Abstract
Disulfiram (DSF) is an aldehyde dehydrogenase inhibitor. DSF has potent anti-cancer activity for solid and hematological malignancies. Although the effects on cancer cells have been proven, there have been few studies on DSF toxicity in bone marrow cells (BMs). DSF reduces the metabolic activity and the mitochondrial membrane potential of BMs. In subset analyses, we confirmed that DSF does not affect the proportion of BMs. In addition, DSF significantly impaired the metabolic activity and differentiation of BMs treated with granulocyte macrophage-colony stimulating factor, an essential growth and differentiation factor for BMs. To measure DSF toxicity in BMs in vivo, mice were injected with 50 mg/kg, a dose used for anti-cancer effects. DSF did not significantly induce BM toxicity in mice and may be tolerated by antioxidant defense mechanisms. This is the first study on the effects of DSF on BMs in vitro and in vivo. DSF has been widely studied as an anti-cancer drug candidate, and many anti-cancer drugs lead to myelosuppression. In this regard, this study can provide useful information to basic science and clinical researchers.
Disulfiram (DSF) has been used as a major therapeutic agent of alcohol dependence by inhibiting aldehyde dehydrogenase (ALDH) [1,2]. In the liver, alcohol is metabolized to acetaldehyde and can then be converted to acetic acid by ALDH. DSF blocks the activity of ALDH, resulting in the accumulation of acetaldehyde [3]. This causes serious hangover symptoms such as flushing of the skin, accelerated heart rate, shortness of breath, nausea, vomiting, and throbbing headache. DSF is a potent anti-cancer agent against various cancers [4]. DSF blocks the activity of ALDH, a marker of cancer stem cells, and inhibits proteasome activity by forming complexes with metal ions [5].
Most anti-cancer agents generate serious side effects including vomiting, hair loss, myelosuppression, and hypertension. In particular, myelosuppression is a life-threatening side effect in patients treated with anti-cancer agents [6], as bone marrow cells (BMs) provide hematopoietic and immune cells. Although there are many studies regarding the anti-cancer effects of DSF, useful information on the effects of DSF on BMs is lacking.
In this study, we determined whether DSF may influence the viability and function of BMs and the underlying mechanisms. To determine the toxicity of DSF in BMs, we measured the metabolic activity, mitochondrial function, and subset ratio of DSF-treated BMs. We investigated the effects of DSF on BMs treated with granulocyte-macrophage colony-stimulating factor (GM-CSF), an essential growth and differentiation factor for BMs. In addition, the BM toxicity of the DSF dosage used for anti-cancer effect was evaluated in vivo, based on the cellularity and subset analyses of BMs.
C57BL/6 mice were purchased from ORIENT BIO (Seongnam, Korea) and maintained in our animal facility. 8- to 12-week-old mice were used in this study. For in vivo experiments, 10-week-old Balb/c mice were used. The weight range of mice was 22–26 g. All animal experiments were performed in accordance with the Institutional Guideline for Animal Use and Care of Jeju National University (approval No. 2019-0002, 2019-0034). DSF was dissolved in dimethyl sulfoxide to appropriate concentrations. Lipopolysaccharide (LPS) purified from Escherichia coli O55 was purchased from Sigma (St. Louis, MO, USA) and dissolved in sterile phosphate buffered saline.
BMs were prepared from femur and tibia of mice by flushing as established in our lab [7]. BMs were treated with Ammonium-Chloride-Potassium Lysing Buffer (Thermo Fisher Scientific, Waltham, MA, USA) to remove red blood cells. The cells were then passed through a 70 µm cell strainer to obtain single cells. To culture BMs, 5% complete medium (RPMI 1640 medium containing 5% fetal bovine serum and 100 IU/ml penicillin/streptomycin, 2 mM L-glutamine) was used.
For measurement of BM metabolic activity, BMs were cultured in 96-well culture plates at a concentration of 1 × 106 cells/ml (200 µl/well) and treated with DSF. After 3 days of culture, Cell Counting Kit-8 (CCK-8; Dojindo Molecular Technologies, Kumamoto, Japan) solution was added 10 µl/well for 4 h. The viable cells generate orange-colored products in proportion to their metabolic activity. The optical density of samples was measured at 450 nm using a microplate reader (Multiskan FC; Thermo Fisher Scientific) [8].
BMs were cultivated at a concentration of 1 × 106 cells/ml in 6-well culture plates and treated with DSF for 3 days. The treated BMs were harvested and used for flow cytometry analysis. To measure apoptosis, the cells were stained with annexin V-fluorescein isothiocyanate (FITC) and 0.25 µg/ml propidium iodide (PI). To check mitochondrial membrane potential (MMP) in the BMs, the cells were incubated with 10 µg/mL rhodamine 123 (Sigma) for 30 min at room temperature. Additionally, to detect the proportion of granulocytes and B cells, allophycocyanin-labeled anti-Gr-1 antibody and biotin-labeled anti-B220 antibody, streptavidin-FITC were used. To analyze dendritic cell (DC)-specific marker expression, the cells were stained with FITC-labeled anti-MHC II antibody, phycoerythrin-labeled anti-CD11c antibody. All stained cells were analyzed with CytoFLEX flow cytometer and CytExpert software, or BD LSRFortessa Cell Analyzer and FlowJo software (all from BD Biosciences, San Diego, CA, USA).
DSF was injected at a dosage of 50 mg/kg by intraperitoneal injection three times every other day (day 0, day 2, day 4). To measure the effects of DSF in vivo, the mice were divided into four groups including control. Mice were sacrificed on day 3, day 5, day 7. At indicated days, BMs were harvested from the femur and tibia of the mice. The cell number was counted by a trypan blue exclusion test and marker analysis was performed.
Data in graphs were presented as mean ± standard deviation (SD). Flow cytometry data were obtained from more than 3 independent experiments. Statistical significance was analyzed by Student’s t-test or one-way ANOVA, followed by Tukey-Kramer multiple comparison test using GraphPad Prism (GraphPad Software, San Diego, CA, USA). A p-value of < 0.05 was considered as significant. *, **, *** indicate p < 0.05, 0.01, 0.001 compared to the control, respectively.
To assess the effects of DSF on BMs, they were treated in the absence or presence of 1 µg/ml lipopolysaccharide (LPS, a representative inflammatory agent) and DSF over a range of concentrations (0–5 µM). The CCK-8 assay demonstrated that LPS significantly increased the metabolic activity of BMs compared to control BMs (Fig. 1). DSF significantly decreased the cellular activity of BMs in the absence or presence of LPS. DSF suppressed basal and LPS-induced metabolic activity in BMs.
To investigate how DSF affects the metabolic activity of BMs, we measured MMP of the cells. DSF-treated BMs were stained with rhodamine 123 solution. DSF significantly decreased the MMP of BMs at a range of DSF concentrations (0.04–5 µM) (Fig. 2B), indicating that DSF can destabilize the double-membrane structure of the mitochondria. Destabilization of the mitochondrial membrane in cells is closely correlated with cell death [9]. To determine whether DSF induces the death of BMs, the cells were stained with annexin V-FITC and PI. This quantitative cell death analysis revealed that DSF significantly increased the numbers of late apoptotic cells (annexin V+/PI+) and necrotic cells (annexin V–/PI+) compared to the control at DSF concentration of 1 and 5 µM (Fig. 2D).
To investigate how DSF influences the population of BMs, we measured the expression of subset-specific markers, B220 and Gr-1, on DSF-treated BMs (Fig. 3). Flow cytometry analysis revealed that DSF did not significantly affect the percentage of Gr-1- or B220-positive cells. These results indicate that DSF does not damage the ratio of BM subsets.
GM-CSF is an essential growth and differentiation factor for BMs [10]. To investigate whether DSF affects BMs, we measured the metabolic activity and differentiation of BMs after GM-CSF and DSF treatment. The metabolic activity of GM-CSF-treated BMs was not affected by DSF at lower concentrations (0–0.06 µM), whereas it was significantly affected at higher concentrations (0.125–0.5 µM) (Fig. 4A). In addition, 1 and 5 µM DSF blocked the differentiation of GM-CSF-treated BMs to CD11c+ DCs (Fig. 4B). These results demonstrated that DSF markedly impaired the metabolic activity and differentiation of GM-CSF-treated BMs.
To evaluate the effects of DSF on the BMs in vivo, we injected mice with DSF (Fig. 5A). In this study, the mice were intraperitoneally injected with 50 mg/kg DSF, as described in the Methods. To measure the myelosuppressive effects of DSF, we counted the number of BMs. DSF marginally decreased the number of BMs in mice compared to control, but without statistical significance. A graph comparing the control and DSF (day 5) group is presented in Fig. 5B, which showed that DSF at the dosage does not significantly suppress the bone marrow in vivo. The major subsets of BMs were analyzed using surface markers, Gr-1 and B220 (Fig. 5C, D). The marker analysis revealed that DSF does not significantly change the proportion of granulocytes and B lymphocytes in BMs.
DSF has been used for the treatment of alcohol dependence by blocking ALDH [2]. Recent studies have demonstrated anti-cancer effects of DSF on solid and hematological malignancies. In breast cancer cells, DSF inhibits ALDH activity and modulate intracellular reactive oxygen species (ROS) generation [11]. In addition, DSF was identified as a novel cancer selective growth inhibitory compound for prostate cancer cells via high-throughput cell-based screening [12]. However, there have been few studies on the effects of DSF on BMs, although the mechanisms underlying the effects of DSF on cancer cells have been investigated. In this study, we demonstrated that DSF suppressed BMs, especially granulocytes, in vitro.
The metabolic activity of DSF-treated BMs was measured using the CCK-8 assay (Fig. 1). In vitro, DSF inhibits the metabolic activity of BMs. To assess the effects of DSF on inflammation, we stimulated the activity of BMs using LPS. DSF dramatically reduces the metabolic activity of BMs activated by LPS. While there was no significant change at low concentrations of DSF (0–0.04 µM), the metabolic activity of BMs was significantly decreased at DSF concentrations of 0.2–5 µM in the presence of LPS and 1–5 µM of DSF in the absence of LPS (Fig. 1). In addition, the MMP and death of DSF-treated BMs were investigated by flow cytometry. Similar to the results of the CCK-8 assay, the MMP of DSF-treated BMs was also decreased at DSF concentrations of 0.04–5 µM compared to the control (Fig. 2B). Considering that reduction of MMP in cells is related to cell death [9], DSF may cause the death of BMs. Annexin V-FITC/PI staining revealed that 1 and 5 µM DSF significantly induced the necrosis and late apoptosis of BMs (Fig. 2D).
To investigate which types of cells in BMs were affected by DSF, we performed flow cytometry; subset-specific marker analysis was performed using B220 and Gr-1 (Fig. 3). B220 is a B cell-specific marker [13] and Gr-1 is commonly used as a granulocyte marker [14]. Subset analysis demonstrated that DSF did not significantly affect the proportion of BMs.
A recent study reported that DSF/copper increased the level of ROS and the expression of superoxide dismutase (SOD)2, a closely associated enzyme [15]. Hydrogen peroxide produced by SOD in neutrophils has been considered the main mediator of ROS-induced neutrophil apoptosis [16]. These findings suggest that DSF may cause the death of BMs by increasing SOD and ROS production. The relationship between DSF, SOD, and damage to BMs need to be further studied.
To investigate whether DSF affects the metabolic activity and differentiation of BMs modulated by a growth factor or cytokine, we used GM-CSF, an essential growth/differentiation factor for BM-derived DCs [17]. The CCK-8 assay revealed that DSF affected the metabolic activity of GM-CSF-treated BMs in a concentration-dependent manner (Fig. 4A). The metabolic activity of GM-CSF-treated BMs was not significantly decreased by 0–0.06 µM DSF; however, it was markedly decreased by 0.125–0.5 µM DSF. Furthermore, flow cytometry using the DC-specific marker, CD11c, showed that 1–5 µM DSF significantly inhibited DC generation from BMs (Fig. 4B). These results demonstrated that DSF affects the metabolic activity of BMs modulated by GM-CSF and can block the differentiation of DCs from BMs over a certain concentration.
To evaluate the toxicity of DSF on BMs in vivo, we injected mice with DSF 50 mg/kg via the peritoneal cavity (Fig. 5A). The dose of DSF was determined based on several previous studies regarding the anti-cancer effects of DSF in vivo [18-20]. The results of BM cellularity showed that 50 mg/kg DSF did not significantly decrease the cell number of BMs in mice (Fig. 5B). On Day 5, BMs were harvested and marker analysis was performed; there was no significant proportional change in the subsets of BMs compared to the control (Fig. 5C, D). Therefore, we searched the potential defense mechanisms against DSF in vivo to explain the discrepancy between the in vitro and in vivo results. There are several antioxidant mechanisms in vivo including SOD that can reduce the oxidative stress caused by ROS [21,22]. In a previous in vitro study, DSF increased SOD and ROS [23]. It may work similarly in vivo; however, DSF 50 mg/kg is estimated to be tolerated in mice by antioxidant defense mechanisms. Although 50 mg/kg DSF did not induce in vivo toxicity in BMs in this study, it is likely that DSF doses over 50 mg/kg may induce myelosuppression in mice, given that 200, 400 or 800 mg/kg DSF have been shown to be genotoxic in BMs [24]. Future studies are needed to investigate DSF toxicity in vivo and action mechanisms.
REFERENCES
1. Mutschler J, Grosshans M, Soyka M, Rösner S. 2016; Current findings and mechanisms of action of disulfiram in the treatment of alcohol dependence. Pharmacopsychiatry. 49:137–141. DOI: 10.1055/s-0042-103592. PMID: 26987743.


2. Petersen EN. 1992; The pharmacology and toxicology of disulfiram and its metabolites. Acta Psychiatr Scand Suppl. 369:7–13. DOI: 10.1111/j.1600-0447.1992.tb03309.x. PMID: 1471556.


3. O'Farrell TJ, Allen JP, Litten RZ. 1995; Disulfiram (antabuse) contracts in treatment of alcoholism. NIDA Res Monogr. 150:65–91. DOI: 10.1037/e495792006-007. PMID: 8742773.
4. Viola-Rhenals M, Patel KR, Jaimes-Santamaria L, Wu G, Liu J, Dou QP. 2018; Recent advances in antabuse (Disulfiram): the importance of its metal-binding ability to its anticancer activity. Curr Med Chem. 25:506–524. DOI: 10.2174/0929867324666171023161121. PMID: 29065820. PMCID: PMC6873226.


5. Jiao Y, Hannafon BN, Ding WQ. 2016; Disulfiram's anticancer activity: evidence and mechanisms. Anticancer Agents Med Chem. 16:1378–1384. DOI: 10.2174/1871520615666160504095040. PMID: 27141876.


6. Maxwell MB, Maher KE. 1992; Chemotherapy-induced myelosuppression. Semin Oncol Nurs. 8:113–123. DOI: 10.1016/0749-2081(92)90027-Z. PMID: 1621002.


7. Kwon MJ, Joo HG. 2018; Dapsone modulates lipopolysaccharide-activated bone marrow cells by inducing cell death and down-regulating tumor necrosis factor-α production. J Vet Sci. 19:744–749. DOI: 10.4142/jvs.2018.19.6.744. PMID: 30304888. PMCID: PMC6265590.


8. Lee YJ, Han Y, Joo HG. 2020; Bordetella bronchiseptica is a potent and safe adjuvant that enhances the antigen-presenting capability of dendritic cells. Korean J Physiol Pharmacol. 24:47–52. DOI: 10.4196/kjpp.2020.24.1.47. PMID: 31908574. PMCID: PMC6940501.


9. Ly JD, Grubb DR, Lawen A. 2003; The mitochondrial membrane potential (deltapsi(m)) in apoptosis; an update. Apoptosis. 8:115–128. DOI: 10.1023/A:1022945107762. PMID: 12766472.
10. Becher B, Tugues S, Greter M. 2016; GM-CSF: from growth factor to central mediator of tissue inflammation. Immunity. 45:963–973. DOI: 10.1016/j.immuni.2016.10.026. PMID: 27851925.


11. Yang Z, Guo F, Albers AE, Sehouli J, Kaufmann AM. 2019; Disulfiram modulates ROS accumulation and overcomes synergistically cisplatin resistance in breast cancer cell lines. Biomed Pharmacother. 113:108727. DOI: 10.1016/j.biopha.2019.108727. PMID: 30870721.


12. Iljin K, Ketola K, Vainio P, Halonen P, Kohonen P, Fey V, Grafström RC, Perälä M, Kallioniemi O. 2009; High-throughput cell-based screening of 4910 known drugs and drug-like small molecules identifies disulfiram as an inhibitor of prostate cancer cell growth. Clin Cancer Res. 15:6070–6078. DOI: 10.1158/1078-0432.CCR-09-1035. PMID: 19789329.


13. Coffman RL, Weissman IL. 1981; B220: a B cell-specific member of th T200 glycoprotein family. Nature. 289:681–683. DOI: 10.1038/289681a0. PMID: 6970340.


14. Fleming TJ, Fleming ML, Malek TR. 1993; Selective expression of Ly-6G on myeloid lineage cells in mouse bone marrow. RB6-8C5 mAb to granulocyte-differentiation antigen (Gr-1) detects members of the Ly-6 family. J Immunol. 151:2399–2408. PMID: 8360469.
15. Hassani S, Ghaffari P, Chahardouli B, Alimoghaddam K, Ghavamzadeh A, Alizadeh S, Ghaffari SH. 2018; Disulfiram/copper causes ROS levels alteration, cell cycle inhibition, and apoptosis in acute myeloid leukaemia cell lines with modulation in the expression of related genes. Biomed Pharmacother. 99:561–569. DOI: 10.1016/j.biopha.2018.01.109. PMID: 29902866.


16. Yasui K, Kobayashi N, Yamazaki T, Agematsu K, Matsuzaki S, Ito S, Nakata S, Baba A, Koike K. 2005; Superoxide dismutase (SOD) as a potential inhibitory mediator of inflammation via neutrophil apoptosis. Free Radic Res. 39:755–762. DOI: 10.1080/10715760500104066. PMID: 16036355.


17. Helft J, Böttcher J, Chakravarty P, Zelenay S, Huotari J, Schraml BU, Goubau D, Reis e Sousa C. 2015; GM-CSF mouse bone marrow cultures comprise a heterogeneous population of CD11c+MHCII+ macrophages and dendritic cells. Immunity. 42:1197–1211. DOI: 10.1016/j.immuni.2015.05.018. PMID: 26084029.


18. Skrott Z, Mistrik M, Andersen KK, Friis S, Majera D, Gursky J, Ozdian T, Bartkova J, Turi Z, Moudry P, Kraus M, Michalova M, Vaclavkova J, Dzubak P, Vrobel I, Pouckova P, Sedlacek J, Miklovicova A, Kutt A, Li J, et al. 2017; Alcohol-abuse drug disulfiram targets cancer via p97 segregase adaptor NPL4. Nature. 552:194–199. DOI: 10.1038/nature25016. PMID: 29211715. PMCID: PMC5730499.


19. Bu W, Wang Z, Meng L, Li X, Liu X, Chen Y, Xin Y, Li B, Sun H. 2019; Disulfiram inhibits epithelial-mesenchymal transition through TGFβ-ERK-Snail pathway independently of Smad4 to decrease oral squamous cell carcinoma metastasis. Cancer Manag Res. 11:3887–3898. Erratum. DOI: 10.2147/CMAR.S348759. PMID: 34858055. PMCID: PMC8630542.
20. Xu X, Xu J, Zhao C, Hou X, Li M, Wang L, Chen L, Chen Y, Zhu L, Yang H. 2019; Antitumor effects of disulfiram/copper complex in the poorly-differentiated nasopharyngeal carcinoma cells via activating ClC-3 chloride channel. Biomed Pharmacother. 120:109529. DOI: 10.1016/j.biopha.2019.109529. PMID: 31606620.


21. Valko M, Rhodes CJ, Moncol J, Izakovic M, Mazur M. 2006; Free radicals, metals and antioxidants in oxidative stress-induced cancer. Chem Biol Interact. 160:1–40. DOI: 10.1016/j.cbi.2005.12.009. PMID: 16430879.


22. Niki E. 2010; Assessment of antioxidant capacity in vitro and in vivo. Free Radic Biol Med. 49:503–515. DOI: 10.1016/j.freeradbiomed.2010.04.016. PMID: 20416370.
23. Salem K, McCormick ML, Wendlandt E, Zhan F, Goel A. 2015; Copper-zinc superoxide dismutase-mediated redox regulation of bortezomib resistance in multiple myeloma. Redox Biol. 4:23–33. DOI: 10.1016/j.redox.2014.11.002. PMID: 25485927. PMCID: PMC4309843.


24. Madrigal-Bujaidar E, Velazquez-Guadarrama N, Morales-Ramirez P, Mendiola MT, Martínez AL, Chamorro G. 1999; Sister-chromatid exchanges induced by disulfiram in bone marrow and spermatogonial cells of mice treated in vivo. Food Chem Toxicol. 37:757–763. DOI: 10.1016/S0278-6915(99)00061-7. PMID: 10496378.


Fig. 1
DSF decreases the metabolic activity of BMs.
BMs (1 × 106 cells/ml) were incubated in 96-well culture plates and treated with DSF at the indicated concentrations (0–5 µM) in the absence or presence of 1 µg/ml LPS. After 3 days, the CCK-8 assay was performed. Data are presented as mean ± SD. DSF, disulfiram; BMs, bone marrow cells; LPS, lipopolysaccharide; CCK-8, Cell Counting Kit-8; O.D., optical density. ***, ###indicate p < 0.001 compared to BMs treated without LPS or LPS alone (DSF 0 µM), respectively.
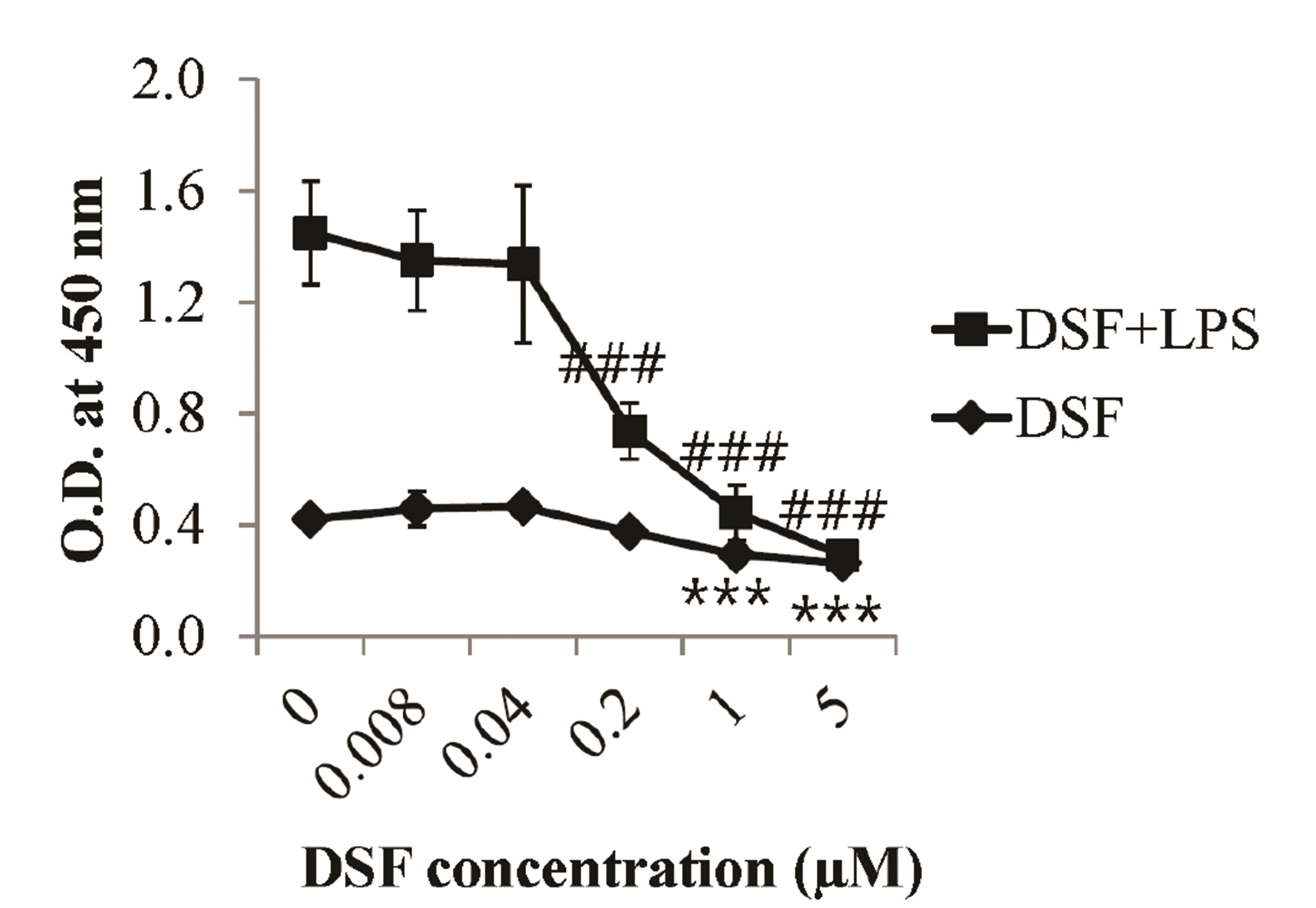
Fig. 2
DSF diminishes the MMP and causes the death of BMs.
BMs were cultured in 6-well culture plates (1 × 106 cells/ml) and treated with DSF at the indicated concentrations (0–5 µM). To measure MMP, treated BMs were stained with rhodamine 123 solution. The number in the histograms indicates mean fluorescence intensity (MFI) (A). The control group was set to 100% and the MFI of each histogram was calculated (B). The cells were treated for 2 days and stained with annexin V-FITC/PI. The cells in quadrants indicate necrosis (upper left), late apoptosis (upper right), early apoptosis (lower right), and viable (lower left) cells. The number in quadrants indicates the percentage of cells (C) and the percentage of PI-positive cells was presented (D). DSF, disulfiram; MMP, mitochondrial membrane potential; BMs, bone marrow cells; V-FITC, V-fluorescein isothiocyanate; PI, propidium iodide. *, **Indicate p < 0.05, 0.01 compared to the control (DSF concentration 0 μM), respectively.
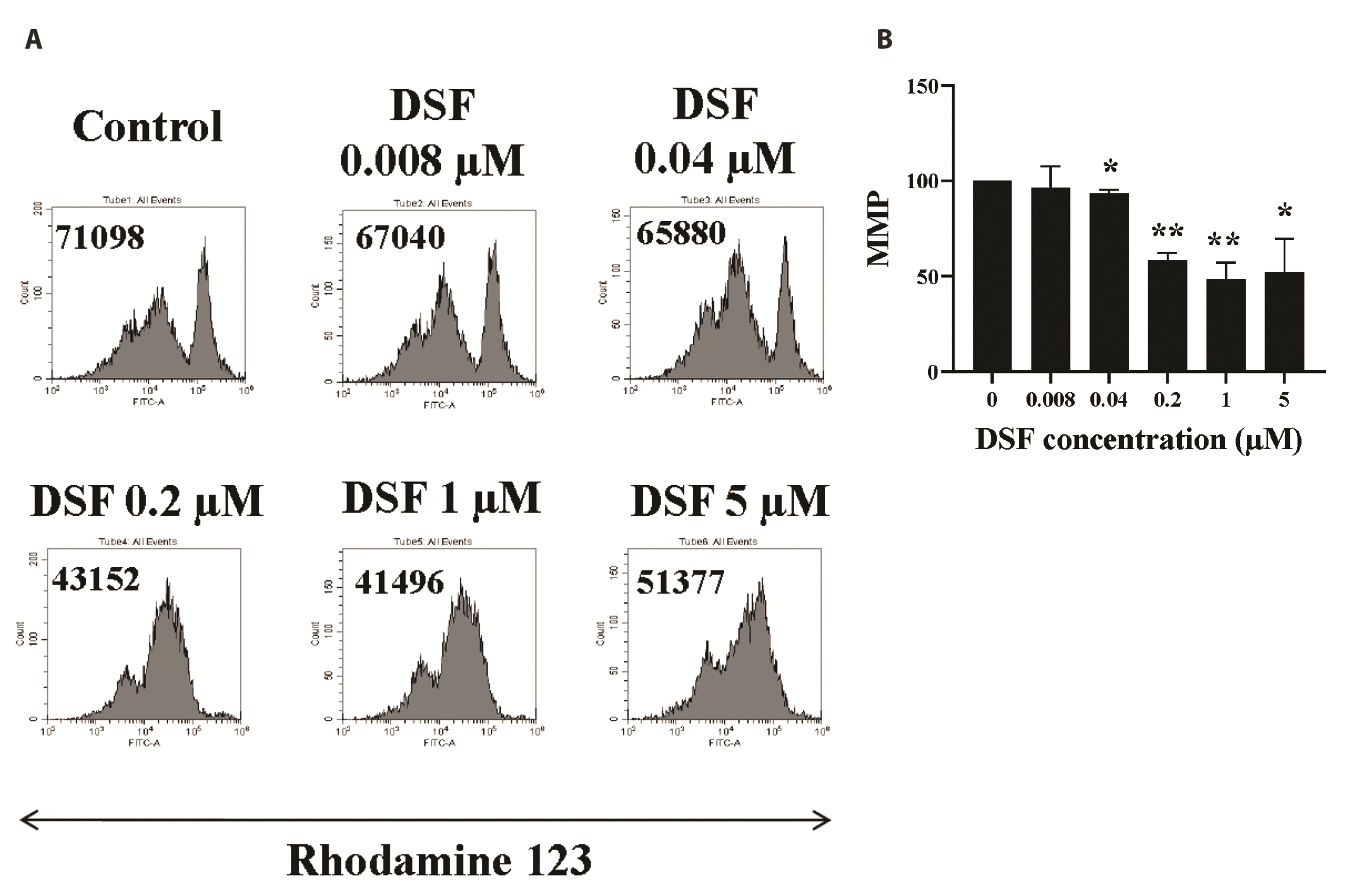

Fig. 3
DSF does not affect the ratio of BM subsets.
BMs (1 × 106 cells/ml) were seeded in 24-well culture plates and treated with DSF. The representative dot plots (A) and the percentage of positive cells (B) were presented. DSF, disulfiram; BMs, bone marrow cells.

Fig. 4
DSF impairs the metabolic activity and differentiation of GM-CSF-treated BMs.
BMs (1 × 106 cells/ml) were incubated in 96-well culture plates and treated with DSF at the indicated concentrations (0–0.5 µM) in the absence or presence of 10 ng/ml GM-CSF for 3 days. The metabolic activity of BMs was measured using the CCK-8 assay (A). Data are expressed as the mean ± SD. To investigate the differentiation to DCs, BMs treated with GM-CSF and DSF were stained with DC-specific markers, CD11c. The percentage of CD11c-positive cells after gating a region based on forward scatter (FSC) and side scatter (SSC) was presented (B). DSF, disulfiram; GM-CSF, granulocyte-macrophage colony-stimulating factor; BMs, bone marrow cells; CCK-8, Cell Counting Kit-8; DC, dendritic cell; O.D., optical density. **, ##, ### indicate p < 0.01 and 0.001 compared to the control BMs in the absence or presence of GM-CSF, respectively.
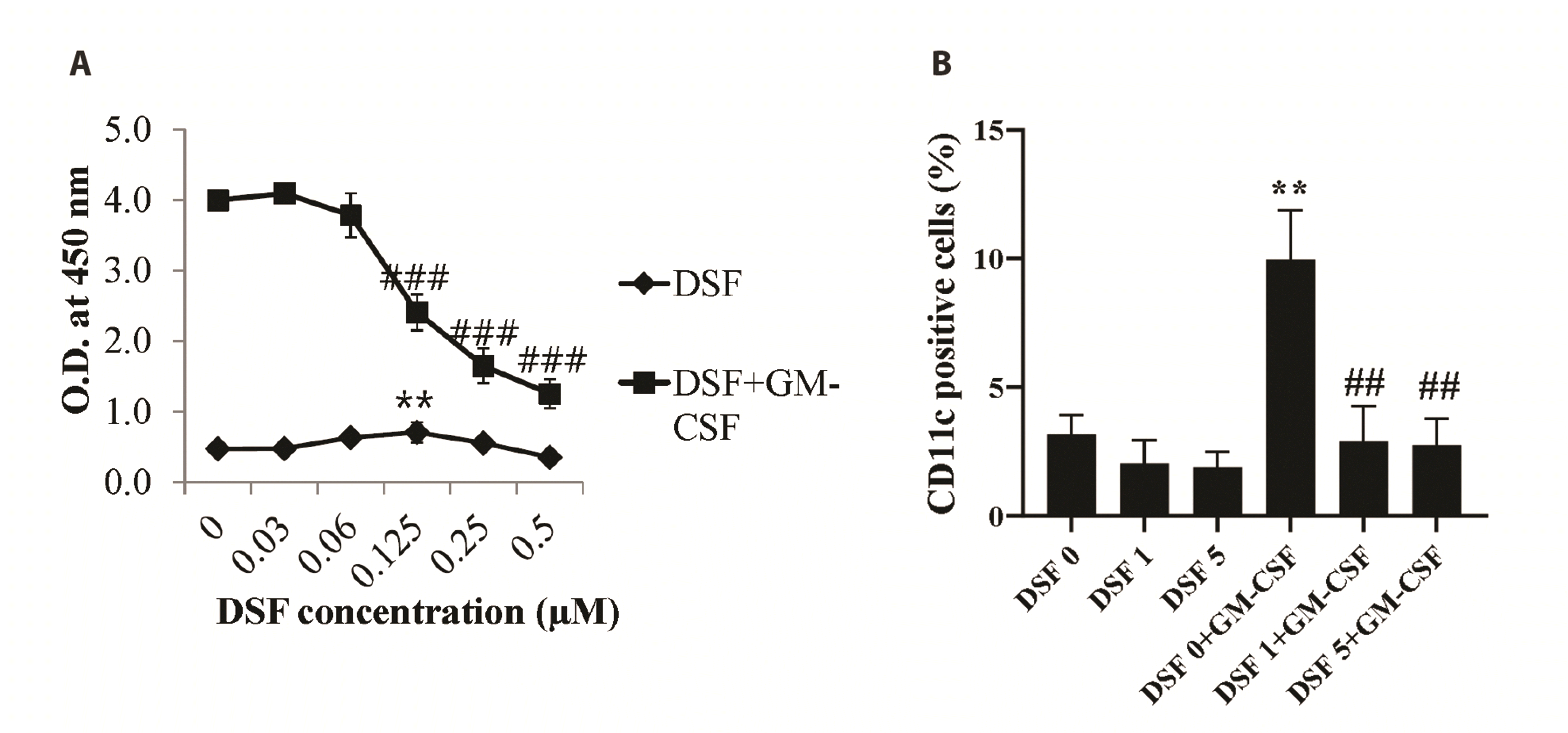
Fig. 5
DSF does not significantly induce myelosuppression in vivo.
The mice were intraperitoneally injected with 50 mg/kg DSF every 2 days, on day 0, 2, and 4 (A). Control mice were injected with DMSO alone. The mice were sacrificed at indicated times and BMs were harvested. The cellularity of BMs was calculated using the trypan blue exclusion test. The graph was generated using in vivo data of 12 mice in each group from more than three independent experiments (B). The representative subset proportion (C) and the percentage of positive cells (D) were presented. DSF, disulfiram; DMSO, dimethyl sulfoxide; BMs, bone marrow cells; ns, non-significant.
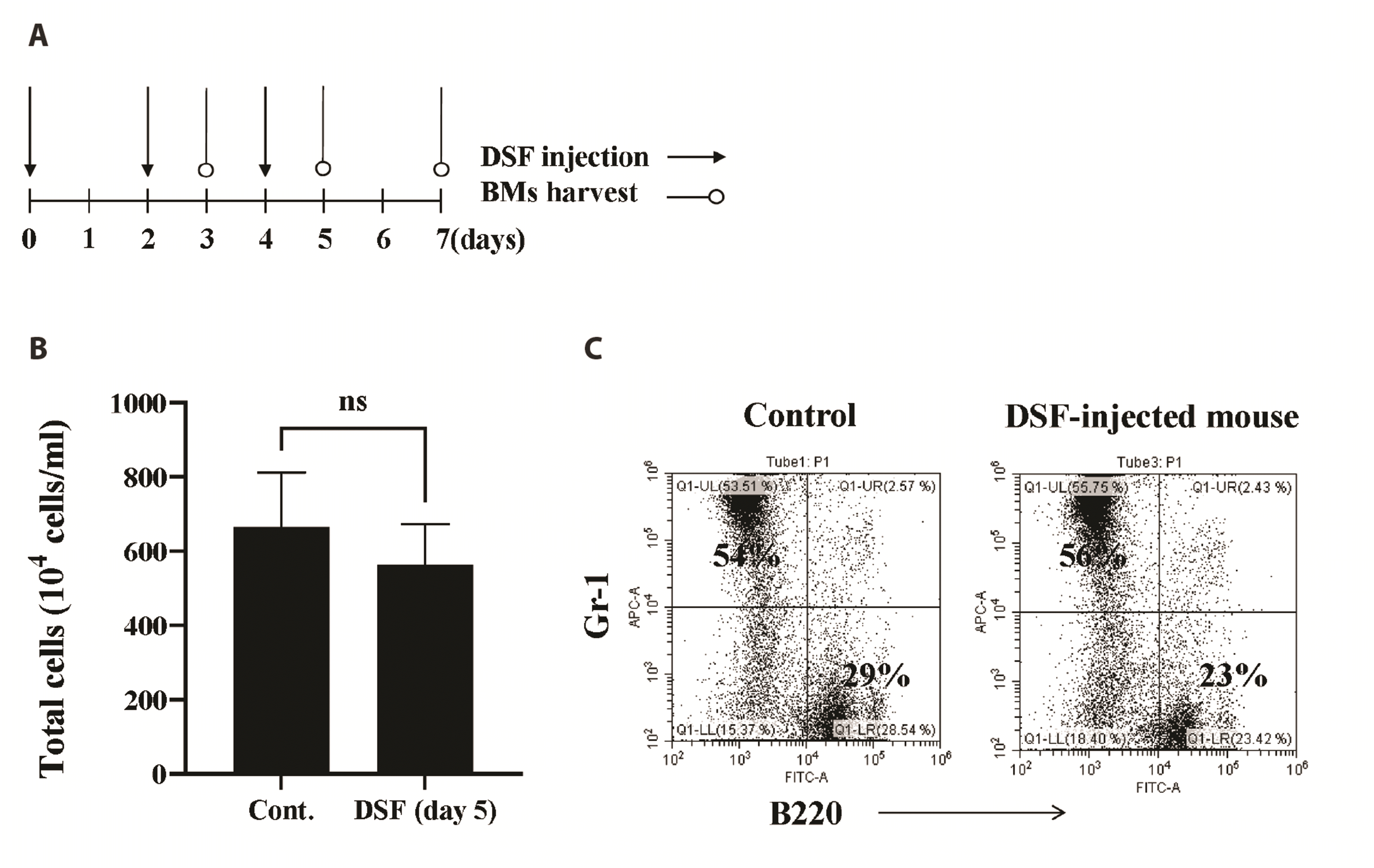
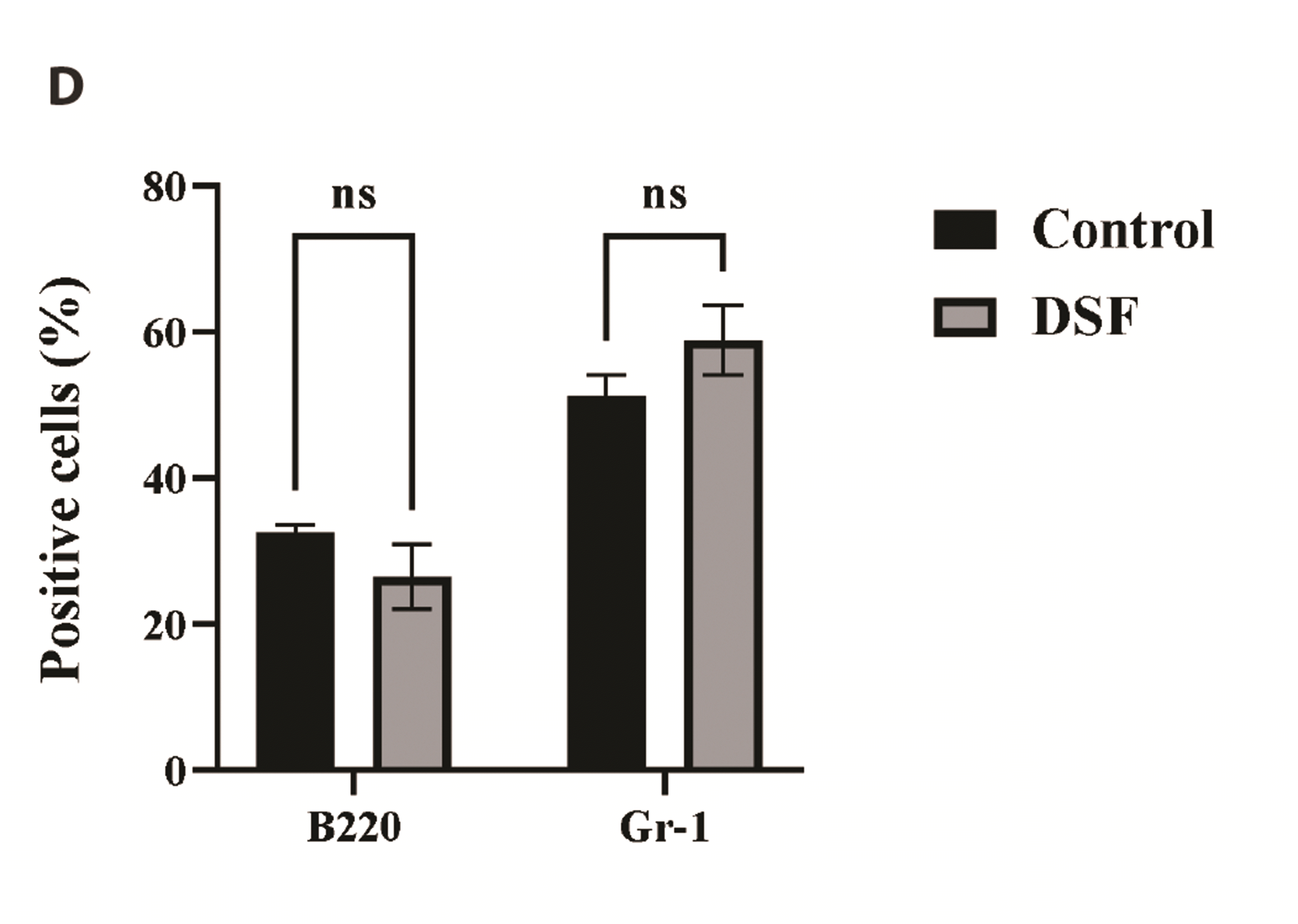