진 치과용 도재에 비해 상대적으로 높은 반투명도를 가진다.16 따라서 리튬디실리케이트는 하부구조나 전장도재 없이 단일구조관으로 사용이 가능하며, 전장관, 고정성 보철물, 비니어, 온레이 등에 널리 사용된다.17 그러나 상실치를 대체하는 고정성 보철물의 경우 전치, 소구치 부위의 3본(3-unit)으로 제한하고 있다.18
체계적 문헌고찰에 따르면, 리튬디실리케이트 단일치관은 85.3 - 100%의 생존율을 보이며, 보철물 탈락이나 파절 등의 합병증이 많지 않다고 하였다.19 그러나 3본 이상의 단일구조 리튬디실리케이트 고정성 보철물에 대한 장기간의 임상연구는 부족하며, 현재까지의 임상결과를 요약하면 Table 2와 같다.20-23
정리하면 리튬디실리케이트는 단일관에 비해 가공치가 포함된 고정성 보철물의 장기 임상연구가 부족하며,특히 연결부(connector)에서 잦은 파절이 발생하므로 연결부 단면적 및 디자인 시 제조사 권장사항 따르는 각별한 주의가 필요하다.
반투명 지르코니아의 물성에 관한 대부분의 연구에서기계적 성질은 리튬디실리케이트와 비슷하거나 양호할수 있지만, 광학적 성질은 리튬디실리케이트가 더 우수하다고 하였다.24-29 제조사에 따라 약간의 차이는 있으나 5Y-PSZ는 대략 500 - 700 MPa, 리튬디실리케이트는 약 250 - 400 MPa의 굴곡 강도를 보인다.13 그러나 시편의 형태, 지대치 종류와 접착, 하중조건 등에 따라 파절저항성의 측정값이 다를 수 있다. Yan 등26은 디스크 모양의 3Y-TZP, 4Y-PSZ, 5Y-PSZ 및 리튬디실리케이트 시편을 상아질 유사 기질(epoxy filled with woven glass fibers; NEMA grade G10)에 접착하여 파절강도와 반투명도를 비교하였다. 파절강도는 3Y-TZP, 4Y-PSZ, 리튬디실리케이트, 5Y-PSZ 순으로 우수하였으며, 반투명도는 5Y-PSZ와 리튬디실리케이트가 4Y-PSZ, 3Y-TZP보다 높은 것으로 나타났다. 저자는 보철물이 상아질에 접착될 때 하중에 저항하는 능력은 변화할 수 있으며, 탄성계수, 수복물의 두께, 접착에 사용된 시멘트 등에 의해 영향을 받을 수 있다고 하였다. Zadeh 등24은 반투명 지르코니아 6종과 리튬디실리케이트 시편의 굴곡강도와 파괴인성, 반투명도를 비교한 결과 굴곡강도, 파괴인성과 같은 재료 자체의 기계적 강도는 반투명 지르코니아가, 반투명도는 리튬디실리케이트가 더 우수하였지만, 와이블계수 측정 결과 두 재료의 신뢰도(reliability) 간에는 차이가 없다고 하였다.
Lawson 등25은 3Y-TZP, 5Y-PSZ, 리튬디실리케이트 단일구조관의 파절하중을 측정한 결과 3Y-TZP가 5383 N으로 가장 높았다. 그러나 5Y-PSZ는 3647 N, 리튬디실리케이트가 3768 N으로 두 재료 간 파절저항성의 차이는 없었다. 비록 5Y-PSZ 자체의 굴곡강도는 리튬디실리케이트에 비해 약 두 배 이상 높았으나, 복합레진 지대주와 보철물 간 탄성계수 부조화 및 접착력이 실험결과에 영향을 준 것으로 저자는 분석하였다. Choo 등30도 2종의 5Y-PSZ (Lava Esthetic, 3M ESPE, St. Paul, USA; Katana UTML, Kuraray Noritake Dental Inc., Tokyo, Japan)와 2종의 리튬디실리케이트(e.max CAD, Ivoclar Vivadent AG; Amber Mill, HASS, Gangneung, Korea)
상악전치부 단일관의 동적하중 후 파절저항성을 비교하였다. 5Y-PSZ와 리튬디실리케이트 간의 파절저항성의 차이가 없었으며 오히려 일부 리튬디실리케이트(Amber Mill, HASS)에서 5Y-PSZ (Katana UTML, Kuraray Noritake Dental Inc.)보다 더 높은 파절저항성을 보였는데 이러한 원인으로 레진시멘트와의 결합력의 차이, 지르코니아와 지대주 간의 높은 탄성계수 부조화를 언급하였다.
기존 여러 연구들에서 지대주의 탄성계수는 고정성 보철물의 파절저항성에 영향을 미치며 지대주의 탄성계수증가가 보철물의 파절저항성을 개선시킨다고 하였다.31-33 실제 지르코니아와 리튬디실리케이트 보철물의 임상적환경은, 임플란트용 티타늄합금 지대주 또는 상아질로 이루어진 지대치에 적용되는 경우가 대부분이다. 법랑질의 탄성계수는 8.2 - 95.8 GPa, 상아질은 5.3 - 13.3 GPa 정도로 낮은 반면,34 티타늄합금은 약 115 GPa, 지르코니아는 약 210 GPa, 리튬디실리케이트는 약 60.1 GPa의 탄성계수를 갖고있다.35,36 따라서 상아질과 지르코니아 조합 시 탄성계수 부조화가 두드러질 수 있다.
그러나 실질적인 반투명 지르코니아 보철물의 생존율과 성공률에 대한 장기간의 임상연구는 부족한 실정이다. Gardell 등37은 반투명 지르코니아와 리튬디실리케이트 단일구조관의 3년간 추적관찰연구를 시행한 결과 리튬디실리케이트는 3년간 100%의 생존율, 89.7%의 성공률을 보였으며, 지르코니아는 93.3%의 생존율과 84.7%의 성공률을 보여 두 재료 간 유의한 차이가 없다고 하였다. 반면 3Y-TZP의 경우 50개의 구치부 단일구조관을 대상으로 한 5년간의 전향적 연구에서는 98%의 생존율과 94%의 성공률이 보고되었다.38
정리하면 반투명 지르코니아는 기존의 3Y-TZP 보다 낮은 기계적 성질을 보이며, 특히 5Y-PSZ와 같이 이트리아 함량이 많은 경우 이러한 경향이 뚜렷함을 여러 연구에서 확인할 수 있었다. 또한 이트리아 함량과 무관하게높은 지르코니아의 탄성계수와 이트리아 함량에 따른 반투명 지르코니아의 물성 변화가 실제 구강 내 기능 시 미치는 영향에 관한 추가 연구가 필요하다.
하나 이상의 상실치를 수복하는 고정성 보철물의 제작시, 재료의 물성과 더불어 보철물의 연결부 디자인 또한 예후를 결정하는 요소이다.39 고정성 보철물의 연결부는 불규칙한 형태의 빔(beam)의 가장 얇은 부분에 해당하므로 보철물에서 가장 취약하다.40 교합력이 가해지면, 고정성 보철물에 굽힘 모멘트가 발생하며, 하부 연결부에는 인장응력이 발생하는데 이 때, 인장에 의한 단면적의 수축을 고려하지 않은 응력을 공칭응력(σnom, nominal stress)라고 한다. 반면, 인장에 의해 연결부 단면적은 급격하게 감소하게 되고, 연결부의 뿌리 부분에는 공칭 응력을 넘어서는 최대응력(σmax, maximum stress)이 발생하게 된다. 응력집중(Kt, stress concentration)은 공칭응력에 대한 최대응력의 크기로 정의되며, 연결부 직경(d), 가공치의 직경(D), 그리고 연결부 곡률의 직경(ρ) 등에 의해 영향을 받을 수 있다(Fig. 1).41
이러한 연결부의 적절한 크기 및 디자인에 대한 다양한연구가 있다.41-44 3Y-TZP의 경우 대구치에도 사용할 수 있으며, 9 mm2 이상의 연결부 면적이 추천된다.42 Larsson 등43은 연결부 직경을 다르게 하여 고정성 보철물의 파절 하중을 측정하여 2 mm와 2.5 mm의 직경을 가진 보철물에 30 - 300 N의 전하중을 가하였을 때 모두 파절되었음을 보고하며 3Y-TZP 일지라도 충분한 연결부 직경의 필요성을 주장하였다. Spies 등44은 지르코니아 4본 고정성 보철물의 파절하중을 비교한 결과 5Y-PSZ는 3Y-TZP, 4Y-PSZ에 비해 낮은 파절하중을 보였고 특히 변연에서 기시된 편파절(crack)이 주로 발생하여 4본 고정성 보철물로 적절하지 않다고 하였다. 일반적으로 동일한 연결부 단면적이라면 연결부의 협설 폭보다는 수직높이가 더 중요한 것으로 알려져 있다.45,46 리튬디실리케이트의 경우 최소 16 mm2 이상의 연결부 단면적이 필요하며 소구치까지로 사용이 제한된다(Table 3). Junker 등47은 리튬디실리케이트 3본 고정성 보철물의 경우 9 mm2, 12 mm2의 연결부 단면적을 갖는 경우 파절저항성 차이가 없으나 16 mm2의 연결부 단면적을 갖는 보철물은 더 높은 파절저항성을 보고하였다.
연결부 곡률의 직경에 의해서도 고정성 보철물의 강도는 영향을 받을 수 있다. Hamza 등48은 연결부 곡률의 직경이 0.6 mm인 것을 ‘round design’, 직경이 0.1 mm인 것을 ‘sharp design’로 구분하고, 각각 2 mm, 3 mm 직경의 연결부를 가지도록 디자인하였다. ‘round design’은 ‘sharp design’에 비해 높은 굽힘강도를 보였고, 2 mm 지경의 ‘sharp design’에서 가장 낮은 굽힘강도가 측정되었다. 이와 유사한 연구49에서도, 연결부 곡률의 직경이 큰(즉, 둥근 형태의 연결부를 가진) 경우 높은 강도를 보이며 응력을 견디기에 더 유리하였다.
정리하면 반투명 지르코니아 고정성 보철물의 파절저항성은 이트리아 함량에 따라 연결부 및 가공치 단면적의 넓이의 기준이 다르게 적용되어야 하며 특히 5Y-PSZ의 경우 리튬디실리케이트와 유사한 기준이 필요하다. 또한 동일한 연결부 단면적일지라도 폭보다는 높이 확보
가 더 중요하며, 가급적 완만한 연결부 곡률의 디자인 형성이 파절방지에 보다 유리하다.
반투명 지르코니아는 기존 지르코니아(3Y-TZP)에 비해 광학적 성질은 우수하지만 기계적 성질의 감소를 동반한다. 이러한 경향은 이트리아 함량이 증가할수록 더 뚜렷하여 5Y-PSZ는 리튬디실리케이트 결정화유리와 유사한 반투명도와 파절저항성을 나타낸다. 따라서 5Y-PSZ를 이용한 보철물 디자인 및 적응증은 기존 지르코니아보다는 리튬디실리케이트처럼 보다 보수적인 접근이 필요하다. 지르코니아 보철물과 자연치 지대치간의발생하는 탄성계수 부조화가 실제 구강 내 기능 시 미치는 영향에 대해서는 추가 연구가 필요하다. 5Y-PSZ 고정성 보철물의 연결부 파절을 방지하기 위해서는 연결부의완만한 형태뿐만 아니라 리튬디실리케이트와 유사한 수준의 연결부 단면적과 더불어 충분한 연결부 높이가 확보되어야 한다.
References
1. Ghodsi S, Jafarian Z. 2018; A review on translucent zirconia. Eur J Prosthodont Restor Dent. 26:62–74. DOI: 10.1922/EJPRD_01759Ghodsi13. PMID: 29797847.
2. Zhang Y. 2014; Making yttria-stabilized tetragonal zirconia translucent. Dent Mater. 30:1195–203. DOI: 10.1016/j.dental.2014.08.375. PMID: 25193781. PMCID: PMC4167579.


3. Sulaiman TA, Abdulmajeed AA, Donovan TE, Ritter AV, Vallittu PK, Närhi TO, Lassila LV. 2015; Optical properties and light irradiance of monolithic zirconia at variable thicknesses. Dent Mater. 31:1180–7. DOI: 10.1016/j.dental.2015.06.016. PMID: 26198027.


4. Baldissara P, Wandscher VF, Marchionatti AME, Parisi C, Monaco C, Ciocca L. 2018; Translucency of IPS e.max and cubic zirconia monolithic crowns. J Prosthet Dent. 120:269–75. DOI: 10.1016/j.prosdent.2017.09.007. PMID: 29475752.


5. Hallmann L, Ulmer P, Reusser E, Louvel M, Hämmerle C. 2012; Effect of dopants and sintering temperature on microstructure and low temperature degradation of dental Y-TZP-zirconia. J Eur Ceram Soc. 32:4091–104. DOI: 10.1016/j.jeurceramsoc.2012.07.032.


6. Nakamura T, Nakano Y, Usami H, Wakabayashi K, Ohnishi H, Sekino T, Yatani H. 2016; Translucency and low-temperature degradation of silica-doped zirconia: A pilot study. Dent Mater J. 35:571–7. DOI: 10.4012/dmj.2015-274. PMID: 27477222.


7. Zhang Y, Lawn BR. 2018; Novel zirconia materials in dentistry. J Dent Res. 97:140–7. DOI: 10.1177/0022034517737483. PMID: 29035694. PMCID: PMC5784474.


8. Zhang F, Inokoshi M, Batuk M, Hadermann J, Naert I, Van Meerbeek B, Vleugels J. 2016; Strength, toughness and aging stability of highly-translucent Y-TZP ceramics for dental restorations. Dent Mater. 32:e327–37. DOI: 10.1016/j.dental.2016.09.025. PMID: 27697332.


9. Stawarczyk B, Ozcan M, Hallmann L, Ender A, Mehl A, Hämmerlet CH. 2013; The effect of zirconia sintering temperature on flexural strength, grain size, and contrast ratio. Clin Oral Investig. 17:269–74. DOI: 10.1007/s00784-012-0692-6. PMID: 22358379.


10. Bravo-Leon A, Morikawa Y, Kawahara M, Mayo MJ. 2002; Fracture toughness of nanocrystalline tetragonal zirconia with low yttria content. Acta Materialia. 50:4555–62. DOI: 10.1016/S1359-6454(02)00283-5.


11. Wang J, Rainforth M, Stevens R. Meriani S, Palmonari C, editors. 1989. The grain size dependence of the mechanical properties in TZP ceramics. Zirconia' 88: Advances in Zirconia Science and Technology. Springer Netherlands;Dordrecht: p. 337–66. DOI: 10.1007/978-94-009-1139-0_31.


12. Sulaiman TA. 2020; Materials in digital dentistry-A review. J Esthet Restor Dent. 32:171–81. DOI: 10.1111/jerd.12566. PMID: 31943720.


13. Holman CD, Lien W, Gallardo FF, Vandewalle KS. 2020; Assessing flexural strength degradation of new cubic containing zirconia materials. J Contemp Dent Pract. 21:114–8. DOI: 10.5005/jp-journals-10024-2762. PMID: 32381812.


14. McLaren EA, Lawson N, Choi J, Kang J, Trujillo C. 2017; New high-translucent cubic-phase-containing zirconia: Clinical and laboratory considerations and the effect of air abrasion on strength. Compend Contin Educ Dent. 38:e13–6. PMID: 28586235.
15. Zarone F, Di Mauro MI, Ausiello P, Ruggiero G, Sorrentino R. 2019; Current status on lithium disilicate and zirconia: a narrative review. BMC Oral Health. 19:134. DOI: 10.1186/s12903-019-0838-x. PMID: 31272441. PMCID: PMC6610968. PMID: bfc46b06676d444baad3dcf6a074e8f1. PMID: bfc46b06676d444baad3dcf6a074e8f1.


16. Zhang F, Reveron H, Spies BC, Van Meerbeek B, Chevalier J. 2019; Trade-off between fracture resistance and translucency of zirconia and lithium-disilicate glass ceramics for monolithic restorations. Acta Biomater. 91:24–34. DOI: 10.1016/j.actbio.2019.04.043. PMID: 31034947.


17. Sulaiman TA, Abdulmajeed AA, Delgado A, Donovan TE. 2020; Fracture rate of 188695 lithium disilicate and zirconia ceramic restorations after up to 7.5 years of clinical service: A dental laboratory survey. J Prosthet Dent. 123:807–10. DOI: 10.1016/j.prosdent.2019.06.011. PMID: 31703926.


18. Laksono H. 2007; The clinical potential and limits of the all-ceramic fixed partial denture restorations. Dental Journal. 40:186–92. DOI: 10.20473/j.djmkg.v40.i4.p186-192.


19. Mazza LC, Lemos CAA, Pesqueira AA, Pellizzer EP. 2021; Survival and complications of monolithic ceramic for tooth-supported fixed dental prostheses: A systematic review and meta-analysis. J Prosthet Dent. S0022-3913(21)00065-2. DOI: 10.1016/j.prosdent.2021.01.020. PMID: 33745685.


20. Garling A, Sasse M, Becker MEE, Kern M. 2019; Fifteenyear outcome of three-unit fixed dental prostheses made from monolithic lithium disilicate ceramic. J Dent. 89:103178. DOI: 10.1016/j.jdent.2019.08.001. PMID: 31394121.


21. Reich S, Endres L, Weber C, Wiedhahn K, Neumann P, Schneider O, Rafai N, Wolfart S. 2014; Three-unit CAD/CAM-generated lithium disilicate FDPs after a mean observation time of 46 months. Clin Oral Investig. 18:2171–8. DOI: 10.1007/s00784-014-1191-8. PMID: 24493230.


22. Kern M, Sasse M, Wolfart S. 2012; Ten-year outcome of three-unit fixed dental prostheses made from monolithic lithium disilicate ceramic. J Am Dent Assoc. 143:234–40. DOI: 10.14219/jada.archive.2012.0147. PMID: 22383203.


23. Makarouna M, Ullmann K, Lazarek K, Boening KW. 2011; Six-year clinical performance of lithium disilicate fixed partial dentures. Int J Prosthodont. 24:204–6. PMID: 21519566.
24. Zadeh PN, Lümkemann N, Sener B, Eichberger M, Stawarczyk B. 2018; Flexural strength, fracture toughness, and translucency of cubic/tetragonal zirconia materials. J Prosthet Dent. 120:948–54. DOI: 10.1016/j.prosdent.2017.12.021. PMID: 29807742.


25. Lawson NC, Jurado CA, Huang CT, Morris GP, Burgess JO, Liu PR, Kinderknecht KE, Lin CP, Givan DA. 2019; Effect of surface treatment and cement on fracture load of traditional zirconia (3Y), translucent zirconia (5Y), and lithium disilicate crowns. J Prosthodont. 28:659–65. DOI: 10.1111/jopr.13088. PMID: 31145492. PMCID: PMC6642729.


26. Yan J, Kaizer MR, Zhang Y. 2018; Load-bearing capacity of lithium disilicate and ultra-translucent zirconias. J Mech Behav Biomed Mater. 88:170–5. DOI: 10.1016/j.jmbbm.2018.08.023. PMID: 30173069. PMCID: PMC6179910.


27. Heintze SD, Monreal D, Reinhardt M, Eser A, Peschke A, Reinshagen J, Rousson V. 2018; Fatigue resistance of all-ceramic fixed partial dentures - Fatigue tests and finite element analysis. Dent Mater. 34:494–507. DOI: 10.1016/j.dental.2017.12.005. PMID: 29395474.


28. Kim HK. 2020; Optical and mechanical properties of highly translucent dental zirconia. Materials. 13:3395. DOI: 10.3390/ma13153395. PMID: 32751942. PMCID: PMC7435650. PMID: 5291091c6c4543b7bc40eb9cc2ab8670. PMID: 5291091c6c4543b7bc40eb9cc2ab8670.


29. Cho YE, Lim YJ, Han JS, Yeo IS, Yoon HI. 2020; Effect of yttria content on the translucency and masking ability of yttria-stabilized tetragonal zirconia polycrystal. Materials. 13:4726. DOI: 10.3390/ma13214726. PMID: 33105902. PMCID: PMC7660169. PMID: e89f00a2a2964cb2906ceca10d7c27d7. PMID: e89f00a2a2964cb2906ceca10d7c27d7.


30. Choo SS, Ko KH, Huh YH, Park CJ, Cho LR. 2021; Fatigue resistance of anterior monolithic crowns produced from CAD-CAM materials: An in vitro study. J Prosthet Dent. S0022-3913(21)00568-0. DOI: 10.1016/j.prosdent.2021.09.034. PMID: 34980473.


31. Fischer H, Weber M, Eck M, Erdrich A, Marx R. 2004; Finite element and experimental analyses of polymer-based dental bridges reinforced by ceramic bars. J Biomech. 37:289–94. DOI: 10.1016/j.jbiomech.2003.08.013. PMID: 14757447.


32. Mahmood DJ, Linderoth EH, Vult von Steyern P. 2011; The influence of support properties and complexity on fracture strength and fracture mode of allceramic fixed dental prostheses. Acta Odont Scand. 69:229–37. DOI: 10.3109/00016357.2010.549508. PMID: 21231816.


33. Scherrer SS, de Rijk WG. 1993; The fracture resistance of all-ceramic crowns on supporting structures with different elastic moduli. Int J Prosthodont. 6:462–7. PMID: 8297457.
34. Chun KJ, Choi HH, Lee JY. 2014; Comparison of mechanical property and role between enamel and dentin in the human teeth. J Dent Biomech. 5:1758736014520809. DOI: 10.1177/1758736014520809. PMID: 24550998. PMCID: PMC3924884.


35. Elsaka SE, Elnaghy AM. 2016; Mechanical properties of zirconia reinforced lithium silicate glass-ceramic. Dent Mater. 32:908–14. DOI: 10.1016/j.dental.2016.03.013. PMID: 27087687.


36. Pereira GKR, Graunke P, Maroli A, Zucuni CP, Prochnow C, Valandro LF, Caldas RA, Bacchi A. 2019; Lithium disilicate glass-ceramic vs translucent zirconia polycrystals bonded to distinct substrates: Fatigue failure load, number of cycles for failure, survival rates, and stress distribution. J Mech Behav Biomed Mater. 91:122–30. DOI: 10.1016/j.jmbbm.2018.12.010. PMID: 30579109.


37. Gardell E, Larsson C, von Steyern PV. 2021; Translucent zirconium dioxide and lithium disilicate: A 3-year follow-up of a prospective, practice-based randomized controlled trial on posterior monolithic crowns. Int J Prosthodont. 34:163–72. DOI: 10.11607/ijp.6795. PMID: 33882563.


38. Solá-Ruiz MF, Baixauli-López M, Roig-Vanaclocha A, Amengual-Lorenzo J, Agustín-Panadero R. 2021; Prospective study of monolithic zirconia crowns: clinical behavior and survival rate at a 5-year follow-up. J Prosthodont Res. 65:284–90. DOI: 10.2186/jpr.JPR_D_20_00034. PMID: 33041280.


39. Ambré MJ, Aschan F, Vult von Steyern P. 2013; Fracture strength of yttria-stabilized zirconium-dioxide (Y-TZP) fixed dental prostheses (FDPs) with different abutment core thicknesses and connector dimensions. J Prosthodont. 22:377–82. DOI: 10.1111/jopr.12003. PMID: 23289522.


40. Motta AB, Pereira LC, da Cunha AR, Duda FP. 2008; The influence of the loading mode on the stress distribution on the connector region of metal-ceramic and all-ceramic fixed partial denture. Artif Organs. 32:283–91. DOI: 10.1111/j.1525-1594.2008.00544.x. PMID: 18370942.


41. Quinn GD, Studart AR, Hebert C, VerHoef JR, Arola D. 2010; Fatigue of zirconia and dental bridge geometry: Design implications. Dent Mater. 26:1133–6. DOI: 10.1016/j.dental.2010.07.014. PMID: 20813401. PMCID: PMC2967591.


42. Lohbauer U, Amberger G, Quinn GD, Scherrer SS. 2010; Fractographic analysis of a dental zirconia framework: a case study on design issues. J Mech Behav Biomed Mater. 3:623–9. DOI: 10.1016/j.jmbbm.2010.07.004. PMID: 20826369. PMCID: PMC3086638.


43. Larsson C, Holm L, Lövgren N, Kokubo Y, Vult von Steyern P. 2007; Fracture strength of four-unit Y-TZP FPD cores designed with varying connector diameter. An in-vitro study. J Oral Rehabil. 34:702–9. DOI: 10.1111/j.1365-2842.2007.01770.x. PMID: 17716270.


44. Spies BC, Zhang F, Wesemann C, Li M, Rosentritt M. 2020; Reliability and aging behavior of three different zirconia grades used for monolithic four-unit fixed dental prostheses. Dent Mater. 36:e329–39. DOI: 10.1016/j.dental.2020.08.002. PMID: 32948330.


45. Takuma Y, Nomoto S, Sato T, Sugihara N. 2013; Effect of framework design on fracture resistance in zirconia 4-unit all-ceramic fixed partial dentures. Bull Tokyo Dent Coll. 54:149–56. DOI: 10.2209/tdcpublication.54.149. PMID: 24334628.


46. Murase T, Nomoto S, Sato T, Shinya A, Koshihara T, Yasuda H. 2014; Effect of connector design on fracture resistance in all-ceramic fixed partial dentures for mandibular incisor region. Bull Tokyo Dent Coll. 55:149–55. DOI: 10.2209/tdcpublication.55.149. PMID: 25212560.


47. Junker R, Höller M, Yoshida-Anastasova Y, Frank W, Nothdurft FP. 2019; Influence of connector diameter on fracture load of CAD/CAM-processed monolithic lithium disilicate fixed partial dentures. Int J Prosthodont. 32:68–70. DOI: 10.11607/ijp.5955. PMID: 30677115.


48. Hamza TA, Attia MA, El-Hossary MM, Mosleh IE, Shokry TE, Wee AG. 2016; Flexural strength of small connector designs of zirconia-based partial fixed dental prostheses. J Prosthet Dent. 115:224–9. DOI: 10.1016/j.prosdent.2015.06.022. PMID: 26547519.


49. Plengsombut K, Brewer JD, Monaco EA Jr, Davis EL. 2009; Effect of two connector designs on the fracture resistance of all-ceramic core materials for fixed dental prostheses. J Prosthet Dent. 101:166–73. DOI: 10.1016/S0022-3913(09)60022-6. PMID: 19231568.


Fig. 1
Schematic diagram of fixed partial denture and connector.41 (A) 4-unit fixed dental prosthesis (FPD), (B) Compressive and tensile stress in connector area.
d, connector diameter; D, diameter at the pontics; ρ, radius of curvature; σnom, nominal stress; M, Moment.
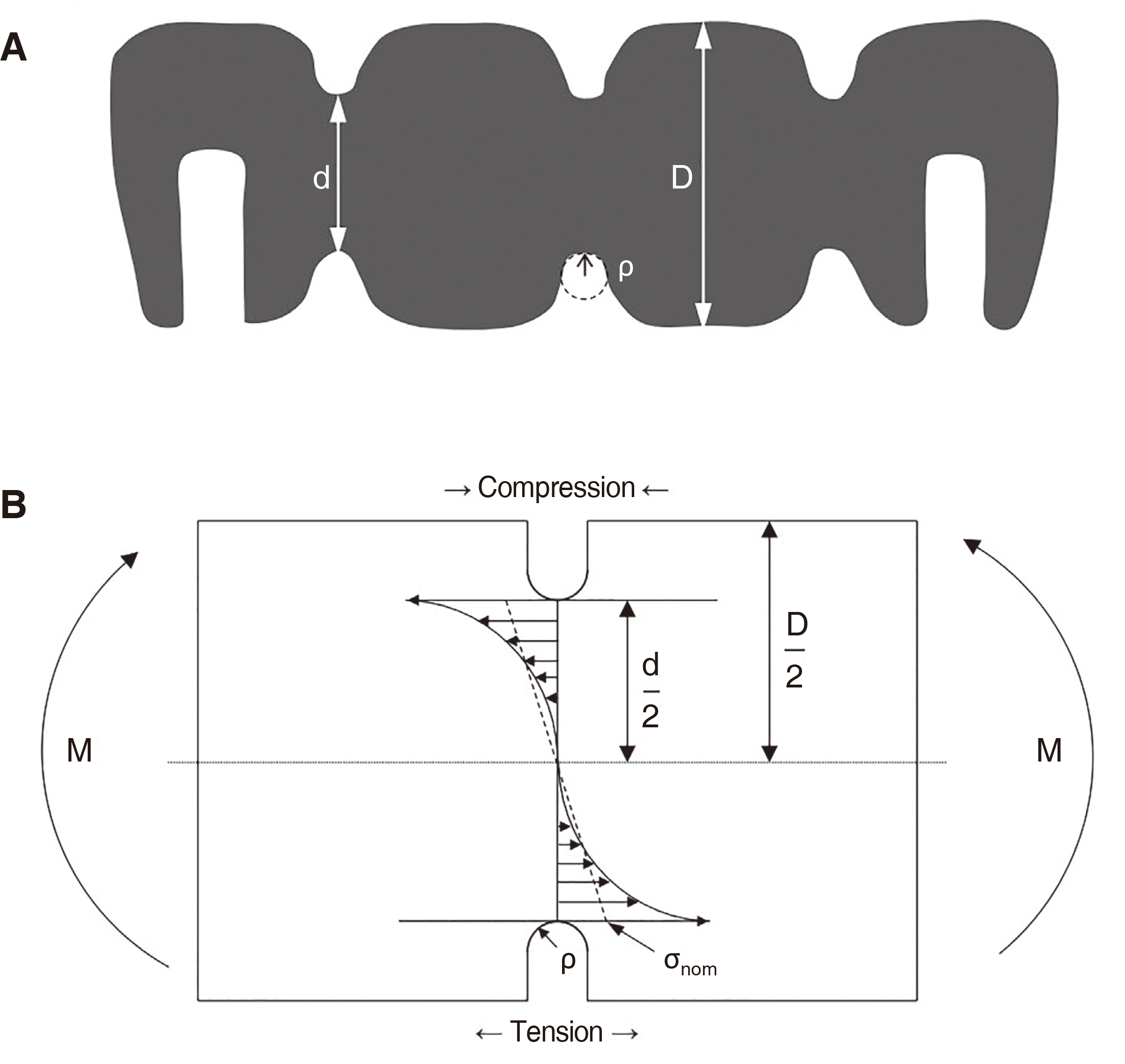
Table 1
Mechanical properties of representative dental zirconia ceramics8
Table 2
Clinical study on the prognosis of monolithic lithium disilicate FPDs
Garling et al.20 | Reich et al.21 | Kern et al.22 | Makarouna et al.23 | |
---|---|---|---|---|
Study design | Prospective | Prospective | Prospective | RCT |
Patient/Prosthesis | 28/36 | 32/32 | 28/36 | 18/18 |
Area | Ant/Post | Ant/Post | Ant/Post | Ant/Post |
Biologic failure | Not reported | Endodontic treatment (3/36) | Periodontal disease (1/36) | |
Endodontic treatment (2/36) | ||||
Technical failure | Fracture of Restoration (6/36) | Fracture of restoration (3/36) | Debonding (2/36) | Fracture of restoration (6/18) |
Fracture of restoration (5/36) | ||||
Follow up | 167 months | 2 years | 5 years/10 years | 6 years |
Survival rate (%) | 66.6% | 93% | 100%/87.9% | 62.7% |
Success rate (%) | - | 83% | 91.1%/69.8% | - |
Table 3
Manufacturer’s recommendation for minimum connector size of all-ceramic FPDs