Abstract
Background/Aims
Angiogenesis is essential for the outgrowth and metastasis of tumors. The structure and characteristics of tumor vasculature differ from those of normal vessels. We compared the characteristics of differentially expressed genes in endothelial cells (ECs) isolated from gastric and normal cells.
Methods
Previously, we had isolated pure tumor ECs (TECs) and normal ECs (NECs) from advanced gastric cancer (AGC) lesions and normal mucosal tissues, respectively. Using the oligomer chip platform of the Affymetrix GeneChip technology, genes that were expressed more than three-fold with a significance of p≤0.001 were measured. The intercellular adhesion molecule 1 (ICAM-1) was found to be overexpressed in the TECs compared to the normal gastric ECs. In this study, the upregulation of ICAM-1 was confirmed in cultured TECs by immunofluorescence.
Results
The expression of ICAM-1 was upregulated in the ECs, as well as in the stromal and immune cells, in early human gastric preneoplastic and hepatic fibrotic tissues. Upregulation of ICAM-1 was observed in the TECs, immune cells, and cancer epithelial cells in AGC and hepatocellular carcinoma (HCC). These results suggest that increased ICAM-1 expression in the ECs of the tissue microenvironment progressively contributes to the recruitment of immune cells to promote inflammation, leading to fibrosis and tumorigenesis.
Tumor angiogenesis is a pathological process characterized by vessels with aberrant architecture and properties. Tumor vasculature is distinct from normal vasculature structurally and functionally in that it is leaky, tortuous, and chaotic. In addition, from a genetic standpoint, these vessels have specific genes and surface proteins called tumor endothelial cell (EC) markers, suggestive of the genetic background shared with the tumors that surround them.1,2 Cancer cells and the surrounding stromal and inflammatory cells interact with one another to form an inflammatory tumor microenvironment (TME). The inflammatory response plays a key role in the development of cancer and promotes all the stages of tumorigenesis.3
Gastric cancer (GC) is the fifth most frequent malignancy worldwide and the third most common cause of death, wherein the rate of median survival is less than one year in the advanced stage.4 Three-quarters of patients with GC are Asian and this is the most prevalent malignant neoplasm among Koreans.5 The aggressiveness of GC has been linked to many genetic factors, such as mutations in various oncogenes and tumor suppressor genes. The aberrant expression of these genes and their downstream targets can confer a phenotype of increased angiogenesis and metastasis.6 Gastric tumors exhibit various angiogenic phenotypes, and highly vascularized GC has a poorer prognosis than less vascularized cancers.7,8 Angiogenesis and the destruction of vascular architecture are also associated with the progression of chronic hepatic diseases, such as cirrhosis and hepatocellular carcinoma (HCC). Inflammation is a recurrent factor in the pathophysiology of both GC and chronic hepatic diseases. Proinflammatory mediators can elicit angiogenic responses by activating angiogenic factors, such as macrophages, mast cells, and activated leukocytesA.6,9
The intercellular adhesion molecule 1 (ICAM-1) is a cell-surface glycoprotein and is identified as a regulator of leukocyte recruitment from circulation to the sites of inflammation. It is mainly expressed in the vascular ECs, but its expression is highly induced in epithelial and immune cells in response to inflammatory stimulation.10 As ICAM-1 plays a central role in leukocyte trafficking, lymphocyte activation, and numerous immune responses, the upregulation of ICAM-1 is a signature event during inflammation, particularly in the endothelium.11 ICAM-1 expression has been positively correlated with various stages of gastric or hepatic cancers12 but negatively correlated with GC cells and patient prognosis.13 Through DNA microarray analysis we previously found that ICAM-1 is upregulated by isolated human GC ECs.2 However, whether ICAM-1 is overexpressed in tumor blood vessel ECs and premalignant tissues is largely unknown depending on the progression of the GC and HCC. Here, we found that the ICAM-1 expression pattern is highly upregulated in isolated human GC TECs compared with NECs. In addition, the ICAM-1 expression pattern in ECs and tissues was observed in human premalignant lesions and GC (early and advanced), hepatic cirrhosis, and HCC.
We obtained fresh specimens of human GC tissue and adjacent normal mucosa from nine patients who were treated at the Seoul National University Hospital, South Korea. The university pathology laboratory determined that the primary gastric carcinomas in these patients were either Stage III (TEC1) or Stage IIb (TEC2) of the poorly differentiated type. This study was approved by the Institutional Review Board (IRB) of Seoul National University Hospital (IRB No. C-0602-069-168), and informed consent was obtained from each subject.
NECs and gastric carcinoma TECs were isolated from their respective tissues using the Dynabeads magnetic bead system (Dynal Biotech, Inc., Lake Success, NY, USA). Fresh GC tissues were obtained within 30 minutes of surgical resection. For the normal tissue samples, gastric mucosa was taken exclusively from regions 10 cm away from tumors with clearly defined margins. The isolation protocols were carried out as previously described with slight modifications.14 Isolation of EC from patient tissues was performed as previously described.2 ECs were subsequently cultured onto gelatin-coated dishes with EC growth media-2 for microvessel EGM™-2MV (Clonetics™, Lonza Walkersville, Inc., Walkersville, MD, USA) supplemented with EGM™-2 SingleQuots™ (containing human vascular endothelial growth factor [hVEGF], human epidermal growth factor [hEGF], human fibroblast growth factor, insulin-like growth factor- 1, ascorbic acid, gentamycin, and heparin, and 20% fetal bovine serum (Hyclone Laboratories, Inc., Logan, UT, USA) containing penicillin, streptomycin, and fungizone (PSF; Gibco, Thermofischer Scientific, Waltham, MA, USA). This nutrient-rich culture media will be referred to hereafter as EBM-2MV. The cells were maintained in a humidified incubator at 37℃ and 5% CO2.
After ECs from GC or normal tissues were cultured, ECs were fixed with 4% paraformaldehyde, and incubated at 4℃ overnight with primary antibodies, ICAM-1 (sc-8439, 1:50, Santa Cruz Biotechnology, Dallas, TX, USA), von Willebrand factor (vWF; A0082, 1:50, DAKO) or CD31 (sc-1506, 1:50, Santa Cruz Biotechnology). The next day, the cells were washed several times and further incubated with secondary antibodies with specific fluorescent probes and visualized using a confocal microscope (Leica TCS SP5 II Dichroic/CS, Leica Microsystems, Wetzlar, Germany).
GC, HCC, and other cancer specimens in the human tumor tissue array blocks were immunostained. Normal mucosa, liver cirrhotic tissues, and other cancer tissues were included in the same block to serve as the control (SuperBioChips, Seoul, Korea). The tissue sections were deparaffinized and then dehydrated with xylene and ethanol. The anti-ICAM-1 antibody (Santa Cruz Biotechnology) was diluted at 1:50 and was used for immunostaining in conjunction with a Vector ABC kit (Vector Laboratories, Inc., Burlingame, CA, USA). The immunostained tissues were observed using a light microscope at ×200 magnification (Axio Imager A1, Carl Zeiss, Oberkochen, Germany). Images were taken of clearly stained lesions from tumoral and normal tissues. The ICAM-1–positive ECs in each image were counted. The data were expressed as the mean±SD of at least three fields. p-values <0.01 were considered statistically significant. Data were analyzed using a one-way analysis of variance.
After we isolated ECs from the NEC and TEC, we cultured the ECs on gelatin-coated dishes and performed an immunofluorescent (IF) assay with EC markers, vWF, and CD31 (Fig. 1A). We found both NECs and TECs expressed vWF and CD31, suggesting that the isolated ECs were homogeneous with high purity. When we compared the gene expression patterns of vascular NECs and TECs, ICAM-1 was increased 26-fold in the TECs, as determined using the Affymetrix GeneChip technology, whole human genome oligomer chips analysis.2 To confirm this result, the ICAM-1 protein expression was examined, which showed that the ICAM-1 protein level was highly increased in the TECs (Fig. 1B).
Next, we evaluated the ICAM-1 expression in the human stomach and liver tissues during disease progression by immunohistochemistry. ICAM-1 was expressed only in normal stomach vascular ECs (Fig. 2A). The expression of ICAM-1 in vascular ECs progressively increased through various stages of the disease from intestinal metaplasia and adenoma to early GC (Fig. 2B-D). In addition, ICAM-1 expression was also observed in recruited inflammatory cells and stromal cells. Compared with the ICAM-1 expression pattern in normal stomach vascular ECs, the ICAM-1 expression patterns in the stromal cells and recruited inflammatory cells of intestinal metaplasia, adenoma, and early GC were membranous and cytoplasmic. Moreover, ICAM-1 was preferentially expressed around regions of the intestinal metaplasia, adenoma, and the tumor compared with the non-lesion areas (Fig. 2B-D).
In the liver, ICAM-1 was expressed in sinusoidal ECs and the fibrotic matrix interstitial region in cirrhosis (Fig. 2F, G). In cirrhotic livers, increased ICAM-1 expression was observed in recruited inflammatory cells, stromal cells, and ECs, mainly in the cirrhotic area. Both cytoplasmic and membranous ICAM-1 expressions were observed in recruited inflammatory cells and stromal cells in the cirrhotic liver (Fig. 2G), in contrast to the membranous ICAM-1 expression pattern in the vascular endothelium of a normal liver. The ICAM-1 expression pattern in ECs was membranous; however, in the monocyte and matrix regions of the stomach and liver tissues the expression patterns were cytosolic and membranous (Fig. 2B-D, G). These data suggest that the ICAM-1 expression was upregulated, and the expression pattern was changed during the disease progression in the stomach and liver tissues.
Furthermore, we assessed the ICAM-1 expression in various human advanced cancer tissues, and classified them as vascularized or non-vascularized, based on the number of ECs in the tissues. In vascularized tumors, such as advanced gastric GC and HCC,15 increased ICAM-1 expression was observed in the recruited inflammatory cells and stromal cells near the tumor cells, as well as the TECs (Fig. 3).
As a relatively non-vascularized tumor, osteosarcoma has a few stromal and inflammatory cells,16 where scant expression of ICAM-1 was observed (Fig. 4A). Lymphoma is a hematologic malignancy that typically lacks both stromal and vascular components. Nevertheless, expression of ICAM-1 in tumor cells (Fig. 4B) is frequently found in hematologic tumor cells.17 Gastrointestinal stromal tumors showed a few ICAM-1– positive inflammatory cells, and ICAM-1 expression was cytoplasmic and membranous (Fig. 4C). In non-vascularized invasive ductal breast cancer, stromal fibrosis surrounding the infiltrating cancer cells was prominent. Membranous and cytoplasmic ICAM-1 expressions were observed in the stromal and inflammatory cells in the fibrotic areas, but tumor vasculature was not observed (Fig. 4D).
Here, we demonstrated that ICAM-1 was upregulated in TECs. Upregulation of ICAM-1 progressively increased with the pathogenesis of the disease in gastric and hepatic cancers from fibrosis to tumorigenesis. ICAM-1 expression was also observed in cancer cells, recruited inflammatory cells, and stromal cells, as well as vascular ECs. Thus, high ICAM-1 expression in these cells may contribute to tumorigenesis by changing nonfibrotic, non-vascularized stroma into a fibrotic and richly vascularized TME.
ICAM-1 is an adhesion molecule that regulates leukocyte recruitment from the blood to the sites of inflammation. It is a cell-surface glycoprotein expressed at a low basal level in immune, endothelial, and epithelial cells but is upregulated in response to inflammatory stimulation.18 Inflammation triggers various pathological conditions, and its continuous or sporadic events lead to chronic diseases, such as fibrosis and cancer.3,18,19 Increased ICAM-1 expression in vascular vessels induce the increased recruitment of immune cells via transendothelial migration. In turn, recruited immune cells secrete various cytokines, such as tumor necrosis factor-alpha (TNF-α) or interleukin-1 beta (IL-1β), to increase inflammatory responses and ICAM-1 expression.
Here, we demonstrated that the TECs express ICAM-1 more than the NECs (Fig. 1). Tumor vasculatures have typical genotypes and abnormal characteristics, such as leakiness. These abnormal properties may contribute to disease aggressiveness as well as resistance to chemotherapeutics.20,21 Increased ICAM-1 expression in the TECs may be induced by inflammatory cytokines secreted from immune cells in the TME. Tumor epithelial cells also upregulate ICAM-1 (Fig. 3) in the same way as the TECs. ICAM-1 expression in tumor cells and TECs would sustain and potentiate leukocyte trafficking and its effector function in the TME, leading to tumor growth.
We also found that ICAM-1 expression in the ECs or stromal cells increased during the course of the pathogenesis of fibrosis or the initiation stage of preneoplasia (Fig. 2). Recent reports suggest that ICAM-1 regulates the clearance of apoptotic or dead cells and efferocytosis of macrophages.22 Efferocytosis induces the resolution of the inflammatory response via the production of pro-resolution cytokines, IL-10, transforming growth factor-beta (TGF-β), and prostaglandin E2 (PGE2).23 The reports suggest that ICAM-1 induces healing of tissue injury and facilitates the resolution of inflammation. This function of ICAM-1 may be due to the promotion of neovascularization.24 The pathogenesis of fibrosis and tumorigenesis are the results of repetitive inflammation and wound repair.3,10
Our study suggests that progressive increased ICAM-1 expression in the ECs, stromal cells, and immune cells in the tissue microenvironment contributes to the recruitment of immune cells to promote inflammation and subsequently induces wound healing, leading to fibrosis and tumorigenesis. Therefore, upregulated ICAM-1 in vascular ECs in inflammatory diseases, including cancers or fibrosis, could be a promising target for prevention or treatment.
Notes
REFERENCES
1. Hida K, Hida Y, Amin DN, et al. 2004; Tumor-associated endothelial cells with cytogenetic abnormalities. Cancer Res. 64:8249–8255. DOI: 10.1158/0008-5472.CAN-04-1567. PMID: 15548691.


2. Kim WH, Lee SH, Jung MH, et al. 2009; Neuropilin2 expressed in gastric cancer endothelial cells increases the proliferation and migration of endothelial cells in response to VEGF. Exp Cell Res. 315:2154–2164. DOI: 10.1016/j.yexcr.2009.04.018. PMID: 19409892.


3. Greten FR, Grivennikov SI. 2019; Inflammation and cancer: triggers, mechanisms, and consequences. Immunity. 51:27–41. DOI: 10.1016/j.immuni.2019.06.025. PMID: 31315034. PMCID: PMC6831096.


4. Machlowska J, Baj J, Sitarz M, Maciejewski R, Sitarz R. 2020; Gastric cancer: epidemiology, risk factors, classification, genomic characteristics and treatment strategies. Int J Mol Sci. 21:4012. DOI: 10.3390/ijms21114012. PMID: 32512697. PMCID: PMC7312039.


5. Rawla P, Barsouk A. 2019; Epidemiology of gastric cancer: global trends, risk factors and prevention. Prz Gastroenterol. 14:26–38. DOI: 10.5114/pg.2018.80001. PMID: 30944675. PMCID: PMC6444111.


6. Hsieh HL, Tsai MM. 2019; Tumor progression-dependent angiogenesis in gastric cancer and its potential application. World J Gastrointest Oncol. 11:686–704. DOI: 10.4251/wjgo.v11.i9.686. PMID: 31558974. PMCID: PMC6755109.


7. Chen CN, Cheng YM, Lin MT, Hsieh FJ, Lee PH, Chang KJ. 2002; Association of color Doppler vascularity index and microvessel density with survival in patients with gastric cancer. Ann Surg. 235:512–518. DOI: 10.1097/00000658-200204000-00009. PMID: 11923607. PMCID: PMC1422466.


8. Gong W, Wang L, Yao JC, et al. 2005; Expression of activated signal transducer and activator of transcription 3 predicts expression of vascular endothelial growth factor in and angiogenic phenotype of human gastric cancer. Clin Cancer Res. 11:1386–1393. DOI: 10.1158/1078-0432.CCR-04-0487. PMID: 15746037.


9. Coulon S, Heindryckx F, Geerts A, Van Steenkiste C, Colle I, Van Vlierberghe H. 2011; Angiogenesis in chronic liver disease and its complications. Liver Int. 31:146–162. DOI: 10.1111/j.1478-3231.2010.02369.x. PMID: 21073649.


10. Bui TM, Wiesolek HL, Sumagin R. 2020; ICAM-1: a master regulator of cellular responses in inflammation, injury resolution, and tumorigenesis. J Leukoc Biol. 108:787–799. DOI: 10.1002/JLB.2MR0220-549R. PMID: 32182390. PMCID: PMC7977775.


11. Ramos TN, Bullard DC, Barnum SR. 2014; ICAM-1: isoforms and phenotypes. J Immunol. 192:4469–4474. DOI: 10.4049/jimmunol.1400135. PMID: 24795464. PMCID: PMC4015451.


12. Maruo Y, Gochi A, Kaihara A, et al. 2002; ICAM-1 expression and the soluble ICAM-1 level for evaluating the metastatic potential of gastric cancer. Int J Cancer. 100:486–490. DOI: 10.1002/ijc.10514. PMID: 12115535.


13. Fujihara T, Yashiro M, Inoue T, et al. 1999; Decrease in ICAM-1 expression on gastric cancer cells is correlated with lymph node metastasis. Gastric Cancer. 2:221–225. DOI: 10.1007/s101200050067. PMID: 11957102.


14. St Croix B, Rago C, Velculescu V, et al. 2000; Genes expressed in human tumor endothelium. Science. 289:1197–1202. DOI: 10.1126/science.289.5482.1197. PMID: 10947988.


15. Albini A, Tosetti F, Li VW, Noonan DM, Li WW. 2012; Cancer prevention by targeting angiogenesis. Nat Rev Clin Oncol. 9:498–509. DOI: 10.1038/nrclinonc.2012.120. PMID: 22850752.


16. Kim HS, Lim SJ, Park YK. 2009; Anti-angiogenic factor endostatin in osteosarcoma. APMIS. 117:716–723. DOI: 10.1111/j.1600-0463.2009.02524.x. PMID: 19775339.


17. Terol MJ, Tormo M, Martinez-Climent JA, et al. 2003; Soluble intercellular adhesion molecule-1 (s-ICAM-1/s-CD54) in diffuse large B-cell lymphoma: association with clinical characteristics and outcome. Ann Oncol. 14:467–474. DOI: 10.1093/annonc/mdg057. PMID: 12598355.


18. Hubbard AK, Rothlein R. 2000; Intercellular adhesion molecule-1 (ICAM-1) expression and cell signaling cascades. Free Radic Biol Med. 28:1379–1386. DOI: 10.1016/S0891-5849(00)00223-9.


19. Jeong JH, Ojha U, Lee YM. 2021; Pathological angiogenesis and inflammation in tissues. Arch Pharm Res. 44:1–15. DOI: 10.1007/s12272-020-01287-2. PMID: 33230600. PMCID: PMC7682773.


20. Azzi S, Hebda JK, Gavard J. 2013; Vascular permeability and drug delivery in cancers. Front Oncol. 3:211. DOI: 10.3389/fonc.2013.00211. PMID: 23967403. PMCID: PMC3744053.


21. Jain RK. 2005; Normalization of tumor vasculature: an emerging concept in antiangiogenic therapy. Science. 307:58–62. DOI: 10.1126/science.1104819. PMID: 15637262.


22. Wiesolek HL, Bui TM, Lee JJ, et al. 2020; Intercellular adhesion molecule 1 functions as an efferocytosis receptor in inflammatory macrophages. Am J Pathol. 190:874–885. DOI: 10.1016/j.ajpath.2019.12.006. PMID: 32035057. PMCID: PMC7180595.


23. Elliott MR, Koster KM, Murphy PS. 2017; Efferocytosis signaling in the regulation of macrophage inflammatory responses. J Immunol. 198:1387–1394. DOI: 10.4049/jimmunol.1601520. PMID: 28167649. PMCID: PMC5301545.


24. Gho YS, Kleinman HK, Sosne G. 1999; Angiogenic activity of human soluble intercellular adhesion molecule-1. Cancer Res. 59:5128–5132.
Fig. 1
Intercellular adhesion molecule 1 (ICAM-1) expression in isolated human gastric tumor endothelial cells. (A) Expression of von Willebrand factor (vWF) and a cluster of differentiation 31 (CD31) was identified in both cultured endothelial cells from a gastric tumor (TEC) and those from normal tissues (NEC) using an immunofluorescent (IF) assay. Immunoglobulin (IgG) antibodies were used for IF control. (B) ICAM-1 expression was examined in both cultured TECs and NECs by IF assay.
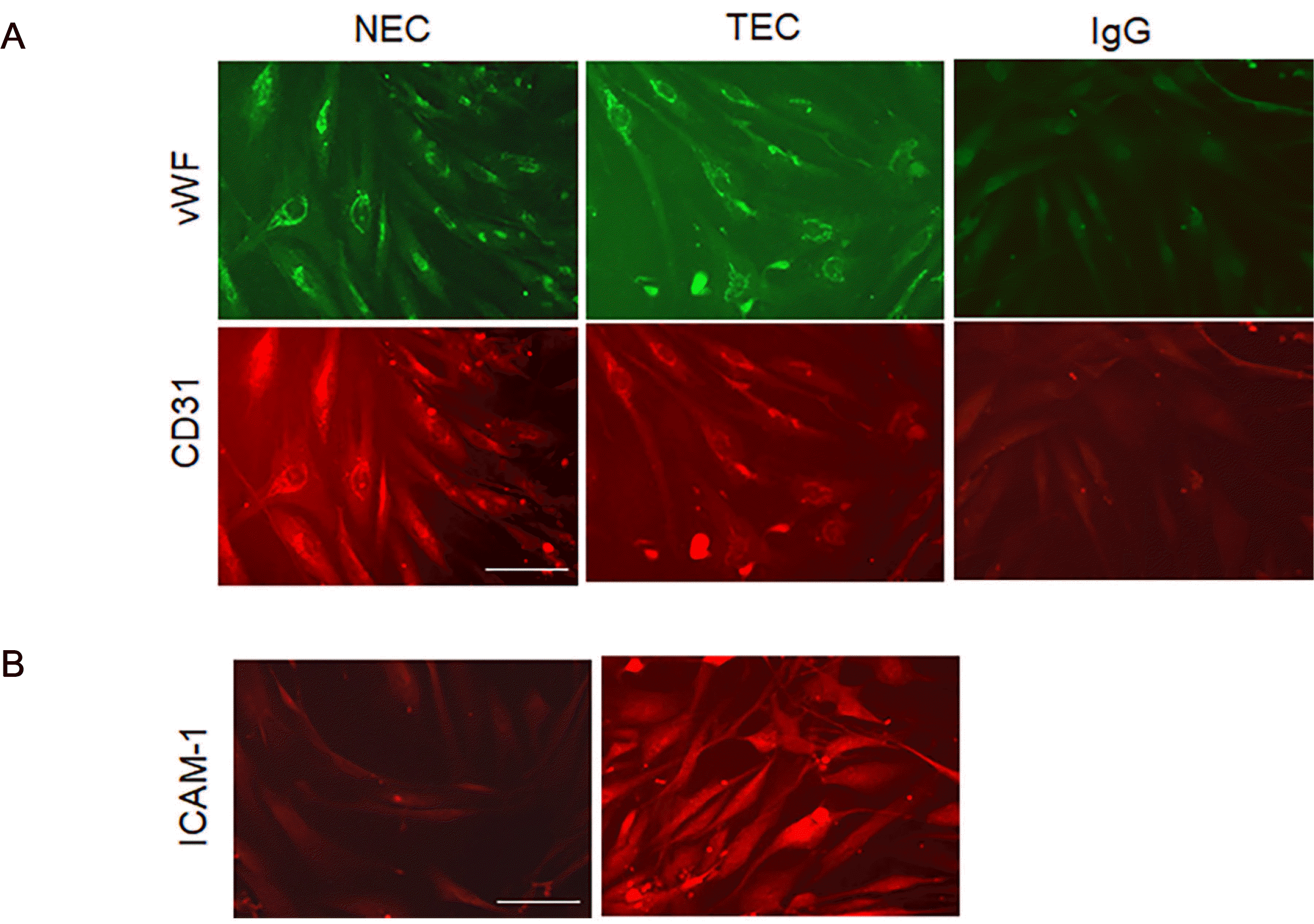
Fig. 2
ICAM-1 expression in gastric intestinal metaplasia, gastric adenoma, early gastric cancer, and fibrotic liver tissues. Human gastric tissue array slides were used for immunohistochemical analysis with anti-ICAM-1 antibodies. (A) Normal stomach, (B) intestinal metaplasia, (C) gastric adenoma, and (D) early gastric cancer. The numbers of ICAM-1–positive ECs were counted in at least three different sampling fields under a light microscope. (E) A graph of the cell counts. Data are expressed as the mean±standard deviation of three different fields. ap<0.01 between groups. (B) Human hepatic tissue array slides of normal and cirrhotic livers were used for immunohistochemical analysis with anti-ICAM-1 antibodies. (F) Normal liver and (G) cirrhotic liver. The numbers of ICAM-1–positive ECs were counted in at least three different sampling fields under the microscope. (H) A graph of the cell counts. Photographs were taken at ×200 magnification. Immunohistochemistry of every tissue samples was performed with 3,3'-diaminobenzidine tetrahydrochloride salt staining methods. Data are expressed as the mean±standard deviation of three different fields. ap<0.01 between groups.
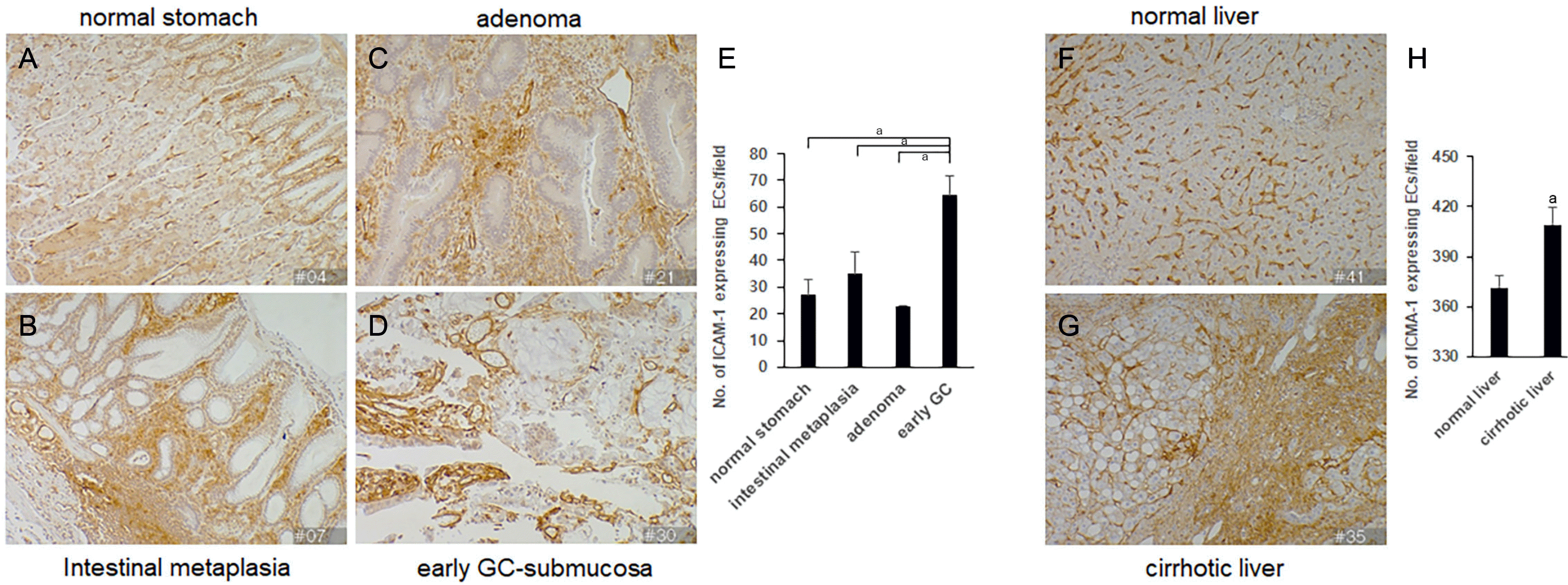
Fig. 3
ICAM-1 expression in human advanced gastric cancer and hepatocellular carcinoma. (A) Human gastric cancer tissue array slides were used for immunohistochemical analysis with anti-ICAM-1 antibodies. (B) Human hepatic cancer tissue array slides were used for immunohistochemical analysis with anti-ICAM-1 antibodies. Photographs were taken at ×200 magnification. Immunohistochemistry of every tissue samples was performed with 3,3'-diaminobenzidine tetrahydrochloride salt staining methods.
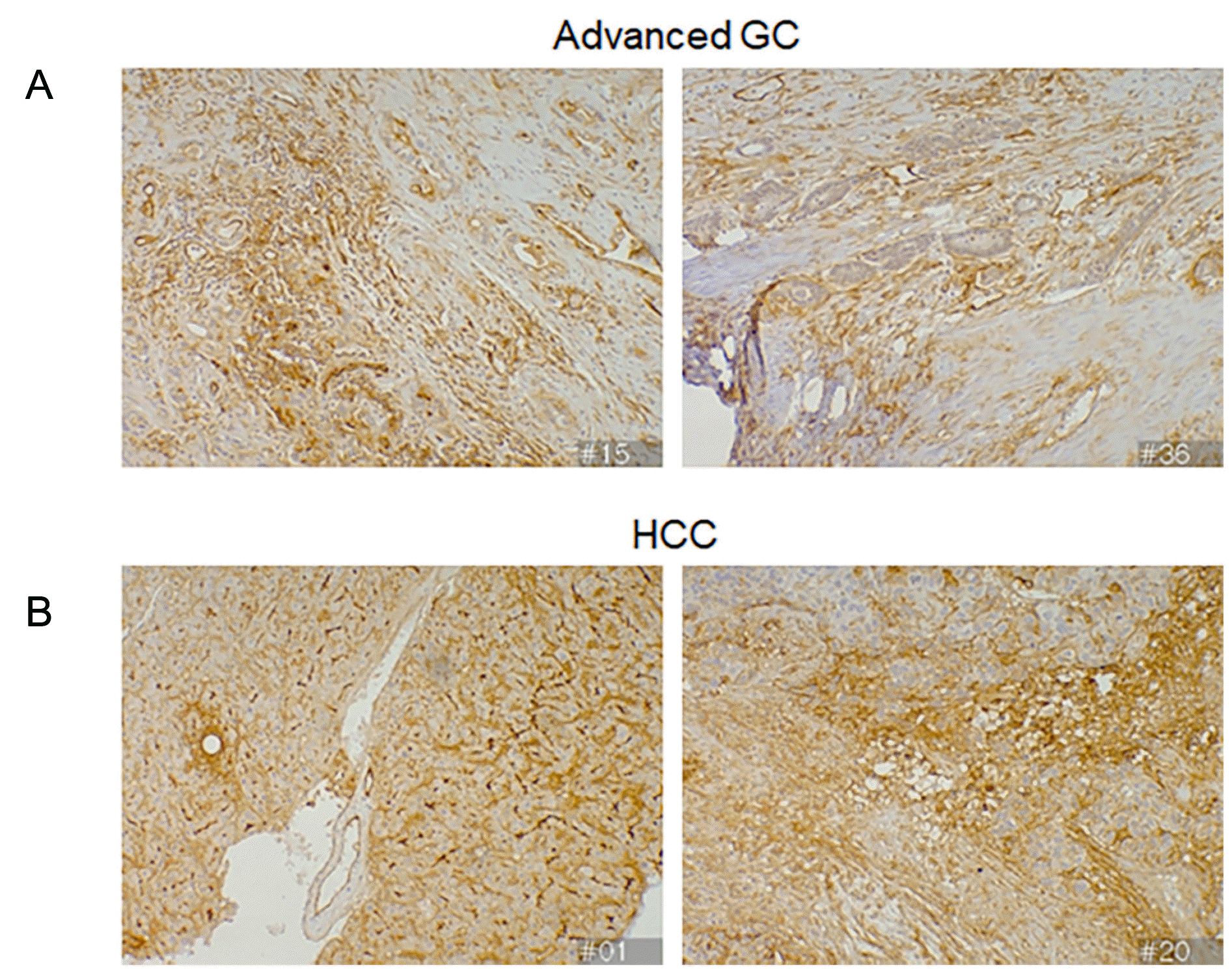
Fig. 4
ICAM-1 expression in various human cancers. An array slide of various human cancer tissues was used with anti-ICAM-1 antibodies. (A) Osteosarcoma, (B) lymphoma (diffuse large B cell lymphoma), (C) gastrointestinal stromal tumor, and (D) breast cancer. Photographs were taken at ×200 magnification. Immunohistochemistry of every tissue samples was performed with 3,3'-diaminobenzidine tetrahydrochloride salt staining methods.
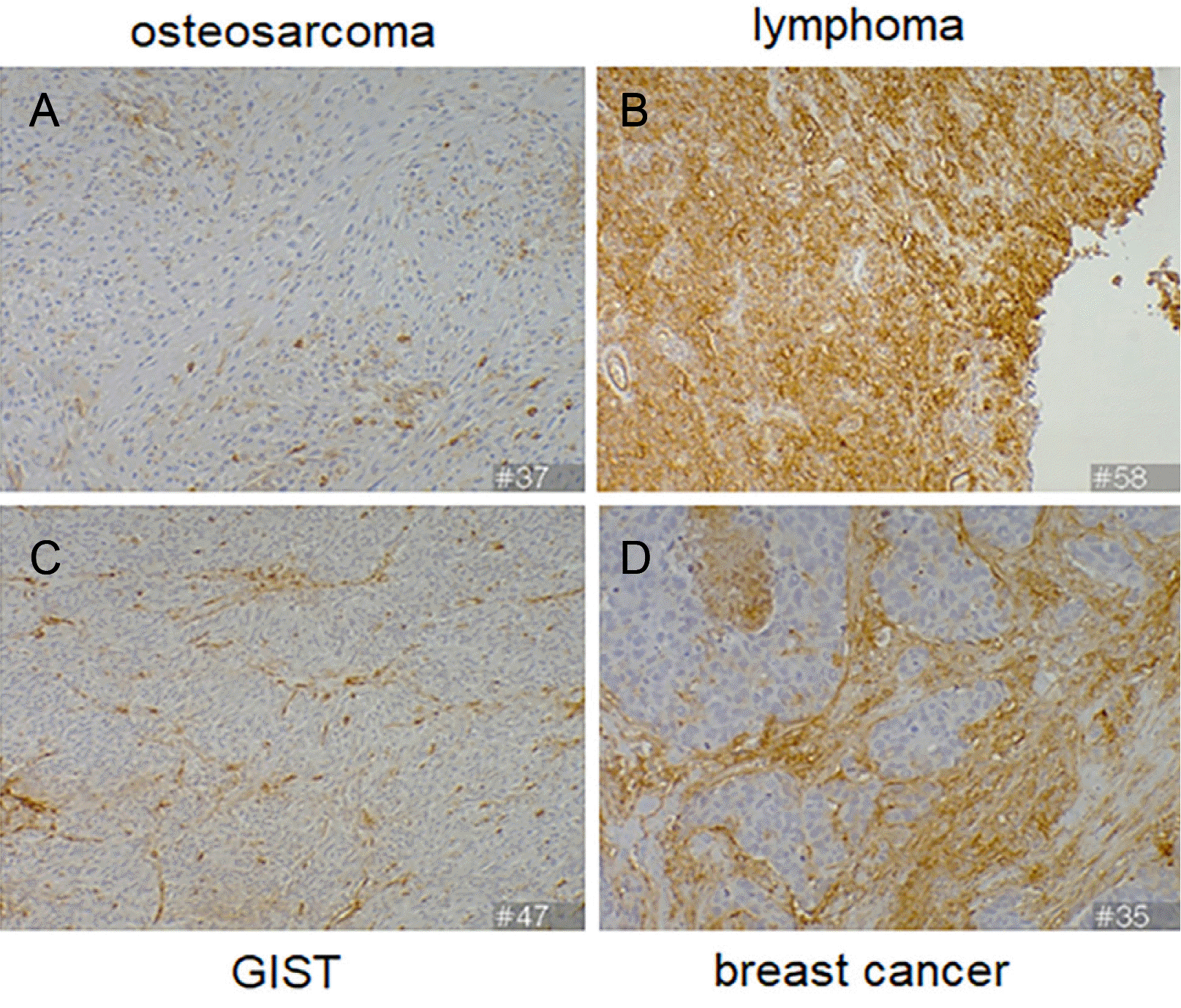