Abstract
The orexin 2 receptor plays a central role in maintaining sleep and wakefulness. Recently, it has been shown that sleep and wakefulness orchestrate the proliferation and differentiation of oligodendrocytes. Here, we explored the role of a selective orexin 2 receptor antagonist (JNJ-10397049) in proliferation and differentiation of neural progenitor cells (NPCs). We evaluated the proliferation potential of NPCs after exposure to different concentrations of JNJ-10397049 by using 3-(4,5-dimethylthiazol-2-yl)-2,5 diphenyl tetrazolium bromide and neurosphere assays. Moreover, the expression of differentiation markers was assessed by immunocytochemistry and real-time polymerase chain reaction. JNJ-10397049 significantly increased the proliferation of NPCs at lower concentrations. In addition, orexin 2 receptor antagonist facilitated progression of differentiation of NPCs towards oligodendroglial lineage by considerable expression of Olig2 and 2’,3’-cyclic-nucleotide 3’-phosphodiesterase as well as decreased expression of nestin marker. The results open a new avenue for future investigations in which the production of more oligodendrocytes from NPCs is needed.
Neural stem cells (NSCs), and their progenies, collectively known as neural precursor cells (NPCs) are known as remarkable reservoir for replacing lost and damaged cells in the central nervous system (CNS) [1]. NPCs are capable of giving rise to astrocytes, neurons and oligodendrocytes in the CNS and have been repeatedly reported to be powerful players in the recovery of various neurodegenerative diseases [2, 3]. Demyelination is a destructive phenomenon commonly present in many neurodegenerative diseases such as multiple sclerosis (MS) [4, 5], spinal cord injury (SCI) [6] and Alzheimer’s disease [7, 8]. NPCs in the adult brain and oligodendrocyte precursor cells (OPCs) present around demyelinated lesions are sources for generation of new myelinating oligodendrocytes [9, 10]. Remyelination is generation of myelin sheath by newly formed mature oligodendrocytes after demyelination. It is a natural mechanism evident in healthy adult brain. In illnesses such as MS the microenvironment of demyelinated lesions halts remyelination due to the presence of inhibitory factors [4, 11, 12]. In these instances, remyelination can be activated endogenously by providing a permissive environment for generation of new myelinating oligodendrocytes or by cell transplantation. NPCs and oligodendrocyte progenitor cells are affected by several factors such as sleep/wakefulness cycles through the action of neurotransmitters, agents and hormones associated to sleep. The effect of several sleep/wakefulness agents such as melatonin, serotonin, and acetylcholine are known on OPCs and NPCs [13-17].
Orexin/hypocretin pathway is also involved in sleep/wakefulness. Orexin A and orexin B are neuropeptides synthesized in neurons originating from the lateral hypothalamus and bind to their cognate receptors, orexin receptor type 1 (OX1R, or Hcrtr-1) and orexin receptor type 2 (OX2R, or Hcrtr-2) [18, 19]. Orexin 2 receptor is part of the orexin/hypocretin signaling pathway that is involved in many physiological phenomena, such as sleep, feeding behavior, reward system and stress response [18, 20, 21]. On the other hand, orexin and orexin receptors are also ectopically expressed in different neurological disorders implying that orexin/hypocretin signaling pathway plays critical role in the pathogenesis of neurological diseases [22, 23]. Orexin and its receptors also regulate sleep/wakefulness agents such as histamine, melatonin, and serotonin [24-28] and may have a role in oligodendrogeneis or remyelination induction or inhibition similar to the aforementioned neurotransmitters involved in sleep/wakefulness. There has not been any study investigating its potential role in oligodendrogenesis. Orexin pathway is targeted by several commercial drugs treating insomnia and therefore, deciphering the role of orexin receptors in oligodendrogenesis can lead to possibly repurposing these marketed drugs for induction of remyelination and simultaneously improve sleep in patients with demyelinating diseases such as MS. Here we will only focus on the effect of a selective orexin 2 receptor inhibitor called JNJ-10397049.
We investigated the effect of JNJ-10397049 on NPC proliferation and guided differentiation to oligodendrocytes [19, 29]. JNJ-10397049 is a selective OX2 receptor antagonist (pIC50=7.4 and pKB values of 5.9 and 8.5 for OX1 and OX2 receptors, respectively). In the current study we used real-time polymerase chain reaction (PCR) to analyze the expression of Orexin 2 receptor; and used 3-(4,5-dimethylthiazol-2-yl)-2,5 diphenyl tetrazolium bromide (MTT) and neurosphere assay to check for proliferation of NPCs. For differentiation analysis after treating cells with different concentrations of JNJ-10307049, we used anti-nestin and Olig2 cell staining.
The reagents and materials used in this study were purchased from the following sources: JNJ 10397049 (Tocris Bioscience, Bristol, United Kingdom); 7-aminoactinomycin D (7AAD) stain (Miltenyi Biotech, Bergisch Gladbach, Germany), Neurocult mouse neural stem cell proliferation kit (Stem Cell Technologies, Vancouver, Canada), epidermal growth factor (Peprotech, Westlake Village, CA, USA), poly-l-ornithine (Sigma Aldrich, St. Louis, MO, USA), penicillin/streptomycin (pen/strep; Gibco, Waltham, MA, USA), Trypsin-EDTA 0.025% (Stem Cell Technologies), fetal bovine serum (Gibco), Dnase I (Sigma Aldrich), Dulbecco’s modified Eagle medium: nutrient mixture F-12 (Thermofisher Scientifics, Waltham, MA, USA), Ketamine, Xylazine, and MTT Reagent (Sigma Aldrich). All antibodies were from Abcam (Cambridge, United Kingdom): rabbit Olig2 antibody, mouse nestin antibody, rabbit fluorescein isothiocyanate (FITC)-conjugated secondary antibody, mouse FITC-conjugated secondary antibody.
All mice examined in this study were obtained from Shiraz University of Medical Sciences’ Animal center. All animal procedures were conducted in accordance with protocols approved by ethics committee of Shiraz University of Medical Sciences (SUMS) animal welfare (no. IR.SUMS.REC.1396.S448). Female and male Balb/c mice were mated at 1:2 ratios (male:female) and female mice were checked for the following next 5 days until vaginal plaque was observed. Mated animals were sacrificed on day 14 post-mating to harvest 14 days old fetal brain cortical NPCs.
On the day 14 of gestation, pregnant female mouse was deeply anesthetized with ketamine and xylazine and sacrificed. Heads of mice embryos that are 14.5 days old were dissected with fine scissors to be used for isolation of NSCs. The cortices were subtly dissected with fine forceps and scissors under dissecting microscope and placed in ice cold phosphate buffered saline (PBS) solution containing 10% penicillin-streptomycin (pen/strep). The cortices were mechanically dissociated into single cells and centrifuged at 200 g for 15 minutes [30]. Cells were plated at a density of 2×105 cells/ml in complete proliferation medium (Neurocult proliferation kit) supplemented with 20 ng/ml epidermal growth factor and 1% pen/strep [31] to grow as neurospheres. Every 5 or 7 days, neurospheres were passaged by trypsinization and then centrifuged at 110 g for 5 minutes. NPCs from passages 2 to 4 were used for the experiments.
The expression of NPCs marker nestin was evaluated by immunocytochemistry. The cells obtained from the second passage were cultured on poly-l-ornithine coated 96 well plates in Neurocult medium. After 2 days, the cells fixed by paraformaldehyde. Following permeabilization and blocking, the cells were incubated with mouse anti-nestin primary antibody overnight. Next, anti-mouse secondary FITC antibody were used, and the nuclei were stained with 7-aad.
In addition, relative expression of orexin 2 receptor (HCRTR2) in NPCs obtained from passages 2 and 4 was assessed by real-time PCR. Total RNA was extracted by RNXplus reagent (Cinnagen, Tehran, Iran) according to the manufacturer’s instructions. Total RNA (1 µM) was reverse transcribed into cDNA using Prime Script II First Strand cDNA synthesis Kit (TaKaRa, Kusatsu, Japan), and 0.1% of cDNA mixture was used as PCR template. Primers are specified in Table 1. The reaction was performed using a SYBR Green PCR Master Mix Kit (Yektataghiz azama, Tehran, Iran) in a Rotor-gene Q (Qiagen, Hilden, Germany) with an initial denaturation step at 95°C for 30 seconds, following 45 cycles. Each cycle transitioned between 3 steps of 95°C for 5 seconds, 60°C for 30 seconds and an extension step of 72°C for 30 seconds. Beta actin was employed as the housekeeping gene to account for sample variability. Relative gene expression is represented as fold change (2–∆Ct).
To evaluate the effect of orexin 2 receptor inhibitor on proliferation of the NPCs, MTT and neurosphere assays were performed.
The MTT assay is based on cells mitochondrial activity by assessing the conversion of MTT into formazan crystals by viable cells. The neurospheres were disrupted into single cells by trypsinization and then were seeded at a density of 5000 cells on poly-l-ornithine coated 96-well plates (Jetbiofil). On day 0 cells were treated with different concentrations of JNJ-10397049 (1, 5, 10, 15, 20, 25, and 30 µM). After 5 days, media was removed, and wells were washed gently twice with PBS. Two hundred µl of Dulbecco’s Modified Eagle Medium/F12 containing 1× MTT (0.5 mg/ml) was then added to each well and incubated for 4 hours. Medium was then removed from each plate and 200 µM of dimethyl sulfoxide (DMSO) was added to each well and the plate was shaken on an orbital shaker for 15 minutes. The absorption was read at 490 nm (FLOUstar Omega; BMG labtech, Ortenberg, Germany).
For neurosphere assay, single cells derived from neurospheres were seeded at a density of 5,000 cells on 96 well plates (SPL Life Sciences, Pocheon, Korea) for 7 days and treated with varying concentrations of Orexin 2 receptor inhibitor on day 0 of the culture; pictures were taken from each well at 5 random locations with 4× magnification on the last day of cell culture. The total number of neurospheres with a diameter of >50 µm were counted and presented as the percentage of neurosphere-forming frequency per well with respect to the total number of cells that were plated initially. The diameter of the neurospheres was also determined in each picture and the mean of diameter in all wells (n=3) was used to display cell self-renewal potency of the NPCs. Evaluation of each image was done using Infinity Analyze 4.6.0 software (Lumera, Bothell, WA, USA).
For assessing the differentiation of NPCs to oligodendroglial lineage following exposure to orexin 2 receptor inhibitor, immunocytochemistry and real-time PCR were done.
NPCs were plated on poly-l-ornithine coated plates at a density of 3×104 cells/well. Cells were cultured in differentiation medium containing Neurocult proliferation media supplemented with 1% fetal bovine serum without growth factor. Cells were treated with 20 µM of JNJ-10397049 for 7 days. For immunocytochemistry, the cells were stained with anti-Olig2 and anti-nestin antibodies and nuclei were counterstained with 7AAD. Immunofluorescent-labeled cells were visualized with Nikon Eclipse TS100 microscope (Melville, NY, USA) coupled with a Tucsen TrueChrome Metrics Camera (STEMMER IMAGING AG, Puchheim, Germany). Images were taken at 10× magnification from 3 random areas in each well (n=3 for each 5 condition). The percentage of nestin-positive and Olig2-positive cells were attained by dividing positive labeled cells for each antibody to total number of nuclei stained with 7AAD. Images were processed using Photoshop CC 2016 (Adobe World Headquarters, San Jose, CA, USA) and analyzed using ImageJ (U. S. National Institutes of Health, Bethesda, MD, USA) and Infinity Analyze 4.6.0 software (Lumera).
In addition, the expression of oligodendrocyte markers, platelet derived growth factor a (PDGFa) and 2’,3’-cyclic-nucleotide 3’-phosphodiesterase (CNPase) were analyzed using real-time PCR after exposure of the NPCs to 20 µM of JNJ-10397049 for 7 days. For this, the NPCs were plated at a density of 2×105 cells per 24 well plates coated with poly-l-ornithine in differentiation medium. RNA extraction, cDNA synthesis and real-time PCR were done as described in previous section. Primers are specified in Table 1.
All experiments were conducted at least in triplicate (n=3). Data were analyzed using SPSS software (version 16.0; SPSS Inc., Chicago, IL, USA). Data in figures are expressed as mean±standard error of the mean. One-way ANOVA followed by Bonferroni’s multiple comparison post hoc was performed to compare differences among multiple treatments. Independent samples t-test was performed to compare differences between two conditions. For all experiments, significance was defined as P-value <0.05.
The cells obtained from embryonic cortex expressed NPCs marker and orexin receptor 2.
From day 3 of culture, neurospheres were formed and showed an exponential growth until day 7. The neurospheres were on average >200 µm in diameter preceeding the passaging time. In addition, immunocytochemistry indicated that almost the majority of the cells were nestin-positive (Fig. 1A, B). As shown in Fig. 1C, the expression of orexin receptor 2 (HCRTR2) in the cells obtained from passage 4 was significantly increased compared to that of the cells obtained from passage 2 (t=–7.92, P<0.001).
NPCs were exposed to various concentrations of JNJ-10397049 (1, 5, 10, 15, 20, 25, and 30 µM). The results of MTT assay showed that the effect of JNJ-10397049 on NPCs proliferation was concentration dependent (Fig. 2). At 1 and 5 µM of JNJ-10397049, cell proliferation significantly increased (F=55.478, P<0.001). The 10, 15, and 20 µM of JNJ-10397049 did not have any significant effect on cell proliferation. However, the concentration of 25 and 30 µM of the drug were toxic (F=55.478, P<0.001). We also used the data obtained from MTT assay to fit it into a Log(half maximal effective concentration, EC50) graph with an R2 (goodness of fitness) of almost 1. Negative control contained 0.05% DMSO. The LogEC50 and EC50 of the drug are 0.9934 and 9.85, respectively.
Using neurosphere assay, frequency of neurospheres and the diameter of representative neurospheres from each condition were determined (Fig. 3). Compared to the control, the number of neurospheres considerably enhanced after exposure of the cells to 1 and 5 µM of JNJ-10397049 (F=13.471, P<0.001). Other concentrations of the drug did not change this index (Fig. 3B). In addition, the diameter of NPCs did not show any noticeable difference among all conditions (Fig. 3C).
Because 20 µM concentration of the drug did not have any effect on cell proliferation as well as it was no-toxic (based on the results of the previous section), this concentration of the drug was chosen for differentiation assay. As illustrated in Fig. 4, exposing the NPCs to JNJ-10397049 at 20 µM showed higher expression of Olig2 compared to control group (t=–6.09, P<0.01). On the other hand, lower expression of nestin was distinctly apparent in cells exposed to the drug (t=10.698, P<0.001). In addition, the presence of cells with a more branched morphology was clear in the group treated with the drug.
Additionally, we analyzed the expression of PDGFa and CNPase after exposure to 20 µM of JNJ-10397049 (Fig. 5). The expression of CNPase mRNA in the cells received the drug was noticeably higher than that of control group (t=–1.16, P<0.05). Although inhibition of orexin 2 receptor enhanced the expression of PDFRa mRNA compared to control group, the difference was not statistically significant.
In this study we presented the effect of JNJ-10397049 (a selective orexin 2 receptor inhibitor) in driving cortical NPCs toward an oligodendroglial lineage. The JNJ-10397049 treated NPCs showed a concentration dependent pattern regarding cell proliferation. The 20 µM of JNJ-10397049 did not have any significant effect on NPCs proliferation but increased differentiation of NPCs toward oligodendroglial lineage.
Generation of oligodendrocytes is important for repairing damaged tissue in neurodegenerative diseases [2]. Demyelination is one of the main events in MS and SCI [6, 8, 32-34]. In this sense, two strategies are considerable for production of oligodendrocytes and remyelination [9, 31]. One of them is stimulation of intrinsic regenerative capacity by using agents that can activate endogenous NPCs and control the proliferation, migration, and differentiation of them. Another approach is utilizing cells from exogenous sources [2, 3, 10, 35-37]. Among various cell sources, NPCs are one of the best candidates due to their capacity to produce specific CNS cells including neurons, astrocytes, and oligodendrocytes [1].
Orexin-containing neurons, originate in the hypothalamus and innervate the paraventricular nucleus of the thalamus (Fig. 6A). The orexin system was first discovered to regulate sleep (wakefulness) and appetite but recently studies revealed its role in pain regulation, cardiovascular function, neuroendocrine modulation, and other biological processes [21, 38]. Orexin receptor antagonists have shown great promise in treatment of insomnia, substance use disorder and psychosis [39-41]. Suvorexant, almorexant, and several other dual and single orexin receptors antagonists promote sleep in humans and are commercially available in the market [39-46]. In demyelinating diseases such as MS, healthy sleeping patterns is disrupted [47]; oligodendrocyte proliferation and/or differentiation and lipid biogenesis patterns are altered throughout sleep and wakefulness [48]. As depicted in Fig. 6A, orexin has an important role in wakefulness by regulating and interacting with serotonin, acetylcholine, histamine and the noradrenergic during this phase [49].
Bellesi et al. [50] demonstrated negative implications of chronic sleep loss on myelination. Orexin potential role on vigilance state has been demonstrated by discovery of orexin 2 receptor mutations in narcoleptic dogs. As previously mentioned, neurotransmitters of vigilance states have recently been associated with oligodendrocyte differentiation and remyelination. Chen et al. [13] presented the negative regulation of oligodendrocyte (OL) differentiation by histamine receptor 3; serotonin adversely influences OL development and myelination [14]; in vitro experiments have demonstrated the potential of muscarinic antagonist treatment on OPC cultures in inducing differentiation towards mature OLs [12]. No studies have explored the role of orexin receptor antagonists on OL formation. However, hypocretin pathway have been investigated in MS mouse models. Becquet et al. [51] showed diminished neuroinflammation in experimental autoimmune encephalomyelitis (EAE) mouse models by systematic administration of orexin A. In another study, orexinergic system was associated with pathologic development of EAE and administration of orexin A attenuated clinical symptoms in EAE mice [52]. In another study by Gencer et al. [53], serum orexin A levels was positively linked with disease progress and impairment of motor function in MS. Here, for the first time, we showed the effects of an orexin receptor antagonist on behavior of NPCs. Using this antagonist helped both in increasing proliferation of NPCs (lower concentrations) and production of oligodendrocytes from them (20 µm of JNJ-10397049).
We studied the differentiation of NPCs to oligodendrocytes by immunostaining cells with nestin and Olig2 antibodies and examining the mRNA expression of CNPase and PDGFa (Fig. 6B) [54, 55]. Oligodendrocyte development depends on stage-specific transcription factors, including Olig2, Sox10, YY1, Olig1, MRF, and ZFP191 [56]. Olig1/2 are two closely related basic helix-loop-helix transcription factors, which are crucial and sufficient in the specification of oligodendroglial lineage and in differentiation, maturation and myelination/remyelination by oligodendrocytes [56]. Olig-2 null mice fail to develop cell of oligodendroglial lineage, moreover, overexpression of Oligo2 in NSCs is sufficient to specify OPCs rather than neurons and/or astrocytes, resulting in a dramatic increase in the number of OPCs [57, 58]. In the present study, blockage of orexin receptor led to enhancement of Olig2 expression in NPCs and consequently increase in oligodendrocyte-like cells. Nestin stains undifferentiated NSCs and is predominantly expressed in control conditions.
Myelin is a unique membrane structure enveloping axonal segments in the nervous system. A mature myelin sheath is comprised of two main morphological segments, a compact and a non-compact myelin. CNPase is a membrane anchored enzyme and the most abundant CNS non-compact myelin protein [59]. This enzyme is concentrated beneath oligodendrocytes cell membranes and in regions of the myelin internodes containing oligodendrocyte cytoplasm. CNPase is a potential autoantigen in MS, which does not have a clear biological role. CNPase-deficient mice develop axonal swelling and degeneration leading to progression of motor deficits and premature death [60, 61]. The function, structure, and importance of CNPase have recently been reviewed by Raasakka et al. [62]. CNPase is widely used as a biomarker of mature oligodendrocytes. Based on our results, inhibition of orexin receptor resulted in increase of CNPase-expressing cells at transcriptional level. Whereas PDGRα which is highly expressed in OPCs shows only a slight increase in transcriptional expression after exposure to JNJ-10397049.
Based on our results, orexin2 receptor is transcriptionally expressed in NPCs. However, the expression of this receptor at protein level and its associated agents in the hypocretin pathway in NPCs needs to be further elucidated. In addition, exposure of NPCs to JNJ-10307049 can be helpful in in vitro cell manipulations for production of more oligodendrocyte-like cells. Further in vivo investigations are needed to clarify remyelination capacity of JNJ-10397049 used in the present study as an inhibitor of orexin 2 receptor. Additionally, further exploring and elucidating the underlying molecular mechanism of orexin receptors and hypocretin pathway in remyelination and oligodendrogenesis or maturation of oligodendrocytes in vivo and in vitro is necessary. This can open a new avenue for remyelinating therapies in demyelinating diseases.
Acknowledgements
This work was financially supported by a grant number 14482 from Shiraz University of Medical Sciences, Shiraz, Iran. We are grateful to Zahra Zeraatpisheh and Marjan Ghorbanpour for their technical help and support. We had collaboration with Department of Neuroscience, School of Advanced Medical Sciences and Technology for performing this research.
Notes
Author Contributions
Conceptualization: NK, TK. Data acquisition: NK, H Aligholi. Data analysis or interpretation: NK, H Aligholi. Drafting of the manuscript: NK, H Azari, TK, H Aligholi. Critical revision of the manuscript: NK, H Azari, H Aligholi. Approval of the final version of the manuscript: all authors.
References
1. Gage FH. 2000; Mammalian neural stem cells. Science. 287:1433–8. DOI: 10.1126/science.287.5457.1433. PMID: 10688783.


2. Ming GL, Song H. 2011; Adult neurogenesis in the mammalian brain: significant answers and significant questions. Neuron. 70:687–702. DOI: 10.1016/j.neuron.2011.05.001. PMID: 21609825. PMCID: PMC3106107.


3. Takano M, Kawabata S, Shibata S, Yasuda A, Nori S, Tsuji O, Nagoshi N, Iwanami A, Ebise H, Horiuchi K, Okano H, Nakamura M. 2017; Enhanced functional recovery from spinal cord injury in aged mice after stem cell transplantation through HGF induction. Stem Cell Reports. 8:509–18. DOI: 10.1016/j.stemcr.2017.01.013. PMID: 28216143. PMCID: PMC5355635.


4. Desai RA, Davies AL, Tachrount M, Kasti M, Laulund F, Golay X, Smith KJ. 2016; Cause and prevention of demyelination in a model multiple sclerosis lesion. Ann Neurol. 79:591–604. DOI: 10.1002/ana.24607. PMID: 26814844. PMCID: PMC4949637.


5. Haider L, Zrzavy T, Hametner S, Höftberger R, Bagnato F, Grabner G, Trattnig S, Pfeifenbring S, Brück W, Lassmann H. 2016; The topograpy of demyelination and neurodegeneration in the multiple sclerosis brain. Brain. 139(Pt 3):807–15. DOI: 10.1093/brain/awv398. PMID: 26912645. PMCID: PMC4766379.


6. Cohen-Adad J, El Mendili MM, Lehéricy S, Pradat PF, Blancho S, Rossignol S, Benali H. 2011; Demyelination and degeneration in the injured human spinal cord detected with diffusion and magnetization transfer MRI. Neuroimage. 55:1024–33. DOI: 10.1016/j.neuroimage.2010.11.089. PMID: 21232610.


7. Bouhrara M, Reiter DA, Bergeron CM, Zukley LM, Ferrucci L, Resnick SM, Spencer RG. 2018; Evidence of demyelination in mild cognitive impairment and dementia using a direct and specific magnetic resonance imaging measure of myelin content. Alzheimers Dement. 14:998–1004. DOI: 10.1016/j.jalz.2018.03.007. PMID: 29679574. PMCID: PMC6097903.


8. Mitew S, Kirkcaldie MT, Halliday GM, Shepherd CE, Vickers JC, Dickson TC. 2010; Focal demyelination in Alzheimer's disease and transgenic mouse models. Acta Neuropathol. 119:567–77. DOI: 10.1007/s00401-010-0657-2. PMID: 20198482.


9. Franklin RJ, Ffrench-Constant C. 2008; Remyelination in the CNS: from biology to therapy. Nat Rev Neurosci. 9:839–55. DOI: 10.1038/nrn2480. PMID: 18931697.


10. Yue T, Xian K, Hurlock E, Xin M, Kernie SG, Parada LF, Lu QR. 2006; A critical role for dorsal progenitors in cortical myelination. J Neurosci. 26:1275–80. DOI: 10.1523/JNEUROSCI.4717-05.2006. PMID: 16436615. PMCID: PMC6674567.


11. Lubetzki C, Zalc B, Williams A, Stadelmann C, Stankoff B. 2020; Remyelination in multiple sclerosis: from basic science to clinical translation. Lancet Neurol. 19:678–88. DOI: 10.1016/S1474-4422(20)30140-X. PMID: 32702337.


12. Melchor GS, Khan T, Reger JF, Huang JK. 2019; Remyelination pharmacotherapy investigations highlight diverse mechanisms underlying multiple sclerosis progression. ACS Pharmacol Transl Sci. 2:372–86. DOI: 10.1021/acsptsci.9b00068. PMID: 32259071. PMCID: PMC7088971.


13. Chen Y, Zhen W, Guo T, Zhao Y, Liu A, Rubio JP, Krull D, Richardson JC, Lu H, Wang R. 2017; Histamine receptor 3 negatively regulates oligodendrocyte differentiation and remyelination. PLoS One. 12:e0189380. DOI: 10.1371/journal.pone.0189380. PMID: 29253893. PMCID: PMC5734789.


14. Fan LW, Bhatt A, Tien LT, Zheng B, Simpson KL, Lin RC, Cai Z, Kumar P, Pang Y. 2015; Exposure to serotonin adversely affects oligodendrocyte development and myelination in vitro. J Neurochem. 133:532–43. DOI: 10.1111/jnc.12988. PMID: 25382136. PMCID: PMC4400220.
15. Ghareghani M, Sadeghi H, Zibara K, Danaei N, Azari H, Ghanbari A. 2017; Melatonin increases oligodendrocyte differentiation in cultured neural stem cells. Cell Mol Neurobiol. 37:1319–24. DOI: 10.1007/s10571-016-0450-4. PMID: 27987059.


16. Olivier P, Fontaine RH, Loron G, Van Steenwinckel J, Biran V, Massonneau V, Kaindl A, Dalous J, Charriaut-Marlangue C, Aigrot MS, Pansiot J, Verney C, Gressens P, Baud O. 2009; Melatonin promotes oligodendroglial maturation of injured white matter in neonatal rats. PLoS One. 4:e7128. DOI: 10.1371/journal.pone.0007128. PMID: 19771167. PMCID: PMC2742165.


17. Sotthibundhu A, Phansuwan-Pujito P, Govitrapong P. 2010; Melatonin increases proliferation of cultured neural stem cells obtained from adult mouse subventricular zone. J Pineal Res. 49:291–300. DOI: 10.1111/j.1600-079X.2010.00794.x. PMID: 20663047.


18. Sakurai T, Mieda M. 2011; Connectomics of orexin-producing neurons: interface of systems of emotion, energy homeostasis and arousal. Trends Pharmacol Sci. 32:451–62. DOI: 10.1016/j.tips.2011.03.007. PMID: 21565412.


19. Yin J, Babaoglu K, Brautigam CA, Clark L, Shao Z, Scheuermann TH, Harrell CM, Gotter AL, Roecker AJ, Winrow CJ, Renger JJ, Coleman PJ, Rosenbaum DM. 2016; Structure and ligand-binding mechanism of the human OX1 and OX2 orexin receptors. Nat Struct Mol Biol. 23:293–9. DOI: 10.1038/nsmb.3183. PMID: 26950369.


20. Ng MC. 2017; Orexin and epilepsy: potential role of REM sleep. Sleep. 40:zsw061. DOI: 10.1093/sleep/zsw061. PMID: 28364414.


21. Soya S, Takahashi TM, McHugh TJ, Maejima T, Herlitze S, Abe M, Sakimura K, Sakurai T. 2017; Orexin modulates behavioral fear expression through the locus coeruleus. Nat Commun. 8:1606. DOI: 10.1038/s41467-017-01782-z. PMID: 29151577. PMCID: PMC5694764. PMID: b6b7b0ef9da64f028089ee1720edac0e.


22. Liblau RS, Vassalli A, Seifinejad A, Tafti M. 2015; Hypocretin (orexin) biology and the pathophysiology of narcolepsy with cataplexy. Lancet Neurol. 14:318–28. DOI: 10.1016/S1474-4422(14)70218-2. PMID: 25728441.


23. Liguori C. 2017; Orexin and Alzheimer's disease. Curr Top Behav Neurosci. 33:305–22. DOI: 10.1007/7854_2016_50. PMID: 28012089.


24. Liu RJ, van den Pol AN, Aghajanian GK. 2002; Hypocretins (orexins) regulate serotonin neurons in the dorsal raphe nucleus by excitatory direct and inhibitory indirect actions. J Neurosci. 22:9453–64. DOI: 10.1523/JNEUROSCI.22-21-09453.2002. PMID: 12417670. PMCID: PMC6758063.


25. Lyons DJ, Hellysaz A, Ammari R, Broberger C. 2017; Hypocretin/orexin peptides excite rat neuroendocrine dopamine neurons through orexin 2 receptor-mediated activation of a mixed cation current. Sci Rep. 7:41535. DOI: 10.1038/srep41535. PMID: 28145492. PMCID: PMC5286397.


26. Tao R, Ma Z, McKenna JT, Thakkar MM, Winston S, Strecker RE, McCarley RW. 2006; Differential effect of orexins (hypocretins) on serotonin release in the dorsal and median raphe nuclei of freely behaving rats. Neuroscience. 141:1101–5. DOI: 10.1016/j.neuroscience.2006.05.027. PMID: 16820265.


27. Yamada N, Katsuura G, Tatsuno I, Asaki T, Kawahara S, Ebihara K, Saito Y, Nakao K. 2008; Orexin decreases mRNA expressions of NMDA and AMPA receptor subunits in rat primary neuron cultures. Peptides. 29:1582–7. DOI: 10.1016/j.peptides.2008.05.002. PMID: 18573570.


28. Yamanaka A, Tsujino N, Funahashi H, Honda K, Guan JL, Wang QP, Tominaga M, Goto K, Shioda S, Sakurai T. 2002; Orexins activate histaminergic neurons via the orexin 2 receptor. Biochem Biophys Res Commun. 290:1237–45. DOI: 10.1006/bbrc.2001.6318. PMID: 11811995.


29. Yin J, Mobarec JC, Kolb P, Rosenbaum DM. 2015; Crystal structure of the human OX2 orexin receptor bound to the insomnia drug suvorexant. Nature. 519:247–50. DOI: 10.1038/nature14035. PMID: 25533960.


30. Azari H, Sharififar S, Rahman M, Ansari S, Reynolds BA. 2011; Establishing embryonic mouse neural stem cell culture using the neurosphere assay. J Vis Exp. (47):2457. DOI: 10.3791/2457. PMID: 21248704. PMCID: PMC3182648.


31. Lassmann H, van Horssen J. 2011; The molecular basis of neurodegeneration in multiple sclerosis. FEBS Lett. 585:3715–23. DOI: 10.1016/j.febslet.2011.08.004. PMID: 21854776.


32. Geurts JJ, Bö L, Roosendaal SD, Hazes T, Daniëls R, Barkhof F, Witter MP, Huitinga I, van der Valk P. 2007; Extensive hippocampal demyelination in multiple sclerosis. J Neuropathol Exp Neurol. 66:819–27. DOI: 10.1097/nen.0b013e3181461f54. PMID: 17805012.


33. Kim LJ, Martinez D, Fiori CZ, Baronio D, Kretzmann NA, Barros HM. 2015; Hypomyelination, memory impairment, and blood-brain barrier permeability in a model of sleep apnea. Brain Res. 1597:28–36. DOI: 10.1016/j.brainres.2014.11.052. PMID: 25482664.


34. Pillai JA, Leverenz JB. 2017; Sleep and neurodegeneration: a critical appraisal. Chest. 151:1375–86. DOI: 10.1016/j.chest.2017.01.002. PMID: 28087304.
35. Mothe AJ, Tam RY, Zahir T, Tator CH, Shoichet MS. 2013; Repair of the injured spinal cord by transplantation of neural stem cells in a hyaluronan-based hydrogel. Biomaterials. 34:3775–83. DOI: 10.1016/j.biomaterials.2013.02.002. PMID: 23465486.


36. Wolswijk G. 1998; Chronic stage multiple sclerosis lesions contain a relatively quiescent population of oligodendrocyte precursor cells. J Neurosci. 18:601–9. DOI: 10.1523/JNEUROSCI.18-02-00601.1998. PMID: 9425002. PMCID: PMC6792542.


37. Yousefifard M, Rahimi-Movaghar V, Nasirinezhad F, Baikpour M, Safari S, Saadat S, Moghadas Jafari A, Asady H, Razavi Tousi SM, Hosseini M. 2016; Neural stem/progenitor cell transplantation for spinal cord injury treatment; a systematic review and meta-analysis. Neuroscience. 322:377–97. DOI: 10.1016/j.neuroscience.2016.02.034. PMID: 26917272.


38. Sakurai T, Amemiya A, Ishii M, Matsuzaki I, Chemelli RM, Tanaka H, Williams SC, Richardson JA, Kozlowski GP, Wilson S, Arch JR, Buckingham RE, Haynes AC, Carr SA, Annan RS, McNulty DE, Liu WS, Terrett JA, Elshourbagy NA, Bergsma DJ, Yanagisawa M. 1998; Orexins and orexin receptors: a family of hypothalamic neuropeptides and G protein-coupled receptors that regulate feeding behavior. Cell. 92:573–85. DOI: 10.1016/S0092-8674(00)80949-6. PMID: 9527442.


39. Recourt K, de Boer P, Zuiker R, Luthringer R, Kent J, van der Ark P, Van Hove I, van Gerven J, Jacobs G, van Nueten L, Drevets W. 2019; The selective orexin-2 antagonist seltorexant (JNJ-42847922/MIN-202) shows antidepressant and sleep-promoting effects in patients with major depressive disorder. Transl Psychiatry. 9:216. Erratum in: Transl Psychiatry 2019;9:240. DOI: 10.1038/s41398-019-0585-4. PMID: 31578318. PMCID: PMC6775147.


40. Zammit G, Dauvilliers Y, Pain S, Sebök Kinter D, Mansour Y, Kunz D. 2020; Daridorexant, a new dual orexin receptor antagonist, in elderly subjects with insomnia disorder. Neurology. 94:e2222–32. DOI: 10.1212/WNL.0000000000009475. PMID: 32341187.


41. Elam HB, Perez SM, Donegan JJ, Lodge DJ. 2021; Orexin receptor antagonists reverse aberrant dopamine neuron activity and related behaviors in a rodent model of stress-induced psychosis. Transl Psychiatry. 11:114. DOI: 10.1038/s41398-021-01235-8. PMID: 33558469. PMCID: PMC7870676. PMID: 98cbb80306624c4e97396c531721d1e7.


42. Brooks S, Jacobs GE, de Boer P, Kent JM, Van Nueten L, van Amerongen G, Zuiker R, Kezic I, Luthringer R, van der Ark P, van Gerven JM, Drevets W. 2019; The selective orexin-2 receptor antagonist seltorexant improves sleep: an exploratory double-blind, placebo controlled, crossover study in antidepressant-treated major depressive disorder patients with persistent insomnia. J Psychopharmacol. 33:202–9. DOI: 10.1177/0269881118822258. PMID: 30644312.


43. Dubey AK, Handu SS, Mediratta PK. 2015; Suvorexant: the first orexin receptor antagonist to treat insomnia. J Pharmacol Pharmacother. 6:118–21. DOI: 10.4103/0976-500X.155496. PMID: 25969666. PMCID: PMC4419247.


44. Kishi T, Matsunaga S, Iwata N. 2015; Suvorexant for primary insomnia: a systematic review and meta-analysis of randomized placebo-controlled trials. PLoS One. 10:e0136910. DOI: 10.1371/journal.pone.0136910. PMID: 26317363. PMCID: PMC4552781.


45. Scammell TE, Winrow CJ. 2011; Orexin receptors: pharmacology and therapeutic opportunities. Annu Rev Pharmacol Toxicol. 51:243–66. DOI: 10.1146/annurev-pharmtox-010510-100528. PMID: 21034217. PMCID: PMC3058259.


46. Roth T, Black J, Cluydts R, Charef P, Cavallaro M, Kramer F, Zammit G, Walsh J. 2017; Dual orexin receptor antagonist, almorexant, in elderly patients with primary insomnia: a randomized, controlled study. Sleep. 40:zsw034. DOI: 10.1093/sleep/zsw034. PMCID: PMC5806584. PMID: 28364509.


47. Braley TJ, Kratz AL, Kaplish N, Chervin RD. 2016; Sleep and cognitive function in multiple sclerosis. Sleep. 39:1525–33. DOI: 10.5665/sleep.6012. PMID: 27166237. PMCID: PMC4945311.


48. Bellesi M, Pfister-Genskow M, Maret S, Keles S, Tononi G, Cirelli C. 2013; Effects of sleep and wake on oligodendrocytes and their precursors. J Neurosci. 33:14288–300. DOI: 10.1523/JNEUROSCI.5102-12.2013. PMID: 24005282. PMCID: PMC3874087.


49. Andretic R, Franken P, Tafti M. 2008; Genetics of sleep. Annu Rev Genet. 42:361–88. DOI: 10.1146/annurev.genet.42.110807.091541. PMID: 18983259.


50. Bellesi M, Haswell JD, de Vivo L, Marshall W, Roseboom PH, Tononi G, Cirelli C. 2018; Myelin modifications after chronic sleep loss in adolescent mice. Sleep. 41:zsy034. DOI: 10.1093/sleep/zsy034. PMID: 29741724. PMCID: PMC5946929.


51. Becquet L, Abad C, Leclercq M, Miel C, Jean L, Riou G, Couvineau A, Boyer O, Tan YV. 2019; Systemic administration of orexin A ameliorates established experimental autoimmune encephalomyelitis by diminishing neuroinflammation. J Neuroinflammation. 16:64. DOI: 10.1186/s12974-019-1447-y. PMID: 30894198. PMCID: PMC6425555. PMID: 69e7f6833b604226b92795a7fa86ab95.


52. Fatemi I, Shamsizadeh A, Ayoobi F, Taghipour Z, Sanati MH, Roohbakhsh A, Motevalian M. 2016; Role of orexin-A in experimental autoimmune encephalomyelitis. J Neuroimmunol. 291:101–9. DOI: 10.1016/j.jneuroim.2016.01.001. PMID: 26857503.


53. Gencer M, Akbayır E, Şen M, Arsoy E, Yılmaz V, Bulut N, Tüzün E, Türkoğlu R. 2019; Serum orexin-A levels are associated with disease progression and motor impairment in multiple sclerosis. Neurol Sci. 40:1067–70. DOI: 10.1007/s10072-019-3708-z. PMID: 30645749.


54. Long KLP, Breton JM, Barraza MK, Perloff OS, Kaufer D. 2021; Hormonal regulation of oligodendrogenesis I: effects across the lifespan. Biomolecules. 11:283. DOI: 10.3390/biom11020283. PMID: 33672939. PMCID: PMC7918364. PMID: 394ed4eb50074feab425dcbd3ffb6324.


55. Armada-Moreira A, Ribeiro FF, Sebastião AM, Xapelli S. 2015; Neuroinflammatory modulators of oligodendrogenesis. Neuroimmunol Neuroinflammation. 2:263–73. DOI: 10.4103/2347-8659.167311.


56. Li H, He Y, Richardson WD, Casaccia P. 2009; Two-tier transcriptional control of oligodendrocyte differentiation. Curr Opin Neurobiol. 19:479–85. DOI: 10.1016/j.conb.2009.08.004. PMID: 19740649. PMCID: PMC2826212.


57. Copray S, Balasubramaniyan V, Levenga J, de Bruijn J, Liem R, Boddeke E. 2006; Olig2 overexpression induces the in vitro differentiation of neural stem cells into mature oligodendrocytes. Stem Cells. 24:1001–10. DOI: 10.1634/stemcells.2005-0239. PMID: 16253982.
58. Mei F, Wang H, Liu S, Niu J, Wang L, He Y, Etxeberria A, Chan JR, Xiao L. 2013; Stage-specific deletion of Olig2 conveys opposing functions on differentiation and maturation of oligodendrocytes. J Neurosci. 33:8454–62. DOI: 10.1523/JNEUROSCI.2453-12.2013. PMID: 23658182. PMCID: PMC3865513.


59. Raasakka A, Myllykoski M, Laulumaa S, Lehtimäki M, Härtlein M, Moulin M, Kursula I, Kursula P. 2015; Determinants of ligand binding and catalytic activity in the myelin enzyme 2',3'-cyclic nucleotide 3'-phosphodiesterase. Sci Rep. 5:16520. DOI: 10.1038/srep16520. PMID: 26563764. PMCID: PMC4643303.


60. Rösener M, Muraro PA, Riethmüller A, Kalbus M, Sappler G, Thompson RJ, Lichtenfels R, Sommer N, McFarland HF, Martin R. 1997; 2',3'-cyclic nucleotide 3'-phosphodiesterase: a novel candidate autoantigen in demyelinating diseases. J Neuroimmunol. 75:28–34. DOI: 10.1016/S0165-5728(96)00230-5. PMID: 9143234.


61. Scherer SS, Braun PE, Grinspan J, Collarini E, Wang DY, Kamholz J. 1994; Differential regulation of the 2',3'-cyclic nucleotide 3'-phosphodiesterase gene during oligodendrocyte development. Neuron. 12:1363–75. DOI: 10.1016/0896-6273(94)90451-0. PMID: 8011341.


62. Raasakka A, Kursula P. 2014; The myelin membrane-associated enzyme 2',3'-cyclic nucleotide 3'-phosphodiesterase: on a highway to structure and function. Neurosci Bull. 30:956–66. DOI: 10.1007/s12264-013-1437-5. PMID: 24807122. PMCID: PMC5562554.


Fig. 1
Characterization of neurospheres established embryonic 14.5 days old mice brain. Phase contrast images of suspended neurospheres on day 5 and day 7 (A) of primary neurosphere culture (passage 0) at 4× magnification. (B) Nestin-labeled neural precursor cells (NPCs; green) on day 7 of culture (passage 0) with 7-aminoactinomycin D (7AAD)-counterstained nuclei (red) at 4× magnification. (C) HCRTR2 expression in the NPCs after passages 2 and 4. *P<0.001. Scale bar=100 μm.

Fig. 2
The results of 3-(4,5-dimethylthiazol-2-yl)-2,5 diphenyl tetrazolium bromide (MTT) assay after exposure of the neural precursor cells (NPCs) to different concentration of Orexin 2 receptor inhibitor. (A) Graph representing obtained absorbance at 490 nm from an MTT assay of NPCs treated with varying concentrations (μM) of JNJ-10397049. Final concentration of dimethyl sulfoxide was 0.05% and was included in control wells. (B) Log(half maximal effective concentration, EC50) of JNJ-10397049 from log-normalized data was calculated using Graph Pad Prism 7. Data are presented as mean±standard error of the mean. *P<0.001 compared to control, n=3.
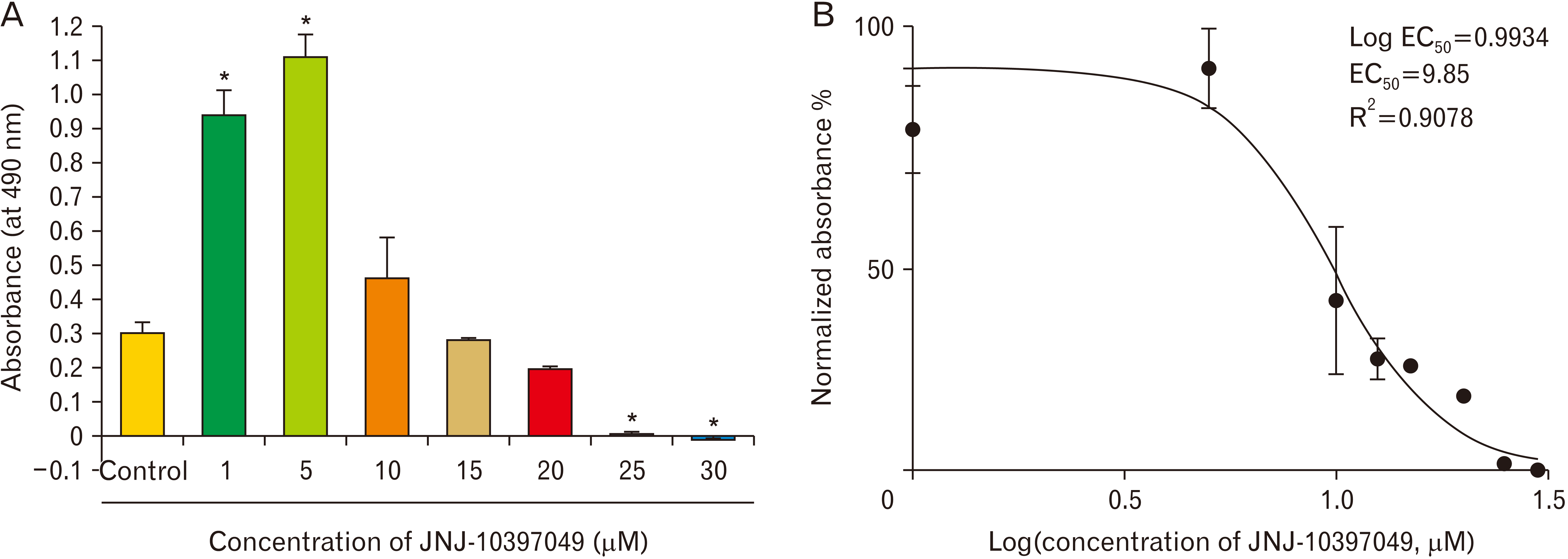
Fig. 3
Neurosphere assay after exposure of the neural precursor cells (NPCs) to different concentration of orexin 2 receptor inhibitor. (A) Phase contrast images of NPCs on day 7 of cell culture treated with varying concentrations of JNJ-10397049 at 4× magnification. The quantitative analysis of the number (B) and the diameter of neurospheres (C). Data are represented as mean±standard error of the mean. *P<0.001, n=3. Scale bar=100 μm.

Fig. 4
Changing the fate of neural precursor cells (NPCs) after exposure to orexin 2 receptor inhibitor. The expression of oligodendroglial marker Olig2 (green; A) and NPCs marker nestin (green; B) were evaluated in NPCs after treatment with 0 or 20 µm of JNJ-10397049. The nuclei were stained with 7-aminoactinomycin D (7AAD) and are shown in red. (C) Representative phase contrast images taken from the differentiation culture on day 7 at 10× magnification. The black arrowheads specify morphologically suggested mature oligodendrocytes. The white arrowhead in image bottom panel of C specifies cells likely to be of neuronal lineage. (D, E) Quantification of the percentage of Olig2 and nestin-positive cells per area on day 7 of culture. *P<0.001. Scale bar=100 μm, n=3.

Fig. 5
Expression of oligodendrocyte-related genes in neural precursor cells (NPCs) after exposure to orexin 2 receptor inhibitor. The relative expression of platelet derived growth factor a (PDGFa) and 2’,3’-cyclic-nucleotide 3’-phosphodiesterase (CNPase) in NPCs were evaluated after treatment of the cells with 0 or 20 µm of JNJ-10397049. *P<0.05, n=4.
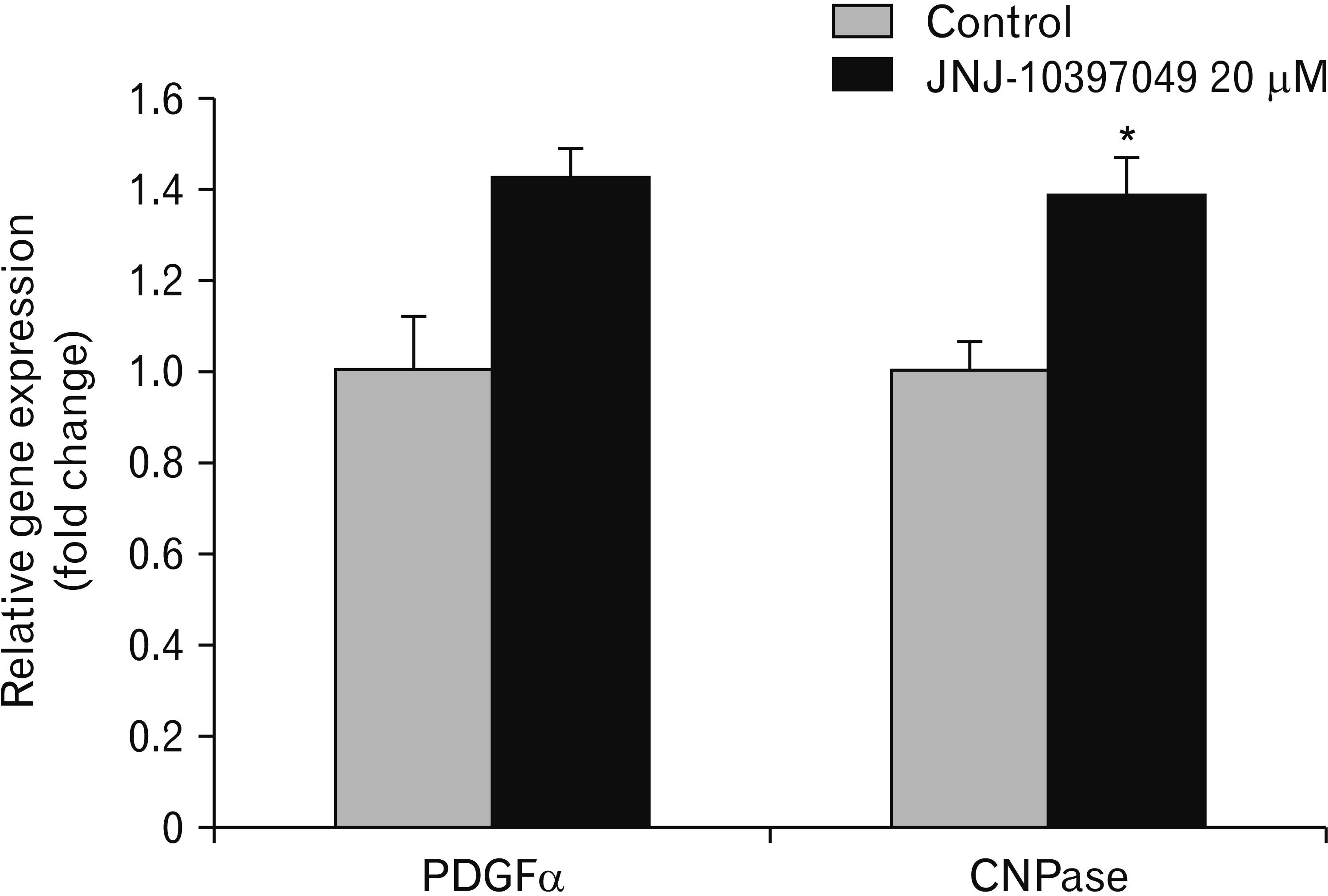
Fig. 6
Orexin interplay with other neurotransmitters involved in vigilance state and expressed biomarkers of oligodendroglial lineage throughout differentiation from neural precursor cells (NPCs). (A) Orexin (Hcrt) is one of the main players of vigilance state and acts via regulating several neurotransmitters involved in this state. Vigilance state is mainted by cholinergic (Ach) inputs from brain stem (LDT, laterodorsal tegmentum; PRP, pontine reticular formation; PPT, pendonuclopontine tegmentum) to thalamus and subsequently activates the cortex. Cholinergic inputs from the basal forebrain (BF) are also involved. Other factors involved in maintaining cortical activation in wakefulness are serotonergic (5HT) dorsal raphe nucleus (DRN), noradrenergic (NA) (LC, corpus coeruleus), dopaminergic (DA) (vPAG, ventral periaqueductal), histaminergic (His) (TMN, tuberomammillary nucleus), and hypocretinergic (Hcrt) inputs [49]. (B) Expression of prominent biomarkers of oligodendrogenesis at different stages of differentiation of NPCs to myelinating oligodendrocytes. Nestin expression is pronounced in NPCs and oligodendrocyte precursor cells (OPCs) and is not detectable in immature oligodendrocytes. Platelet derived growth factor alpha (PDGFa) is present in OPCs and immature oligodendrocytes. Olig2 and Sox10 are expressed throughout almost all stages of oligodendrocyte differentiation (OPC, immature OL, mature and myelinating OL). 2’,3’-cyclic-nucleotide 3’-phosphodiesterase (CNPase) expression is noticeable in immature OLs and gradually increases in mature and myelinating OLs [54, 55].
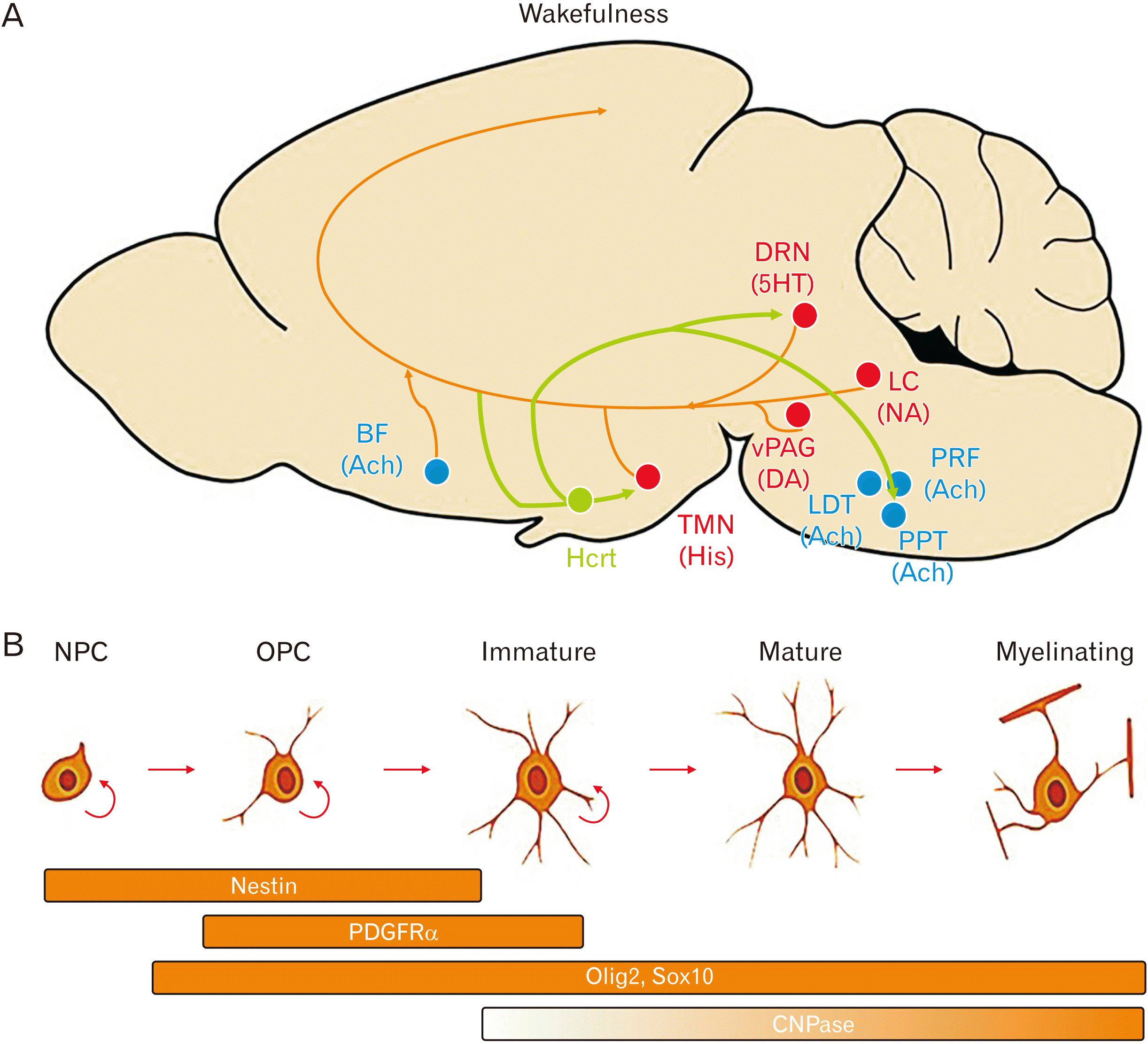
Table 1
List of primers used in the study