Abstract
Oxytocin is a neuropeptide produced primarily in the hypothalamus and plays an important role in the regulation of mammalian birth and lactation. It has been shown that oxytocin has important cardiovascular protective effects. Here we investigated the effects of oxytocin on vascular reactivity and underlying the mechanisms in human umbilical vein endothelial cells (HUVECs) in vitro and in rat aorta ex vivo. Oxytocin increased phospho-eNOS (Ser 1177) and phospho-Akt (Ser 473) expression in HUVECs in vitro and the aorta of rat ex vivo. Wortmannin, a specific inhibitor of phosphatidylinositol 3-kinase (PI3K), inhibited oxytocin-induced Akt and eNOS phosphorylation. In the rat aortic rings, oxytocin induced a biphasic vascular reactivity: oxytocin at low dose (10-9–10-8 M) initiated a vasorelaxation followed by a vasoconstriction at high dose (10-7 M). L-NAME (a nitric oxide synthase inhibitor), endothelium removal or wortmannin abolished oxytocin-induced vasorelaxation, and slightly enhanced oxytocin-induced vasoconstriction. Atosiban, an oxytocin/vasopressin 1a receptor inhibitor, totally blocked oxytocin-induced relaxation and vasoconstriction. PD98059 (ERK1/2 inhibitor) partially inhibited oxytocin-induced vasoconstriction. Oxytocin also increased aortic phospho-ERK1/2 expression, which was reduced by either atosiban or PD98059, suggesting that oxytocin-induced vasoconstriction was partially mediated by oxytocin/V1aR activation of ERK1/2. The present study demonstrates that oxytocin can activate different signaling pathways to cause vasorelaxation or vasoconstriction. Oxytocin stimulation of PI3K/eNOS-derived nitric oxide may participate in maintenance of cardiovascular homeostasis, and different vascular reactivities to low or high dose of oxytocin suggest that oxytocin may have different regulatory effects on vascular tone under physiological or pathophysiological conditions.
Oxytocin is a hypothalamus neuropeptide, which has been shown to play an important role in the regulation of mammalian birth and lactation and a variety of social behaviors [1]. Oxytocin is considered as a typical stress hormone that responds to various acute and chronic stress stimuli, and plays a significant role in a wide array of physiological activities [1]. The cardiovascular system is one of oxytocin’s important targets [2,3]. Oxytocin and its receptors are expressed in the heart and its vascular cells [4]. In the cultured brain microvascular endothelial cells (BMEC), oxytocin inhibits ox-LDL-induced monocyte adhesion to BMEC and the expression of adhesion molecules [5]. Chronic administration of oxytocin reduces atherosclerotic plaque and inflammation in the aorta of ApoE-/- mouse [6]. It has been proposed that the intrinsic oxytocin system may play a critical role in the maintenance of cardiovascular homeostasis [2,4,7].
The cardiovascular effects of oxytocin include natriuresis, negative inotropy, chronotropy, and the regulation of vascular tone or blood pressure [2]. It has been reported that the systemic administration of oxytocin in rats significantly affects blood pressure, vascular tone and cardiovascular regulation [8,9]. Short term intravenous administration of oxytocin to women can enhance uterine contraction or decrease blood loss during labor or caesarean delivery [10]. Oxytocin’s hypotensive response may be mediated by decreasing total vascular resistance [11].
Early studies suggest that oxytocin induces vasodilatory effects in the canine basilar artery which may be mediated through the endothelium and vasopressin 1 receptors [12,13]. Furthermore, Oyama et al. [12] showed that the intracisternal injection of L-NAME, an inhibitor of nitric oxide synthesis, suppressed oxytocin-mediated vasodilatory effects on the basilar arteries in dogs, suggesting the role of nitric oxide (NO) in vasodilatory effect of oxytocin. Although growing evidence has shown that oxytocin plays a role in the regulation of cardiovascular system, and perhaps in the regulation of vascular tone [2,14], the physiological function of oxytocin in terms of the regulation of vascular reactivity and vascular tone remains to be established. To address this question, we investigated the effects of oxytocin on vascular reactivity and elucidated the underlying mechanisms in vitro and ex vivo.
Six-week-old male Sprague–Dawley male rats were purchased from Liaoning Laboratory Animal Center and maintained under controlled conditions of light, temperature, and humidity. Animals were housed in animal facilities accredited by The Chinese Association for Accreditation of Laboratory Animal Care. All studies were approved by the Institutional Animal Care and Use Committee of Shenyang Medical University. After 2 weeks of accommodation to the new environment, the rats were sacrificed by overdose of sodium pentobarbital (100 mg/kg. i.p.). The aorta was removed and cleaned of adherent connective tissue. The thoracic aorta (from 0.5 cm below aortic arch) was cut into 3 mm ring in length and immediately placed into ice-cold modified Krebs-Ringer bicarbonate solution (composition in mM: NaCl 118, KCl 4.7, CaCl2 2.5, MgSO4 1.2, KH2PO4 1.2, NaHCO3 25, and glucose 11.1). In some rings, endothelium was mechanically removed by gentle rubbing of the intimate surface with a hair. The aortic rings were mounted horizontally between 2 steel wires in an organ bath (MO-720; DMT A/S, Aarhus, Denmark) with 5 ml of Krebs’ buffer bubbled with the mixed gas of 95% O2 and 5% CO2 at 37°C. One wire was connected to an isometric force transducer. The rings were equilibrated under a resting tension of 1 gr for 60 min and were exposed 2 times to KSS solution containing 60 mM KCl at 30 min intervals to induced contraction. In some experiments, the aortic tissues were preincubated with 100 nM wortmannin (a specific phosphatidylinositol 3-kinase [PI3K] inhibitor; Selleck, Houston, TX, USA), 100 nM atosiban (an oxytocin receptor antagonist), or 50 mM PD98059 (an inhibitor of ERK1/2 activation) for 30 min before oxytocin incubation. Aortic tissues used for Western blot were incubated with oxytocin (100 nM) for 15 min at 37°C, using cell culture medium bubbled with the mixed gas of 95% O2 and 5% CO2. The tissues were harvested and snap frozen in liquid nitrogen.
Oxytocin-induced vasorelaxation was examined by organ bath as previously described [15]. Briefly, the aortic rings were precontracted with 70% of maximal norepinephrine-induced vasoconstriction (about 30 nM norepinephrine). A cumulative dose of oxytocin (10-11–10-7 M) was then added into an organ chamber. In some experiments, the aortic rings were incubated with the following chemicals for 30 min:100 μM NG-nitric-L-arginine methyl ester (L-NAME, a nitric oxide synthase inhibitor), 100 nM wortmannin, or 100 nM atosiban before norepinephrine and oxytocin were added into organ bath. Wortmannin and atosiban were dissolved in dimethylsulfoxide (DMSO). Final concentration of DMSO was less than 0.1%. The aortic rings in the control oxytocin group were preincubated with 0.1% DMSO for 30 min. Oxytocin-induced vasorelaxation was expressed as the percentage inhibition of 30 nM norepinephrine-induced vasoconstriction.
Oxytocin-induced vasoconstriction was examined in the endothelium-intact rings. A cumulative dose of oxytocin (10-11–10-7 M) was added in the unstimulated aortic rings at resting ten­sion of 1 g. In some experiments, the aortic rings were preincubated with following reagents for 30 min, including 100 nM L-NAME, 100 nM atosiban, 50 μM PD98059, or 1 μM SQ29584 (a prostaglandin H2 [PGH2]/thromboxane A2 [TXB A2] receptor antagonist) to block PGH2/TXB A2, PGH2/TXB A2 is one of the most common endothelium-derived constricting factor. Oxytocin-induced vasoconstricting response was also examined in the aortic rings with endothelium removal. PD98059 or SQ29584 was dissolved in DMSO solvent, in these experiments, the aortic rings in control group were preincubated with 0.1% DMSO for 30 min. Oxytocin-induced vasoconstriction was expressed as percentage of 60 mM KCl-induced vasoconstriction.
HUVECs (ATCC TIB 202; ATCC, Manassas, VA, USA) were cultured in Dulbecco’s modified eagle medium (DMEM) medium supplemented with 10% fetal bovine serum. The cells were cultured at 37°C, 95% humidity and 5% CO2 and used between passages 4 and 16. The cells were seeded in six-well plates (106 cells/well) and starved in serum free DMEM medium for 24 h before the experiments were performed. The cells were incubated with oxytocin (10-10–10-7 M) for 1 to 15 min to determine a time course or dose-dependent peNOS or pAkt expressions. For all other experiments, the cell was treated with 10 nM oxytocin for 5 min. In some experiments, the cells were preincubated with 10 mM wortmannin or 100 nM atosiban for 30 min to block PI3K activity or oxytocin receptor. The cells in control group were incubated with 0.1% DMSO for 30 min.
The aortic tissues were homogenized with lysis buffer containing protease inhibitors including 10 μg/ml aprotinin, 10 μg/ml leupeptin and 1 mM phenylmethylsulphonyl fluoride (PMSF). The protein concentration was determined by the Bio-Rad protein assay. Thirty μg of proteins of the supernatants were loaded and separated by SDS-PAGE. The proteins were transferred to a nitrocellulose membrane. The membrane was blocked with 5% (w/v) milk in the 0.1% Tween/Tris-Buffered Saline (TBS) buffer for 1 h at room temperature. The membrane was incubated with specific primary antibodies against eNOS (1:1,000 dilution; Cell Signaling Technology, Danvers, MA, USA), peNOS (Ser 1177, 1:1,000; Cell Signaling Technology), Akt (1:2,000; Cell Signaling Technology), pAkt (Tyr 473, 1:2,000; Cell Signaling Technology), ERK1/2 (1:1,000) and pERK1/2 (1:1,000; Cell Signaling Technology) at 4°C overnight. After washing, the membranes were incubated with the appropriate secondary antibody against the primary antibody for 1 h at room temperature. The signal for labeled proteins were detected by luminal chemiluminescence. Using the Bio-Rad system, the images were quantified by Image J software. β-actin (1:500; Santa Cruz Biotech, Santa Cruz, CA, USA) was used as an equal protein loading control. The data was normalized to β-actin or correspondence unphosphorylated proteins and expressed as fold increase versus control group.
Statistical analyses were performed by ANOVA with Bonferroni’s correction for multiple comparisons using GraphPad statistical software package (GraphPad Software Inc., San Diego, CA, USA).
All experimental results were expressed as mean ± SEM. Statistical analyses were performed by ANOVA with Bonferroni’s correction for multiple comparisons. Values were considered significant when p < 0.05.
To investigate the effect of oxytocin on eNOS/phospho-eNOS expression, HUVECs were incubated with oxytocin (10-10 to 10-7 M) for 1 to 15 min. As shown in Fig. 1, oxytocin (10 nM) significantly increased the expression of phospho-eNOS (Ser 1177) in a time-dependent manner, starting at the first minute, reaching a peak at 5 min and continuing a plateau at 15 min (Fig. 1A). Incubation of oxytocin for 5 min also dose-dependently increased phospho-eNOS expression with a maximal effect at 100 nM (Fig. 1C). However, oxytocin did not affect the protein expression of total eNOS (Fig. 1B, D). To investigate the signaling pathway that oxytocin promotes eNOS phosphorylation, we determined Akt and pAkt (Tyr473) expression in HUVECs in vitro, oxytocin dose-dependently increased pAkt (Tyr 473) expression but did not significantly affect total Akt expression in HUVECs in vitro (Fig. 2A). Furthermore, either PI3K inhibitor wortmannin or oxytocin receptor antagonist atosiban can prevent oxytocin-induced Akt or eNOS phosphorylation in HUVECs in vitro (Fig. 2B, C). These results suggest that oxytocin stimulates eNOS phosphorylation through the oxytocin receptor-mediated PI3K/Akt pathway.
To investigate whether oxytocin induced eNOS phosphorylation ex vivo, the aorta was incubated with oxytocin (100 nM) for 15 min. Consistent with the findings in the cultured HUVECs in vitro, oxytocin significantly increased the expressions of phospho-Akt (Tyr473) and phospho-eNOS (Ser 1177) but did not significantly affect the expressions of total Akt or eNOS expression in rat aorta ex vivo (Fig. 3A, B). The preincubation with wortmannin or atosiban prevented oxytocin-induced phospho-Akt and phospho-eNOS in the aorta (Fig. 3C, D).
In the aortic rings precontracted with 30 nM norepinephrine, oxytocin induced a biphasic vascular response: vasorelaxation at low dose (10-9 to 10-8 M) of oxytocin with maximal relaxation of about 23% inhibition of norepinephrine-induced vasocontraction. However, oxytocin at a high dose (10-7 M) evoked a constriction response. Either L-NAME or endothelial removal abolished oxytocin-induced vasorelaxation and slightly increased oxytocin-induced vasoconstriction (Fig. 4A). Wortmannin also inhibited oxytocin-induced vasorelaxation and slightly enhanced oxytocin-induced vasoconstriction. Oxytocin receptor antagonist atosiban abolished oxytocin-induced vasorelaxation or vasoconstriction (Fig. 4). These results suggest that oxytocin induces vasorelaxation by stimulation of PI3K/eNOS pathway.
Oxytocin evoked a dose-dependent contraction response in the unstimulated aortic rings. Oxytocin-induced vasoconstriction started at 10 nM oxytocin and reached a maximal vasocontraction response at 100 nM, which is about 20% of 60 mM KCl-induced contraction. Both L-NAME and endothelium removal slightly but significantly enhanced the contraction response. PGH2/TXB A2 receptor antagonist SQ29584 did not affect the vasoconstriction. Oxytocin receptor antagonist atoshiban completely abolished the contraction, and ERK1/2 activation inhibitor PD98059 partially attenuated oxytocin-induced vasocontraction (Fig. 5A–E). Furthermore, we determined phospho-ERK1/2 in the aorta which was incubated with 100 nM oxytocin for 15 min ex vivo. Oxytocin significantly increased ERK1/2 phosphorylation. Atosiban markedly attenuated oxytocin-induced ERK1/2 phosphorylation, and PD98059 completely prevented the increase in oxytocin-induced phospho-ERK1/2 expression (Fig. 5F). Because atosiban is an inhibitor of oxytocin receptor and vasopressin 1a receptor (OTR and V1aR), thus the results suggest that the oxytocin-induced vasocontraction was at least partly mediated by OTR/V1aR-induced ERK1/2 activation.
It has been shown that oxytocin has a great number of effects on the cardiovascular system. Major findings for the present study are: 1) in the isolated aortic rings, oxytocin induces biphasic effects on vascular reactivities, oxytocin in low dose (1–10 nM) induces vasorelaxation and high dose of oxytocin (100 nM) triggers vasoconstriction; 2) oxytocin induces vasorelaxation via stimulation of OTR-mediated activation of Akt/eNOS pathway and vasoconstriction via OTR/V1aR-mediated activation of ERK1/2; 3) oxytocin-induced vascular reactivity are dose-dependent, the dose of oxytocin initiation of vasoconstriction is almost 100 time higher than that of oxytocin-induced vasorelaxation. In addition, the dose for oxytocin activation of Akt/eNOS pathway is also much high than oxytocin activation of ERK1/2, which may have important physiological significance, because NO is a vasoprotective molecule, and the activation of ERK1/2 is usually considered to worsen vascular function. Thus low concentration of oxytocin-induced NO production may participate in the maintenance of normal cardiovascular homeostasis, whereas ERK1/2 activated by high concentration of oxytocin may be associated with cardiovascular dysfunction in some pathophysiological conditions.
It has been proposed that oxytocin may participate in the maintenance of normal homeostatic functions of cardiovascular system [1,16,17]. Both the heart and the vessels can synthesize oxytocin and express oxytocin receptor. Oxytocin may increase NO generation in cardiovascular system and has antioxidant effects to reduce reactive oxygen species synthesis [18,19]. Oxytocin may exert therapeutic effects on cardiovascular diseases, chronic administration of oxytocin reduces atherosclerotic lesion formation and vascular inflammation in Watanabe Heritable Hyperlipidemic Rabbits [20]. Long-term treatment with oxytocin can protect against ischemia/reperfusion-induced cardiac injury in vivo, which was partially reversed by L-NAME [17,21]. Oxytocin can improve cardiac function and remodeling and reduced myocardial infarction (MI) size associated with increased eNOS expression in the scar area of acute MI rats [19,22]. In the present study, we demonstrate that oxytocin can stimulate eNOS phosphorylation in vascular endothelium [23]. Furthermore, we demonstrate that oxytocin induces eNOS phosphorylation via oxytocin receptor-mediated activation of PI3K/Akt pathway, because both PI3K inhibitor wortmannin and oxytocin receptor antagonist atosiban can prevent oxytocin-induced eNOS phosphorylation. There are several articles in the literatures to report oxytocin can activate PI3K/Akt/eNOS pathway, for example, Cattaneo et al. [24] showed that oxytocin stimulated Akt/eNOS phosphorylation, which may increase HUVECs proliferation and angiogenesis. Florian et al. [25] showed that oxytocin increased glucose uptake in neonatal rat cardiomyocytes by stimulating PI3K/eNOS pathway, because inhibition of PI3K by wortmannin suppressed oxytocin-induced increase in glucose uptake. NO is an important cardiovascular protective molecule. NO can induce vasorelaxation, inhibit the aggregation of platelet and the proliferation of vascular smooth muscle [26]. Oxytocin is a circulating hormone, continuous stimulation of endothelial NO production by circulating oxytocin may play an important role for the maintenance of cardiovascular homeostasis. The increased endothelium eNOS-derived NO may be one of important mechanisms to explain oxytocin’s therapeutic effects in some cardiovascular pathological conditions.
Vascular oxytocin may play an important role in the regulation of vascular tone and blood pressure. It has been reported that oxytocin can induce either vasorelaxation or vasoconstriction, depending on vascular beds and species [14,27]. Systemic administration of oxytocin has been reported to decrease blood pressure in hypertensive rats [9]. In the present study, we demonstrate that oxytocin induces a biphasic vascular response in the rat aorta, vasorelaxation in low dose and vasoconstriction in high dose. Oxytocin induces vasorelaxation via stimulating Akt/eNOS-derived NO in endothelium, because the blockade of eNOS by L-NAME or removal of endothelium prevents oxytocin-induced vasorelaxation. On the other hand, a high dose of oxytocin induces vasoconstriction and ERK1/2 phosphorylation. Either ERK1/2 inhibitor PD98059 or OTR/V1aR antagonist atosiban prevents oxytocin-induced vasoconstriction and ERK1/2 activation [28]. It has been reported that oxytocin can activation ERK1/2 pathway in neural cells and myometrial cells, but there is no report in the literature that oxytocin can activate ERK1/2 to regulate vascular tone [29,30]. Therefore, our results suggest that oxytocin-induced vasorelaxation appears to be mediated by the activation of OTR/V1aR-induced Akt/eNOS pathway and vasoconstriction to oxytocin is partially mediated by OTR/V1aR-ERK1/2 pathway.
In summary, in the present study, we demonstrate that oxytocin induces eNOS phosphorylation and vasorelaxation via the activation of OTR/PI3K/Akt pathway. On the other hand, a high dose of oxytocin evokes a vasoconstriction response, which is partially mediated by OTR/ViaR-ERK1/2 pathway. The biphasic vascular reactivity of oxytocin and its capability to increase endothelium NO production may help us to understand the role of oxytocin in maintenance of cardiovascular homeostasis and regulation of vascular tone.
Notes
REFERENCES
1. Jurek B, Neumann ID. 2018; The oxytocin receptor: from intracellular signaling to behavior. Physiol Rev. 98:1805–1908. DOI: 10.1152/physrev.00031.2017. PMID: 29897293. PMID: https://www.scopus.com/inward/record.uri?partnerID=HzOxMe3b&scp=85048730134&origin=inward.


2. Jankowski M, Broderick TL, Gutkowska J. 2020; The role of oxytocin in cardiovascular protection. Front Psychol. 11:2139. DOI: 10.3389/fpsyg.2020.02139. PMID: 32982875. PMCID: PMC7477297. PMID: 9bb5c602a4d44bc881e1fbc70afc72d5. PMID: https://www.scopus.com/inward/record.uri?partnerID=HzOxMe3b&scp=85090777237&origin=inward.


3. Szczepanska-Sadowska E, Wsol A, Cudnoch-Jedrzejewska A, Żera T. 2021; Complementary role of oxytocin and vasopressin in cardiovascular regulation. Int J Mol Sci. 22:11465. DOI: 10.3390/ijms222111465. PMID: 34768894. PMCID: PMC8584236. PMID: ca08fa6e6f4040b1aabddb4b719c4eb9. PMID: https://www.scopus.com/inward/record.uri?partnerID=HzOxMe3b&scp=85117595253&origin=inward.


4. McKay EC, Counts SE. 2020; Oxytocin receptor signaling in vascular function and stroke. Front Neurosci. 14:574499. DOI: 10.3389/fnins.2020.574499. PMID: 33071746. PMCID: PMC7544744. PMID: e3b8178eaa9d4cd08db8550fff61dfed. PMID: https://www.scopus.com/inward/record.uri?partnerID=HzOxMe3b&scp=85092278060&origin=inward.


5. Liu S, Pan S, Tan J, Zhao W, Liu F. 2017; Oxytocin inhibits ox-LDL-induced adhesion of monocytic THP-1 cells to human brain microvascular endothelial cells. Toxicol Appl Pharmacol. 337:104–110. DOI: 10.1016/j.taap.2017.10.022. PMID: 29104011. PMID: https://www.scopus.com/inward/record.uri?partnerID=HzOxMe3b&scp=85033378308&origin=inward.


6. Nation DA, Szeto A, Mendez AJ, Brooks LG, Zaias J, Herderick EE, Gonzales J, Noller CM, Schneiderman N, McCabe PM. 2010; Oxytocin attenuates atherosclerosis and adipose tissue inflammation in socially isolated ApoE-/- mice. Psychosom Med. 72:376–382. DOI: 10.1097/PSY.0b013e3181d74c48. PMID: 20368478. PMCID: PMC4784697. PMID: https://www.scopus.com/inward/record.uri?partnerID=HzOxMe3b&scp=77952425954&origin=inward.


7. Japundžić-Žigon N, Lozić M, Šarenac O, Murphy D. 2020; Vasopressin & oxytocin in control of the cardiovascular system: an updated review. Curr Neuropharmacol. 18:14–33. DOI: 10.2174/1570159X17666190717150501. PMID: 31544693. PMCID: PMC7327933. PMID: https://www.scopus.com/inward/record.uri?partnerID=HzOxMe3b&scp=85077294829&origin=inward.


8. Petersson M, Lundeberg T, Uvnäs-Moberg K. 1997; Oxytocin decreases blood pressure in male but not in female spontaneously hypertensive rats. J Auton Nerv Syst. 66:15–18. DOI: 10.1016/S0165-1838(97)00040-4. PMID: https://www.scopus.com/inward/record.uri?partnerID=HzOxMe3b&scp=0031563516&origin=inward.


9. Gutkowska J, Aliou Y, Lavoie JL, Gaab K, Jankowski M, Broderick TL. 2016; Oxytocin decreases diurnal and nocturnal arterial blood pressure in the conscious unrestrained spontaneously hypertensive rat. Pathophysiology. 23:111–121. DOI: 10.1016/j.pathophys.2016.03.003. PMID: 27020751. PMID: https://www.scopus.com/inward/record.uri?partnerID=HzOxMe3b&scp=84962184528&origin=inward.


10. Somjit M, Surojananon J, Kongwattanakul K, Kasemsiri C, Sirisom M, Prawannoa K, Thepsuthammarat K, Komwilaisak R. 2020; Comparison of low dose versus high dose of oxytocin for initiating uterine contraction during cesarean delivery: a randomized, controlled, non-inferiority trial. Int J Womens Health. 12:667–673. DOI: 10.2147/IJWH.S260073. PMID: 32904472. PMCID: PMC7455765. PMID: https://www.scopus.com/inward/record.uri?partnerID=HzOxMe3b&scp=85090506827&origin=inward.
11. Wsol A, Wojno O, Puchalska L, Wrzesien R, Szczepanska-Sadowska E, Cudnoch-Jedrzejewska A. 2020; Impaired hypotensive effects of centrally acting oxytocin in SHR and WKY rats exposed to chronic mild stress. Am J Physiol Regul Integr Comp Physiol. 318:R160–R172. DOI: 10.1152/ajpregu.00050.2019. PMID: 31644319. PMID: https://www.scopus.com/inward/record.uri?partnerID=HzOxMe3b&scp=85077762246&origin=inward.


12. Oyama H, Suzuki Y, Satoh S, Kajita Y, Takayasu M, Shibuya M, Sugita K. 1993; Role of nitric oxide in the cerebral vasodilatory responses to vasopressin and oxytocin in dogs. J Cereb Blood Flow Metab. 13:285–290. DOI: 10.1038/jcbfm.1993.35. PMID: 8436620. PMID: https://www.scopus.com/inward/record.uri?partnerID=HzOxMe3b&scp=0027476776&origin=inward.


13. Suzuki Y, Satoh S, Kimura M, Oyama H, Asano T, Shibuya M, Sugita K. 1992; Effects of vasopressin and oxytocin on canine cerebral circulation in vivo. J Neurosurg. 77:424–431. DOI: 10.3171/jns.1992.77.3.0424. PMID: 1506890. PMID: https://www.scopus.com/inward/record.uri?partnerID=HzOxMe3b&scp=0026641189&origin=inward.


14. Katusic ZS, Shepherd JT, Vanhoutte PM. 1986; Oxytocin causes endothelium-dependent relaxations of canine basilar arteries by activating V1-vasopressinergic receptors. J Pharmacol Exp Ther. 236:166–170. PMID: 3001282. PMID: https://www.scopus.com/inward/record.uri?partnerID=HzOxMe3b&scp=0022645675&origin=inward.
15. Cai R, Hao Y, Liu YY, Huang L, Yao Y, Zhou MS. 2020; Tumor necrosis factor alpha deficiency improves endothelial function and cardiovascular injury in deoxycorticosterone acetate/salt-hypertensive mice. Biomed Res Int. 2020:3921074. DOI: 10.1155/2020/3921074. PMID: 32190663. PMCID: PMC7064859. PMID: https://www.scopus.com/inward/record.uri?partnerID=HzOxMe3b&scp=85082006872&origin=inward.


16. Reiss AB, Glass DS, Lam E, Glass AD, De Leon J, Kasselman LJ. 2019; Oxytocin: potential to mitigate cardiovascular risk. Peptides. 117:170089. DOI: 10.1016/j.peptides.2019.05.001. PMID: 31112739. PMID: https://www.scopus.com/inward/record.uri?partnerID=HzOxMe3b&scp=85067972878&origin=inward.


17. Faghihi M, Alizadeh AM, Khori V, Latifpour M, Khodayari S. 2012; The role of nitric oxide, reactive oxygen species, and protein kinase C in oxytocin-induced cardioprotection in ischemic rat heart. Peptides. 37:314–319. DOI: 10.1016/j.peptides.2012.08.001. PMID: 22902709. PMID: https://www.scopus.com/inward/record.uri?partnerID=HzOxMe3b&scp=84865337214&origin=inward.


18. Szeto A, Nation DA, Mendez AJ, Dominguez-Bendala J, Brooks LG, Schneiderman N, McCabe PM. 2008; Oxytocin attenuates NADPH-dependent superoxide activity and IL-6 secretion in macrophages and vascular cells. Am J Physiol Endocrinol Metab. 295:E1495–E1501. DOI: 10.1152/ajpendo.90718.2008. PMID: 18940936. PMCID: PMC2603556. PMID: https://www.scopus.com/inward/record.uri?partnerID=HzOxMe3b&scp=57349088269&origin=inward.


19. Hussien NI, Mousa AM. 2016; Could nitric oxide be a mediator of action of oxytocin on myocardial injury in rats? (biochemical, histological and immunohistochemical study). Gen Physiol Biophys. 35:353–362. DOI: 10.4149/gpb_2015049. PMID: 27226256. PMID: https://www.scopus.com/inward/record.uri?partnerID=HzOxMe3b&scp=84974628199&origin=inward.


20. Szeto A, Rossetti MA, Mendez AJ, Noller CM, Herderick EE, Gonzales JA, Schneiderman N, McCabe PM. 2013; Oxytocin administration attenuates atherosclerosis and inflammation in Watanabe Heritable Hyperlipidemic rabbits. Psychoneuroendocrinology. 38:685–693. DOI: 10.1016/j.psyneuen.2012.08.009. PMID: 22998949. PMCID: PMC3543511. PMID: https://www.scopus.com/inward/record.uri?partnerID=HzOxMe3b&scp=84876090828&origin=inward.


21. Gonzalez-Reyes A, Menaouar A, Yip D, Danalache B, Plante E, Noiseux N, Gutkowska J, Jankowski M. 2015; Molecular mechanisms underlying oxytocin-induced cardiomyocyte protection from simulated ischemia-reperfusion. Mol Cell Endocrinol. 412:170–181. DOI: 10.1016/j.mce.2015.04.028. PMID: 25963797. PMID: https://www.scopus.com/inward/record.uri?partnerID=HzOxMe3b&scp=84939573492&origin=inward.


22. Anvari MA, Imani A, Faghihi M, Karimian SM, Moghimian M, Khansari M. 2012; The administration of oxytocin during early reperfusion, dose-dependently protects the isolated male rat heart against ischemia/reperfusion injury. Eur J Pharmacol. 682:137–141. DOI: 10.1016/j.ejphar.2012.02.029. PMID: 22406244. PMID: https://www.scopus.com/inward/record.uri?partnerID=HzOxMe3b&scp=84859110002&origin=inward.


23. Gutkowska J, Jankowski M, Antunes-Rodrigues J. 2014; The role of oxytocin in cardiovascular regulation. Braz J Med Biol Res. 47:206–214. DOI: 10.1590/1414-431X20133309. PMID: 24676493. PMCID: PMC3982941. PMID: https://www.scopus.com/inward/record.uri?partnerID=HzOxMe3b&scp=84898896689&origin=inward.


24. Cattaneo MG, Chini B, Vicentini LM. 2008; Oxytocin stimulates migration and invasion in human endothelial cells. Br J Pharmacol. 153:728–736. DOI: 10.1038/sj.bjp.0707609. PMID: 18059319. PMCID: PMC2259201. PMID: https://www.scopus.com/inward/record.uri?partnerID=HzOxMe3b&scp=39449121993&origin=inward.


25. Florian M, Jankowski M, Gutkowska J. 2010; Oxytocin increases glucose uptake in neonatal rat cardiomyocytes. Endocrinology. 151:482–491. DOI: 10.1210/en.2009-0624. PMID: 20008042. PMID: https://www.scopus.com/inward/record.uri?partnerID=HzOxMe3b&scp=74949108334&origin=inward.


26. Zhou MS, Schulman IH, Raij L. 2004; Nitric oxide, angiotensin II, and hypertension. Semin Nephrol. 24:366–378. DOI: 10.1016/j.semnephrol.2004.04.008. PMID: 15252776. PMID: https://www.scopus.com/inward/record.uri?partnerID=HzOxMe3b&scp=3142540898&origin=inward.


27. Japundžić-Žigon N. 2013; Vasopressin and oxytocin in control of the cardiovascular system. Curr Neuropharmacol. 11:218–230. DOI: 10.2174/1570159X11311020008. PMID: 23997756. PMCID: PMC3637675. PMID: https://www.scopus.com/inward/record.uri?partnerID=HzOxMe3b&scp=84876703862&origin=inward.


28. Wiśniewski K. 2019; Design of oxytocin analogs. Methods Mol Biol. 2001:235–271. DOI: 10.1007/978-1-4939-9504-2_11. PMID: 31134574. PMID: https://www.scopus.com/inward/record.uri?partnerID=HzOxMe3b&scp=85066281457&origin=inward.


29. Zhong M, Yang M, Sanborn BM. 2003; Extracellular signal-regulated kinase 1/2 activation by myometrial oxytocin receptor involves GαqGβγ and epidermal growth factor receptor tyrosine kinase activation. Endocrinology. 144:2947–2956. DOI: 10.1210/en.2002-221039. PMID: 12810550. PMID: https://www.scopus.com/inward/record.uri?partnerID=HzOxMe3b&scp=0038675282&origin=inward.


30. Wang P, Qin D, Wang YF. 2017; Oxytocin rapidly changes astrocytic GFAP plasticity by differentially modulating the expressions of pERK 1/2 and protein kinase A. Front Mol Neurosci. 10:262. DOI: 10.3389/fnmol.2017.00262. PMID: 28860967. PMCID: PMC5559427. PMID: https://www.scopus.com/inward/record.uri?partnerID=HzOxMe3b&scp=85027869582&origin=inward.


Fig. 1
Effects of oxytocin on the protein expressions of phospho-endothelial nitric oxide synthesis (peNOS, Ser 1177) and eNOS in HUVECs.
Oxytocin time-dependently increased peNOS (A) but not total eNOS expression (B), oxytocin dose-dependently increased peNOS (C) but not total eNOS expression (D). Data is expressed as mean ± SE. n = 6. HUVECs, human umbilical vein endothelial cells; OT, oxytocin. *p < 0.05 vs. control group.
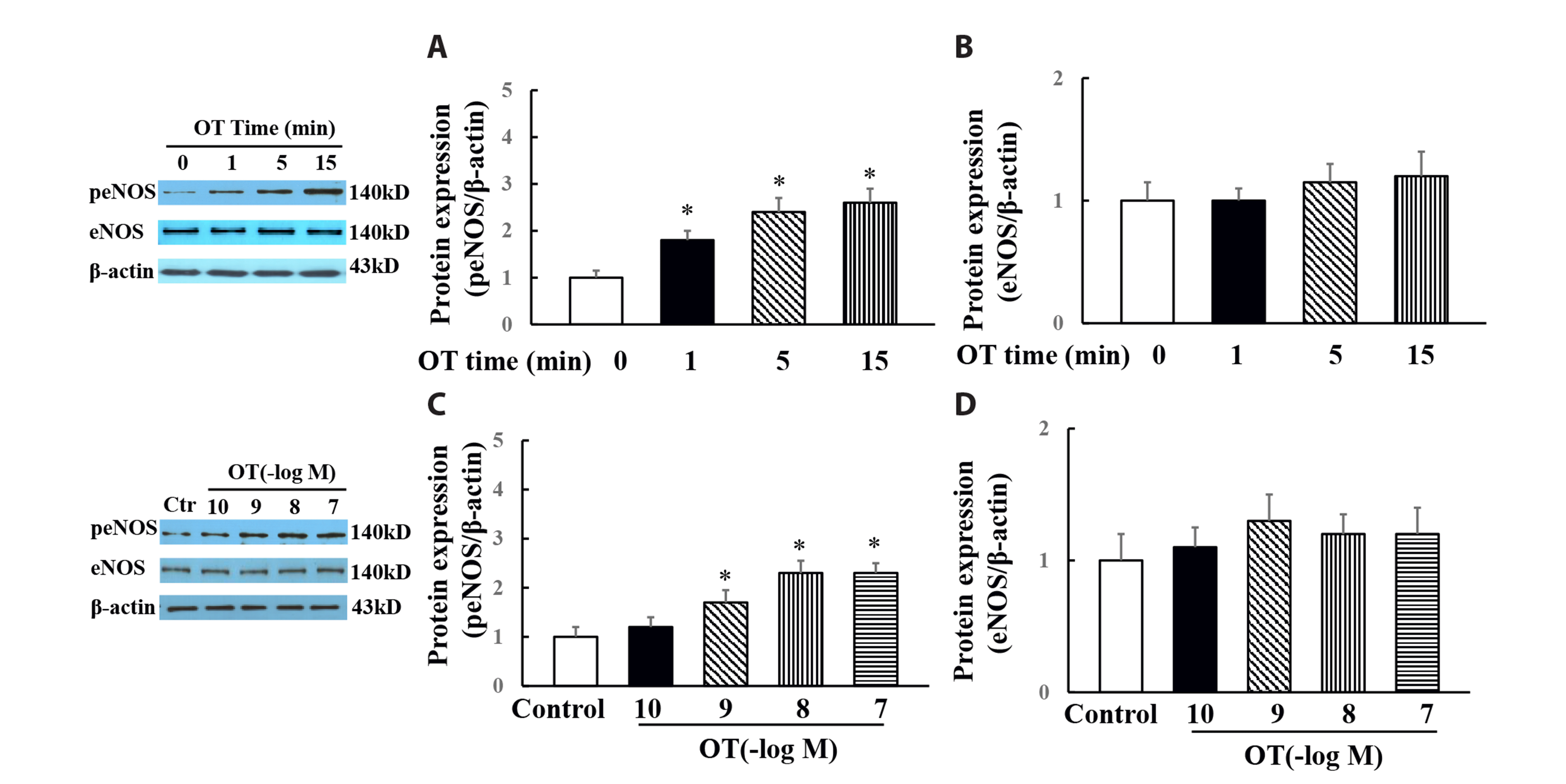
Fig. 2
Effects of PI3K inhibitor wortmannin or oxytocin/vasopressin 1a receptor antagonist atosiban on pAkt (tyr473) or peNOS (ser1177) in the cultured HUVECs.
Oxytocin dose-dependently increased Akt phosphorylation (A); Either wortmannin or atosiban significantly attenuated oxytocin-induced Akt (B) or eNOS phosphorylation (C). PI3K, phosphatidylinositol 3-kinase; HUVECs, human umbilical vein endothelial cells; OT, oxytocin; Wort, wortmannin; Ato, atosiban. n = 6, *p < 0.05 vs. control group, #p < 0.05 vs. oxytocin group.
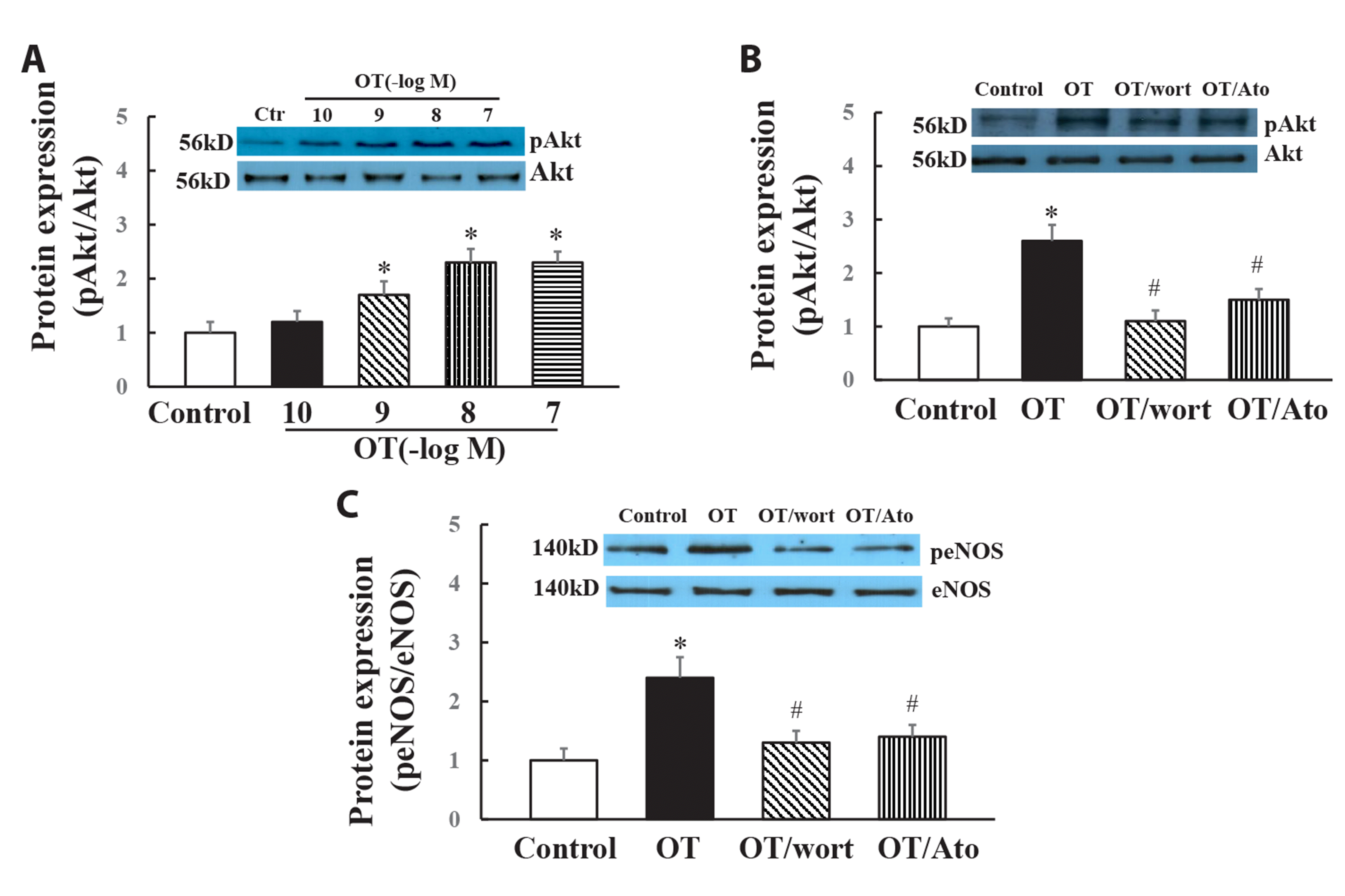
Fig. 3
Effects of oxytocin on pAkt (Tyr 473) and peNOS (Ser 1177) expressions in the rat aorta ex vivo.
Incubation of oxytocin (10 nM) for 5 min ex vivo significantly increased pAkt (Tyr 473, A) and peNOS (Ser 1177, B) expression. Either wortmannin or atosiban prevented oxytocin-indued pAkt (C) and peNOS (D) expression. OT, oxytocin; Wort, wortmannin; Ato, atosiban. n = 8, *p < 0.05 vs. control group; #p < 0.05 vs. oxytocin group.

Fig. 4
Effect of oxytocin (OT) on the vascular reactivity in the rat’s aortic rings pre-constricted by norepinephrine.
(A) L-NAME (an inhibitor of nitric oxide synthase) or endothelium removal (EC–) abolished oxytocin-induced vasorelaxation and slightly enhanced oxytocin-induced vasoconstriction; (B) Atosiban or wortmannin abolished oxytocin-induced vasorelaxation. n = 8. *p < 0.05 vs. oxytocin group.
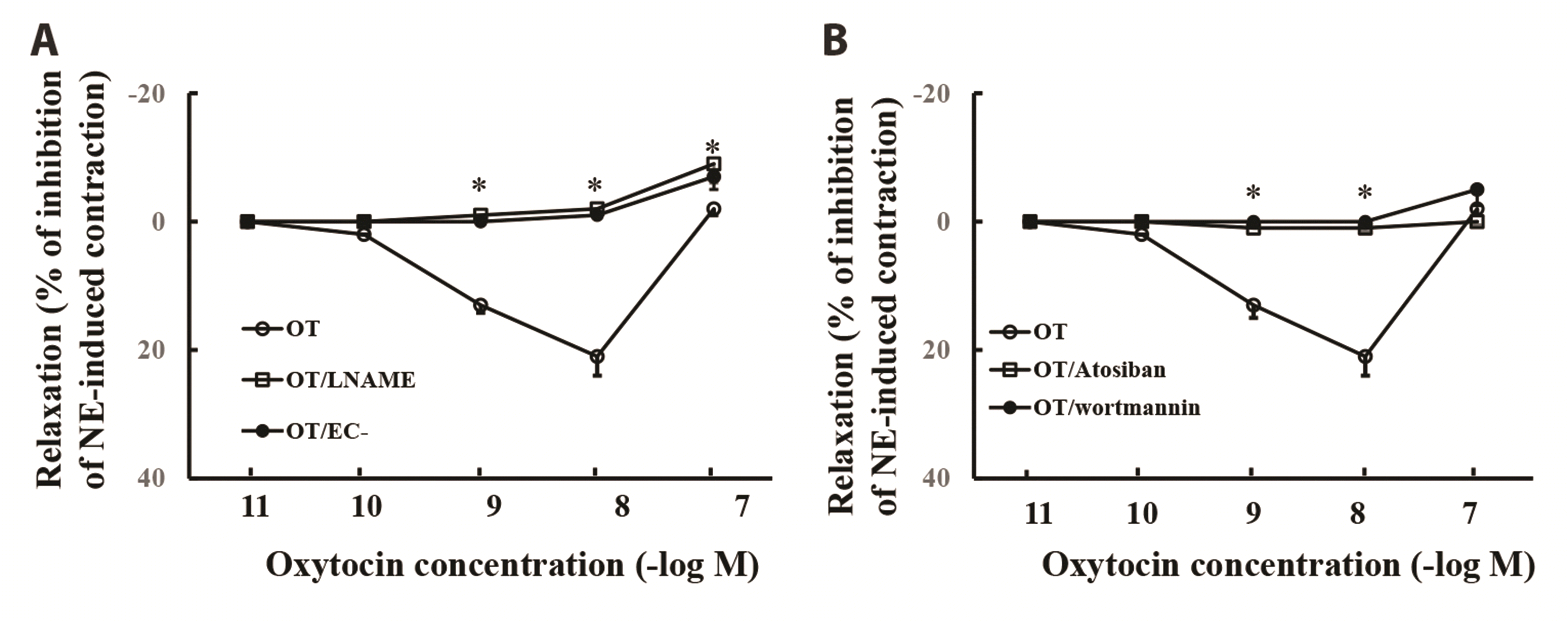
Fig. 5
Oxytocin induced vasoconstriction in the unstimulated rat’s aortic rings.
L-NAME (an inhibitor of nitric oxide synthase) (A) or endothelium removal (B) slightly enhanced oxytocin-induced vasoconstriction; (C) SQ29548 (an inhibitor of prostaglandin H2/thromboxane A2) did not affect oxytocin-induced vasoconstriction; (D) Atosiban completely abolished oxytocin-induced vasoconstriction; (E) PD98059 (an inhibitor of ERK1/2 activation) partially inhibited oxytocin-induced vasoconstriction; (F) Effect of oxytocin on phospho-ERK1/2 expression. OT, oxytocin; Ato, atosiban. n = 8. *p < 0.05 vs. control group, #p < 0.05 vs. oxytocin group.
