Abstract
Purpose
Although chronotropic incompetence (CI) is common in patients with complex congenital heart disease (CHD) and is associated with adverse cardiovascular outcomes, few data are available regarding modifiable predictors of CI in this escalating patient population. We tested the hypothesis that higher levels of physical activity (PA) are associated with a lower prevalence of CI in patients with complex CHD and evaluated the receiver operating characteristic curve to identify the PA level that best predicted CI.
Methods
We evaluated 111 adolescents with complex CHD. CI was defined as the failure to achieve 80% of the chronotropic response index during peak cardiopulmonary exercise test. Self-reported habitual activity was obtained using a global PA questionnaire.
Results
CI was identified in 45 of the 111 cases (40.5%). After adjusting for potential confounding variables, the high PA group demonstrated a lower odds ratio for having CI (odds ratios, 0.25; 95% confidence interval, 0.06‒0.99) compared with the low PA group. The most accurate cut-point for PA to predict the prevalence of CI was 15 metabolic equivalents (METs)-hours/week (areas under the curve, 0.71; 95% confidence interval, 0.61‒0.81; sensitivity, 71%; specificity, 69%).
An abnormal heart rate response to exercise is clinically significant because of its association with autonomic dysfunction and poor clinical outcomes1. Heart rate increases linearly during progressive exercise due to increases in sympathetic activity coupled with concomitant decreases in parasympathetic stimulation2. However, in some individuals, a blunted or attenuated increase in heart rate during exercise may be observed, signifying “chronotropic incompetence (CI)3,4.” Importantly, CI has been shown to be an independent predictor of subsequent cardiovascular events and a heightened cardiovascular mortality in patients with heart failure5,6. Although CI is also common in patients with complex congenital heart disease (CHD) and is associated with adverse cardiovascular outcomes7-9, this response remains under-appreciated, as few data are available regarding modifiable predictors of CI in this escalating patient population. While there is a much higher prevalence of CI (34%–62%) in patients with CHD, as compared with their healthy age-matched counterparts, the underlying mechanisms responsible for the diminished heart rate reserve remain unclear7-9. Previous studies have suggested that the higher prevalence of CI in patients with CHD may be due to congenital cardiac anomalies, damage to the sinoatrial node during cardiovascular surgical procedures, or both, ultimately resulting in autonomic dysfunction or downregulation/desensitization of the associated beta-adrenergic receptors in the synaptic gap10-12. However, it remains unclear whether certain characteristics are linked with CI and whether these are modifiable in patients with CHD.
Although higher levels of physical activity (PA) are associated with an enhanced exercise capacity, improved cardiovascular risk factor profile, altered autonomic nervous function, and quality of life as well as psychosocial outcomes in patients with CHD13-16, it remains unclear whether it can also attenuate the risk of CI in patients with CHD. Interestingly, maximal heart rate and chronotropic index have been reported to increase following exercise training in patients with heart failure17-21. Accordingly, higher levels of PA may favorably impact the chronotropic response to exercise in patients with heart failure and other cardiovascular diseases22. We tested the hypothesis that higher levels of PA are associated with a lower prevalence of CI in patients with complex CHD, independent of potential risk factors. Additionally, we evaluated the receiver operating characteristic curve to identify the PA level that most accurately predicted CI in patients with complex CHD.
This study was conducted from November 2015 to February 2016 using data collected during outpatient visits to the pediatric department of Sejong General Hospital in Bucheon, Korea. Among 240 adolescents who had a confirmed CHD diagnosis in the medical chart, 228 patients were eligible for the study. Initial exclusions (n=12) included patients with arrhythmias that were unrelated to CHD (n=3) and those with incomplete responses to the PA questionnaire (n=9). Additionally, we excluded a subset of adolescents without complex CHD (n=117) who did not undergo cardiopulmonary exercise test. The remaining 111 patients with complex CHD (61 boys and 50 girls, aged 13–18 years) served as our study population. We obtained patient demographic information, diagnosis of CHD, related medical conditions, cyanosis, prescribed medications, and serial surgery data from the medical record. Written informed consent was obtained from the guardians/parents and patients prior to participation. The study protocol adhered to the standards of the Declaration of Helsinki and was approved by the Clinical Trials Board of Sejong General Hospital (No. 2019-0385).
Peak or symptom-limited cardiopulmonary exercise test was conducted via a Q-Stress TM55 treadmill (Quinton Cardiology Systems, Inc. Bothell, WA, USA), and gas analyzer (TrueOne 2400; ParvoMedics, Salt Lake City, UT, USA) using a breath-by-breath method and analyzed in 15-second intervals. A modified Bruce treadmill protocol was used. Criteria for exercise test termination included: (1) a rating of perceived exertion (6–20 category scale) of >17 (very hard), (2) a peak respiratory exchange ratio (RER) of ≥1.10, (3) the subject requested to stop (i.e., volitional fatigue), or (4) combinations thereof. Peak oxygen uptake, oxygen pulse and RER were directly measured during the progressive exercise test. In addition, we measured oxygen saturation and obtained ratings of perceived exertion every stage and at peak exercise. Systolic and diastolic blood pressures were obtained at rest, during the last 30 seconds of each 3 minutes stage, and at peak or maximal exercise. Prior to the exercise test, participants assumed the supine position, and resting heart rate was recorded for 5 minutes via electrocardiogram monitor electrocardiographic monitoring (Q-Stress). Heart rate was also measured during each submaximal stage and at maximal exercise. The peak or maximal heart rate determined by 12-lead electrocardiograms (Q-4500; Quinton Cardiology Systems) was defined as the highest attained value during the progressive exercise test. The CI index was calculated using the equation: (maximal heart rate–resting heart rate)/(220–age–resting heart rate)×100, and patients who did not attain 80% of the index were classified as having CI23.
Habitual activity (metabolic equivalents [METs]-hours/week) was assessed using the global PA questionnaire24. The survey included 16 questions designed to quantitate PA undertaken in three different environments: work (during school time), transport, and discretionary activity (also known as leisure or recreation). METs were used to quantitate the intensity of physical activities that were documented in the global PA questionnaire. For analytical purposes, 4 METs were assigned to the time spent during moderate-intensity activities, and 8 METs to the time spent during vigorous activities. To assess overall PA, MET scores were calculated separately for individual domains and subdomains. For the calculation of a categorical indicator, the total time spent participating in PA during a typical week, the number of days per week, as well as the intensity of PA were considered. To evaluate the reproducibility of the questionnaire, the same questionnaires were administered repeatedly to 14 patients with CHD, yielding correlation coefficients of 0.66 to 0.99. Patients were then divided into groups according to tertiles of PA levels as low (≤10.0 METs-hours/week); moderate (10.1–23.9 METs-hours/week); and high (≥24.0 METs-hours/week).
All values are reported as mean±standard deviation, or frequency and percentage, and normal distribution was assessed using the Kolmogorov-Smirnov test. To compare the differences between the groups with and without CI, Student t-test and Mann-Whitney U-test were employed. Multivariable logistic regression analyses were used to calculate odds ratios and 95% confidence interval of having CI. Because of multicollinearity between PA and peak oxygen uptake (r=0.51, p<0.001), we excluded the latter as an independent variable in the model. Finally, the receiver operating characteristic curve analysis was performed to identify the PA level that most accurately predicted CI. The areas under the curve (AUC) and 95% confidence interval were also obtained. Statistical comparisons were made using the IBM SPSS version 22.0 for Windows (IBM Corp., Armonk, NY, USA), and significance was set at p<0.05.
General characteristics of the 111 subjects are shown in Table 1. The prevalence of CI was identified in 45 of the 111 cases (40.5%). By disease category, CI was most frequent in patients with Fontan disease (58.3%), followed by tetralogy of Fallot (20.6%). Differences in physical and clinical characteristics between the CI and control (No CI) groups are depicted in Table 2. There were no significant differences in age, sex, body mass index, and birth weight between groups. Furthermore, resting heart rate, resting oxygen saturation, peak systolic blood pressure, peak diastolic blood pressure, peak oxygen pulse, and peak rate of perceived exertion did not differ between groups. However, the group with CI demonstrated a higher prevalence of cyanosis (68.4% vs. 31.6%, p=0.012), a lower peak oxygen uptake (27.3±7.3 mL/kg/min vs. 34.4±7.6 mL/kg/min, p<0.001), a higher number of surgical operations (3.9±1.9 vs. 2.1±1.1, p<0.001) and medications (2.2±2.1 vs. 0.9±1.2, p<0.001) than the control group (i.e., No CI). Moreover, the CI group showed a significantly lower peak RER value (1.09±0.10 vs. 1.15±0.10) and PA level than the control group (10.7, 7.3–16. vs. 22.8, 11.5–36.7 METs-hours/week, p<0.001).
In an unadjusted logistic regression model, the high PA group had a lower odds ratio for having CI (odds ratio, 0.18; 95% confidence interval, 0.06–0.51) compared with the low PA group. After adjusting for age, sex, body mass index, birth weight, cyanosis, RER, number of surgical procedures, and number of medications, the high PA group was approximately 75% less likely to have CI than the low PA group (odds ratio, 0.25; 95% confidence interval, 0.06–0.99) (Table 3).
In the multivariate logistic regression analysis, we found that CI was independently related to the number of operations, the number of medications, and the level of PA (each 4 MET increment) (Table 4). The best cut-point of PA levels for predicting the prevalence of CI was 15 METs-hours/week (AUC, 0.71; 95% confidence interval, 0.61–0.81; sensitivity, 71%; and specificity, 69%; p<0.001) (Fig. 1). Patients with <15 METs-hours/week showed a 3.3-fold higher prevalence of CI than patients with >15 METs-hours/week (odd ratio, 3.33; 95% confidence interval, 1.24–8.97, p<0.001) (data not shown).
CI, which is signified by a blunted increase in heart rate during incremental exercise test, is associated with a poor prognosis in patients with CHD7-9. Although the attenuated heart rate response is generally attributed to the associated cardiovascular surgery, identifying potential modulators of CI may be helpful in improving patient outcomes. Furthermore, CI closely contributes to impaired exercise capacity18, which is the single most important variable to predict outcomes in patients with CHD. Although higher levels of PA are associated with enhanced exercise capacity and quality of life and reduced psychological stress in patients with CHD16,25, it remains unclear whether higher levels of PA are associated with a lower prevalence of CI in this subset. Additionally, no previous reports have delineated the minimal weekly PA dosage, below which there is an increased likelihood of CI in patients with CHD.
The novel finding of the present study was that higher levels of PA are associated with a lower prevalence of CI, independent of potential risk factors. Furthermore, the receiver operating characteristic curve to identify the PA level that best predicted CI was 15 METs-hours/week. Thus, PA levels <15 METs-hours/ week were associated with a higher prevalence of CI in patients with CHD. The present results are consistent with previous reports that exercise training reduced the likelihood of CI in patients with heart failure18-21, and extends these findings to patients with CHD. Therefore, beyond congenital cardiac anomalies, PA may be considered as a modifiable risk factor for CI in patients with CHD. To our knowledge, this is the first study to report that a higher level of PA was associated with a lower prevalence of CI in patients with CHD.
The prevalence of CI is reported at 16% to 22% in the general population4,26, whereas it is significantly higher in patients with CHD. Norozi et al.9 found a 34% prevalence of CI in adolescents and adults with CHD after cardiac surgery, whereas Diller et al.7 who investigated a diverse CHD population of varied ages, reported a 62% prevalence of CI. In the present study, we found an intermediate prevalence of CI, that is, 40%, which is higher than that of the general population. The varied prevalence rates of CI reported in previous studies may be due to differing assessment methodologies and/or different types of CHD and age ranges of the subjects.
The underlying mechanisms of CI in patients CHD remain unclear. According to some reports27,28, the density of beta-adrenergic receptors was reduced by 50% in the left ventricular myocardium due to myocardial damage following cardiac surgery. This attenuates the beta-adrenergic pathway and myocardial contractility, resulting in CI27,28. Other studies in patients with CHD have reported that CI was a result of alterations in autonomic nervous system stimulation or desensitization of synaptic beta-adrenergic receptors29 due to sinoatrial node damage during heart surgery10-12. These results are in accord with our findings relative to the relationship between CI and the number of previous surgeries. Clearly, additional studies are needed to further clarify the underlying mechanisms for CI in patients with CHD. In addition, it is a possible that CI in patients with CHD may results in low PA. Therefore, it is not clear whether higher PA levels produce reductions in CI over time, or whether decreased CI results in low PA. Further studies are needed to exam these associations.
Regular exercise increases heart rate variability due to enhanced resting baroreflex sensitivity and parasympathetic activity with corresponding decreases in catecholamines, angiotensin II, vasopressin, plasma B-type natriuretic peptide and sympathetic activity30. Collectively, these adaptations may serve to reduce heart rate at rest and during exercise while modestly increasing the maximal heart rate. This is supported by a meta-analysis in heart failure patients demonstrating that maximal heart rate increased by 4 beats/min following exercise training17. The present study confirmed that PA is independently associated with the prevalence of CI and that 15 METs-hours/week may be used to predict this anomaly. This level of PA corresponds to exercising for 40–60 minutes, at 3–4 METs, 5 days per week. Interestingly, a PA volume of 15 METs-hours/week is compatible with the recommended amount of PA for children and adolescents with CHD31.
The present study has several limitations. First, the results from our cross-sectional study design do not necessarily infer a causal relationship. Accordingly, additional studies are needed to confirm whether structured PA reduces the risk of CI, ideally via a randomized-controlled trial. Secondly, although the PA assessment methodology used in this study has been previously validated, the obtained self-reported data depended on the subjects’ subjective perceptions and recall. Therefore, there is a need to confirm the relationship between CI and PA via more objective measurement tools (e.g., accelerometer, personalized activity intelligence scores). Thirdly, although we classified PA into three categories (low, moderate, and high) based on tertiles of PA, these categories may be viewed as somewhat arbitrary. Finally, some prescribed medications may serve as an independent predictor for CI. However, we did not account for potential confounding medications such as beta-blockers and/or calcium channel blockers, which may have negatively impacted chronotropic reserve. It is an interesting to report that CI significantly improves with exercise training in patients with acute coronary syndrome, irrespective of beta-blockers dose changes32.
Our findings demonstrated that a higher level of PA was independently associated with a lower prevalence of CI, and that 15 METs-hours/week provided the most accurate cut-point for predicting the presence of this prognostic indicator in patients with complex CHD. These results suggest that increasing lifestyle PA, structured exercise, or both, should be considered as modifiable risk factors to reduce the risk of CI in adolescents with CHD, and potentially improve prognosis.
Notes
Author Contributions
Conceptualization: HJK, JKY, SYJ. Data curation, Formal analysis: HJK, JKY. Funding acquisition, Investigation, Methodology, Resources, Validation, Visualization, Software: HJK. Project administration: HJK, SYJ. Supervision: HJK, SYJ, CHK, SHK, BAF. Writing–Original Draft: HJK, JKY. Writing–Review & Editing: All authors.
REFERENCES
1. Hammond HK, Froelicher VF. 1985; Normal and abnormal heart rate responses to exercise. Prog Cardiovasc Dis. 27:271–96. DOI: 10.1016/0033-0620(85)90010-6. PMID: 2857054.


2. Arai Y, Saul JP, Albrecht P, et al. 1989; Modulation of cardiac autonomic activity during and immediately after exercise. Am J Physiol. 256(1 Pt 2):H132–41. DOI: 10.1152/ajpheart.1989.256.1.H132. PMID: 2643348.


3. Ellestad MH. 1996; Chronotropic incompetence: the implications of heart rate response to exercise (compensatory parasympathetic hyperactivity?). Circulation. 93:1485–7. DOI: 10.1161/01.CIR.93.8.1485. PMID: 8608613.
4. Lauer MS, Okin PM, Larson MG, Evans JC, Levy D. 1996; Impaired heart rate response to graded exercise: prognostic implications of chronotropic incompetence in the Framingham Heart Study. Circulation. 93:1520–6. DOI: 10.1161/01.CIR.93.8.1520. PMID: 8608620.
5. Martins S, Soares RM, Cotrim C, et al. 1999; The metabolic-chronotropic relation in patients with heart failure: a correlation with functional capacity. Rev Port Cardiol. 18:887–94. PMID: 10590653.
6. Clark AL, Coats AJ. 1995; Chronotropic incompetence in chronic heart failure. Int J Cardiol. 49:225–31. DOI: 10.1016/0167-5273(95)02316-O. PMID: 7649668.


7. Diller GP, Dimopoulos K, Okonko D, et al. 2006; Heart rate response during exercise predicts survival in adults with congenital heart disease. J Am Coll Cardiol. 48:1250–6. DOI: 10.1016/j.jacc.2006.05.051. PMID: 16979014.


8. Lui GK, Silversides CK, Khairy P, et al. 2011; Heart rate response during exercise and pregnancy outcome in women with congenital heart disease. Circulation. 123:242–8. DOI: 10.1161/CIRCULATIONAHA.110.953380. PMID: 21220738.


9. Norozi K, Wessel A, Alpers V, et al. 2007; Chronotropic incompetence in adolescents and adults with congenital heart disease after cardiac surgery. J Card Fail. 13:263–8. DOI: 10.1016/j.cardfail.2006.12.002. PMID: 17517345.


10. Ohuchi H, Arakaki Y, Yagihara T, Kamiya T. 1997; Cardiores-piratory responses to exercise after repair of the univentricular heart. Int J Cardiol. 58:17–30. DOI: 10.1016/S0167-5273(96)02848-3. PMID: 9021424.


11. Hannon JD, Danielson GK, Puga FJ, Heise CT, Driscoll DJ. 1985; Cardiorespiratory response to exercise after repair of tetralogy of Fallot. Tex Heart Inst J. 12:393–400. PMID: 15227002. PMCID: PMC341897.
12. Massin MM, Dessy H, Malekzadeh-Milani SG, Khaldi K, Topac B, Edelman R. 2009; Chronotropic impairment after surgical or percutaneous closure of atrial septal defect. Catheter Cardiovasc Interv. 73:564–7. DOI: 10.1002/ccd.21857. PMID: 19133677.


13. Thompson PD, Buchner D, Pina IL, et al. 2003; Exercise and physical activity in the prevention and treatment of atherosclerotic cardiovascular disease: a statement from the Council on Clinical Cardiology (Subcommittee on Exercise, Rehabilitation, and Prevention) and the Council on Nutrition, Physical Activity, and Metabolism (Subcommittee on Physical Activity). Circulation. 107:3109–16. DOI: 10.1161/01.CIR.0000075572.40158.77. PMID: 12821592.


14. Müller J, Christov F, Schreiber C, Hess J, Hager A. 2009; Exercise capacity, quality of life, and daily activity in the long-term follow-up of patients with univentricular heart and total cavopulmonary connection. Eur Heart J. 30:2915–20. DOI: 10.1093/eurheartj/ehp305. PMID: 19692392.


15. Buys R, Van De Bruaene A, Budts W, Delecluse C, Vanhees L. 2012; In adults with atrial switch operation for transposition of the great arteries low physical activity relates to reduced exercise capacity and decreased perceived physical functioning. Acta Cardiol. 67:49–57. DOI: 10.1080/AC.67.1.2146565. PMID: 22455089.


16. Rosenthal TM, Leung ST, Ahmad R, et al. 2016; Lifestyle modification for the prevention of morbidity and mortality in adult congenital heart disease. Congenit Heart Dis. 11:189–98. DOI: 10.1111/chd.12341. PMID: 26931766.


17. van Tol BA, Huijsmans RJ, Kroon DW, Schothorst M, Kwakkel G. 2006; Effects of exercise training on cardiac performance, exercise capacity and quality of life in patients with heart failure: a meta-analysis. Eur J Heart Fail. 8:841–50. DOI: 10.1016/j.ejheart.2006.02.013. PMID: 16713337.


18. Brubaker PH, Kitzman DW. 2011; Chronotropic incompetence: causes, consequences, and management. Circulation. 123:1010–20. DOI: 10.1161/CIRCULATIONAHA.110.940577. PMID: 21382903. PMCID: PMC3065291.
19. Miossi R, Benatti FB, Lúciade de Sá Pinto A, et al. 2012; Using exercise training to counterbalance chronotropic incompetence and delayed heart rate recovery in systemic lupus erythematosus: a randomized trial. Arthritis Care Res (Hoboken). 64:1159–66. DOI: 10.1002/acr.21678. PMID: 22438298.
20. Keteyian SJ, Brawner CA, Schairer JR, et al. 1999; Effects of exercise training on chronotropic incompetence in patients with heart failure. Am Heart J. 138(2 Pt 1):233–40. DOI: 10.1016/S0002-8703(99)70106-7. PMID: 10426833.


21. Brubaker PH, Kitzman DW. 2007; Prevalence and management of chronotropic incompetence in heart failure. Curr Cardiol Rep. 9:229–35. DOI: 10.1007/BF02938355. PMID: 17470336.


22. Keytsman C, Dendale P, Hansen D. 2015; Chronotropic incompetence during exercise in type 2 diabetes: aetiology, assessment methodology, prognostic impact and therapy. Sports Med. 45:985–95. DOI: 10.1007/s40279-015-0328-5. PMID: 25834997.


23. Wilkoff BL, Miller RE. 1992; Exercise testing for chronotropic assessment. Cardiol Clin. 10:705–17. DOI: 10.1016/S0733-8651(18)30211-X. PMID: 1423382.


24. Armstrong T, Bull F. 2006; Development of the World Health Organization Global Physical Activity Questionnaire (GPAQ). J Public Health. 14:66–70. DOI: 10.1007/s10389-006-0024-x.


25. Kovacs AH, Kaufman TM, Broberg CS. 2018; Cardiac rehabilitation for adults with congenital heart disease: physical and psychosocial considerations. Can J Cardiol. 34(10 Suppl 2):S270–7. DOI: 10.1016/j.cjca.2018.07.016. PMID: 30274637.


26. Jae SY, Fernhall B, Heffernan KS, et al. 2006; Chronotropic response to exercise testing is associated with carotid atherosclerosis in healthy middle-aged men. Eur Heart J. 27:954–9. DOI: 10.1093/eurheartj/ehi832. PMID: 16537555.


27. Bristow MR, Hershberger RE, Port JD, et al. 1990; Beta-adrenergic pathways in nonfailing and failing human ventricular myocardium. Circulation. 82(2 Suppl):I12–25. PMID: 2164894.
28. Colucci WS, Ribeiro JP, Rocco MB, et al. 1989; Impaired chronotropic response to exercise in patients with congestive heart failure: role of postsynaptic beta-adrenergic desensitization. Circulation. 80:314–23. DOI: 10.1161/01.CIR.80.2.314. PMID: 2546698.


29. Davos CH, Davlouros PA, Wensel R, et al. 2002; Global impairment of cardiac autonomic nervous activity late after repair of tetralogy of Fallot. Circulation. 106(12 Suppl 1):I69–75. DOI: 10.1161/01.cir.0000032886.55215.15. PMID: 12354712.


30. Gademan MG, Swenne CA, Verwey HF, et al. 2007; Effect of exercise training on autonomic derangement and neurohumoral activation in chronic heart failure. J Card Fail. 13:294–303. DOI: 10.1016/j.cardfail.2006.12.006. PMID: 17517350.


31. Takken T, Giardini A, Reybrouck T, et al. 2012; Recommendations for physical activity, recreation sport, and exercise training in paediatric patients with congenital heart disease: a report from the Exercise, Basic & Translational Research Section of the European Association of Cardiovascular Prevention and Rehabilitation, the European Congenital Heart and Lung Exercise Group, and the Association for European Paediatric Cardiology. Eur J Prev Cardiol. 19:1034–65. DOI: 10.1177/1741826711420000. PMID: 23126001.


32. Pimenta T, Rocha JA. 2021; Cardiac rehabilitation and improvement of chronotropic incompetence: is it the exercise or just the beta blockers? Rev Port Cardiol (Engl Ed). 40:947–53. DOI: 10.1016/j.repc.2021.01.014. PMID: 34922702.


Fig. 1
The receiver operating characteristic curve to identify the optimal physical activity level for the prediction of confidence interval. MET, metabolic equivalent.
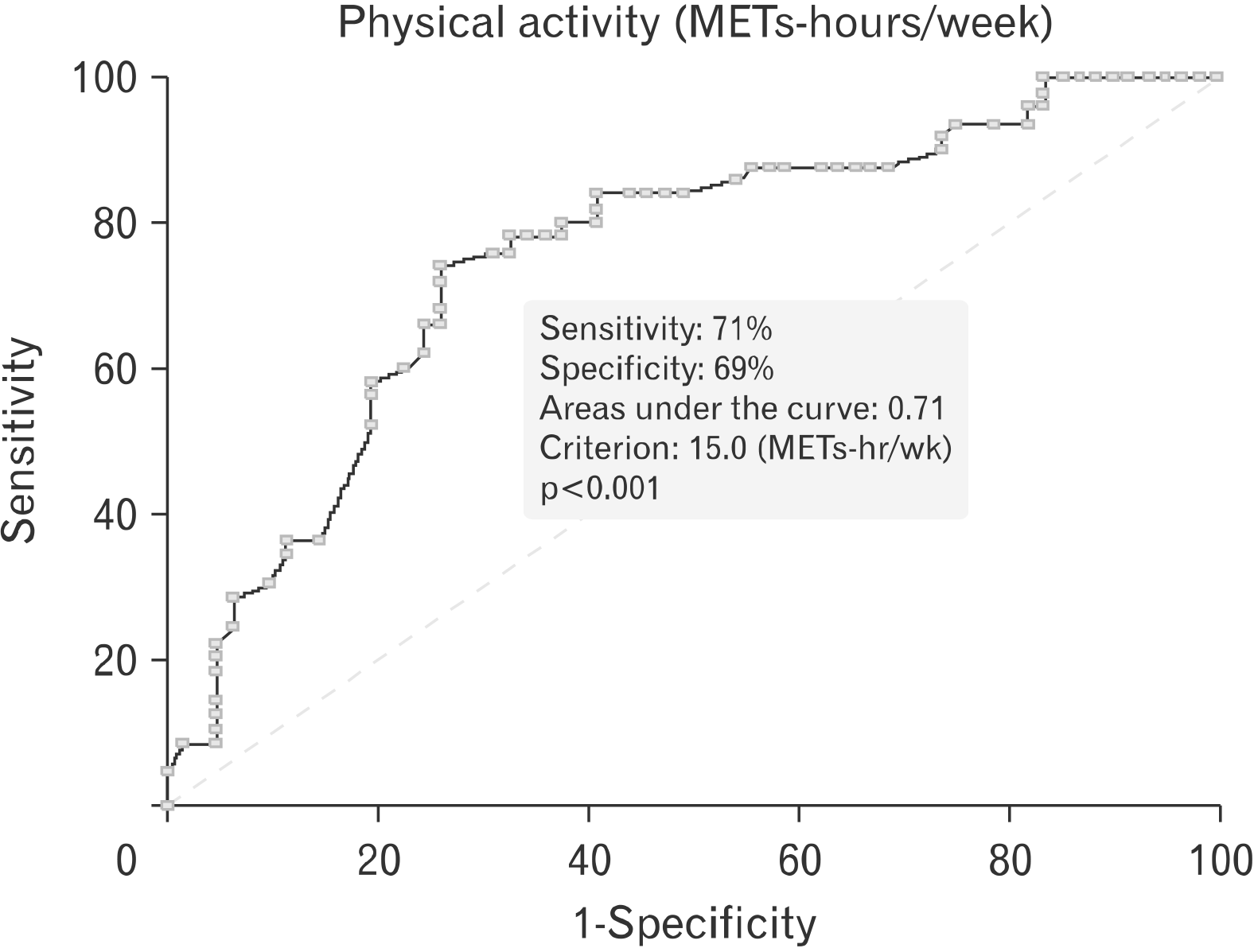
Table 1
Characteristics of patients (n=111)
Characteristic | Data |
---|---|
Age (yr) | 16.2±1.8 |
Sex | |
Male | 61 (55.0) |
Female | 50 (45.0) |
Body mass index (kg/m2) | 20.6±3.5 |
No. of surgical procedures | |
0 | 1 (0.9) |
1−2 | 60 (54.1) |
>3 | 50 (45.0) |
Cyanosis | 19 (17.1) |
Birth weight (kg) | 3.00±0.54 |
No. of medications | |
0 | 41 (36.9) |
1−2 | 49 (44.1) |
>3 | 21 (18.9) |
Diseases | |
Pulmonary stenosis | 3 (2.7) |
Aortic stenosis | 2 (1.8) |
Atrioventricular septal defect | 2 (1.8) |
Coarctation of the aorta | 3 (2.7) |
Double outlet right ventricle | 6 (5.4) |
Fontan circulation | 36 (32.4) |
Pulmonary atresia with ventricular septal defect | 14 (12.6) |
Transposition of great arteries | 6 (5.4) |
Tetralogy of Fallot | 34 (30.6) |
Others* | 5 (4.5) |
Table 2
Characteristics of patients with and without chronotropic incompetence (CI)
Table 3
The ORs and 95% CIs for the prevalence of chronotropic incompetence by physical activity tertiles
Physical activity | Unadjusted OR (95% CI) | Adjusted* OR (95% CI) |
---|---|---|
Low (n=38) | 1 (Reference) | 1 (Reference) |
Moderate (n=38) | 0.53 (0.21−1.31) | 0.86 (0.28−2.65) |
High (n=35) | 0.18 (0.06−0.51) | 0.25 (0.06−0.99) |
Table 4
Multivariate logistic regression for chronotropic incompetence