Abstract
Background
In this study, we aimed to investigate the prevalence, timing, risk factors, and outcomes of acute respiratory distress syndrome (ARDS) in patients with aneurysmal subarachnoid hemorrhage (aSAH).
Methods
PubMed and four other databases were searched for randomized controlled trials (RCTs) and observational studies of patients 18 years or older through October 20, 2021. Study quality was assessed, using the Cochrane Risk of Bias tool for RCTs and the Newcastle-Ottawa scale for cohort and case-control studies. High-grade aSAH was defined as a Hunt-Hess grade ≥3 and/or a modified Fisher score ≥3. A good neurological outcome was defined as a Glasgow outcome scale score ≥4. Random-effects meta-analyses were conducted to estimate the pooled outcome prevalence and 95% confidence interval (CI).
Results
Eleven observational studies (n=6,107) met the inclusion criteria. Overall, 15% of the patients (95% CI, 10.5–20.0; I2=97.8%) developed ARDS after aSAH, with a mean time of 3 days (95% CI, 1.9–3.7; I2=54%). Overall survival at discharge was 80% (95% CI, 75–86; I2=96%), and 67% of aSAH patients (95% CI, 54.9–78.9; I2=94%) had a good neurological outcome at any time. The aSAH cohort without ARDS had a higher rate of survival than those with ARDS (79% vs. 49%, P=0.028). Male sex, patients with a high-grade aSAH, patients who developed pneumonia, and systemic inflammatory response syndrome during hospital admission were at a higher risk of developing ARDS.
Aneurysmal subarachnoid hemorrhage (aSAH) accounts for 3% of all stroke, affecting approximately 30,000–40,000 patients annually in the United States and 600,000 worldwide [1]. Although mortality due to aSAH has decreased in the past two to three decades, the 30-day mortality remains high, at approximately 25%–35% [2]. Non-neurological complications, such as cardiopulmonary injuries and systemic inflammation, are frequently seen in patients with aSAH, and may be responsible for >10% of the deaths in these patients. Other neurological risk factors include the severity of the initial hemorrhage, the timing of surgical or endovascular intervention, and the presence of delayed cerebral ischemia (DCI) [3].
Acute respiratory distress syndrome (ARDS) is a heterogeneous syndrome that is characterized by diffuse damage in the parenchyma of the lung, which is the result of the lung’s innate inflammatory response to an injury, along with non-cardiogenic pulmonary edema due to increased alveolar-capillary vascular permeability and the accumulation of protein-rich edematous fluid, resulting in impaired gas exchange and refractory hypoxemia [4]. At present, the mechanism of ARDS, particularly when it occurs after aSAH, is poorly understood. It is thought, however, to be similar to what occurs in patients with traumatic brain injury, which involves a massive sympathetic storm and systemic inflammation in response to the initial brain injury and intracranial pressure (ICP) crisis, subsequently leading to systemic arterial and pulmonary hypertension, as well as increased vascular permeability and pulmonary edema [5,6].
Increasing evidence shows that pulmonary edema and arterial hypoxemia due to ARDS are significantly associated with poor neurological outcomes, increased mortality, and prolonged hospital stays in patients with aSAH [7,8]. Despite its impact on outcomes and mortality in patients with aSAH, information on the prevalence, timing, and risk factors of ARDS is sparse, and prior studies have reported a wide range of ARDS prevalence, ranging from 11% to 60% of patients with acute aSAH [9,10]. The primary aim of the present study was to investigate the prevalence, timing, risk factors, and outcomes of ARDS in patients with aSAH.
The present systematic review/meta-analysis was reported using the Preferred Reporting Items for Systematic Reviews and Meta-Analyses (PRISMA) guidelines [11]. We searched five databases for subject headings and controlled vocabulary, as well as keywords and language relevant to acute lung injury (ALI), ARDS, and brain injury, as follows: PubMed, both the legacy (results=4,991) and new (results=5,804) databases via The National Center for Biotechnology Information (NCBI); Embase via Elsevier (results=10,216); the Cochrane Library via Wiley (results=791); the Web of Science Core Collection via Clarivate (results=5,249); and Scopus via Elsevier (results=11,193). The initial search was conducted from the inception of the databases through July 6, 2020. An updated search was performed on October 20, 2021. There were no language limitations to this study, and all efforts were made to account for plural words, acronyms, and synonyms. In total, 38,194 results were obtained, from which 20,151 duplicates were removed, leaving 18,043 results. Data deduplication was performed using EndNote X9 (Endnote, Clarivate; available at https://www.endnote.com), after which the results were uploaded to Covidence (Veritas Health Innovation, Melbourne, Australia; available at https://www.covidence.org) for title and abstract screening, and were further reviewed for eligibility. All articles that met the inclusion criteria were retrieved, and the full texts were reviewed. References from the included studies were also manually reviewed to search for additional relevant studies. Supplementary Material 1 presents the details of the search strategy used in our review.
The inclusion criteria were as follows: (1) all randomized controlled trials (RCTs) and observational studies with adult patients (>18 years old); and (2) studies involving ALI or ARDS, as defined by either the American-European Consensus Conference (AECC) or the Berlin criteria, which occurred after aSAH [12,13]. Studies that reported patients with ALI based on the AECC criteria, defined as a PaO2/FiO2 (P/F) ratio between 201 and 300, were classified as having mild ARDS for the present study, while patients with a P/F ratio ≤200 were classified as having moderate/severe ARDS [12,13]. The exclusion criteria were as follows: (1) editorials, commentaries, research protocols, reviews (including systematic reviews and meta-analyses), case series/reports, abstracts, and articles only available in foreign languages; (2) articles with pediatric populations (<18 years old); (3) studies without a description or definition of ALI or ARDS; (4) animal and in vitro studies; and (5) ARDS that occurred prior to aSAH. Additionally, case-control studies were excluded from the analysis of the prevalence of ARDS after aSAH.
The literature results were independently assessed by two reviewers (THF and MH) for eligibility, and any disagreements on the inclusion or exclusion were resolved by a third reviewer (SMC). Data were extracted from eligible studies and recorded in an Excel spreadsheet (Microsoft, Redmond, WA, USA). Full texts and charts were reviewed in detail for data regarding study design, study population, number of patients with high-grade aSAH (defined based on clinical severity as a Hunt Hess grade ≥3, and radiographic severity as a modified Fisher score ≥3), patient characteristics (age, sex, ethnicity, and baseline comorbidities), complications during the hospital stay, including pneumonia, sepsis, systemic inflammatory response syndrome (SIRS; defined as having ≥2 of the following conditions: temperature <36°C or >38°C heart rate >90 beats/min, respiratory rate >20 breaths/min, and/or white blood cell count >12,000 or <4,000 cells/mm3), ARDS variables (etiology, severity, and P/F ratio), Acute Physiology and Chronic Health Evaluation II (APACHE II) score, neurological outcomes, and survival. The Hunt and Hess grade for SAH severity was used instead of the World Federation of Neurosurgical Societies grading system because it was more commonly reported among the included studies.
The primary outcome evaluated was the prevalence of ARDS which occurred after aSAH. Secondary outcomes included the timing of ARDS development, survival at hospital discharge, and prevalence of good neurological outcomes of discharged patients at any time after a diagnosis of aSAH (defined as a Glasgow Outcome Scale score ≥4) [14].
The Newcastle-Ottawa Scale (NOS) was used to assess the quality of nonrandomized studies and to evaluate the risk of bias in case-control and cohort studies. The NOS scores were based on three indices: patient selection, comparability, and assessment of outcome or exposure [15]. Studies scoring ≥6 points were considered to have a low risk for bias. Publication quality was assessed independently by two investigators (THF and MH), and any discrepancies were resolved by consensus with a third investigator (SMC).
We performed the present systematic review according to the PRISMA guidelines (Supplementary Material 2) [10]. The prevalence of each outcome was calculated for each study based on the number of patients with a specific outcome divided by the total number of patients with SAH. This was then pooled for the meta-analysis including all studies. For all meta-analyses of prevalence, we used random effects models with the inverse variance method, while the restricted maximum likelihood model was used to produce unbiased estimates of variance and covariance parameters. The Sidik-Jonkman estimator was used to calculate tau [16], and the Hartung-Knapp adjustment was used to calculate confidence intervals (CI) [17]. The Freeman-Tukey double arcsine transformation was used to calculate the prevalence for all outcomes. Heterogeneity was assessed using the Cochrane Q statistic (chi-square test), and the magnitude of the heterogeneity was evaluated using the I2 statistic [18]. I2 quantified the degree of heterogeneity in a range of 0%–100% where 0%–40% indicates insignificant heterogeneity, 30%–60% indicates moderate heterogeneity, and 75%–100% indicates considerable heterogeneity. The following patient variables were evaluated for their association with the outcomes of ARDS, using the Mann-Whitney U-test for continuous variables and Fisher’s exact test for categorical variables: age, sex, presence of high-grade aSAH on initial presentation, presence of pneumonia, sepsis, SIRS, and DCI during hospital admission. A P-value <0.05 was considered to be statistically significant. For the present study, a meta-regression analysis was not performed, due to insufficient data. The analysis was performed using STATA 17 (StataCorp., College Station, TX, USA).
As indicated above, our search yielded 18,043 results after data deduplication. After title and abstract screening, 284 articles were found to be eligible for a full-text review, of which 273 were excluded based on the aforementioned exclusion criteria, leaving a total of 11 studies (n=6,107) to be included in the present study. Fig. 1 shows a flowchart of the selection process. The studies included five prospective (n=4,219) and six retrospective cohort studies (n=1,888). Details of the included studies can be found in Supplementary Table 1. The references for the included studies have been provided in Supplementary Material 3.
No RCTs were included in the present systematic review and meta-analysis. The NOS was used to evaluate the included observational studies, which did not indicate a high risk of bias for any of the studies, which had a median NOS score of 7 (Supplementary Table 2).
Of the 6,107 patients with aSAH included in the present systematic review and meta-analysis, the median age was 55 years (interquartile range, 52–58 years), and 35% were male (n=2,107). In this aSAH cohort, 39% of the patients had high-grade aSAH, 19% had sepsis, 11% had pneumonia, and 28% had SIRS. The pooled prevalence of patients who developed ARDS after aSAH was 15% (95% CI, 10.5–20.0; I2=97.8%) (Fig. 2) [8,19-28], and the mean time from the initial aSAH diagnosis to the development of ARDS was 3 days (95% CI, 1.9–3.7; I2=54%) (Fig. 3) [8,20,21,26-28]. An analysis of studies published prior to 2012, which used AECC for ARDS, compared to those published after 2012, which used the Berlin criteria for ARDS, showed no significant difference in the prevalence of ARDS (7% vs. 8%). Of the 11 included studies, 6 (n=1,567) reported the number of patients with mild ARDS, the pooled prevalence of which was 9.78% (95% CI, 6.3–13.2; I2 =81%) (Supplementary Fig. 1), while seven studies (n=1,923) reported the number of patients with moderate/severe ARDS, the pooled prevalence of which was 13% (95% CI, 7.8–15.3; I2=93%) (Supplementary Fig. 2).
Of the 11 included studies, five (n=2,313) reported the characteristics of patients with and without ARDS. The univariate analysis of the five studies showed that male sex (odd ratio [OR], 2.07; 95% CI, 1.5–2.8; P=0.04), high-grade aSAH (OR, 2.5; 95% CI, 1.8–3.5; P=0.02), the presence of pneumonia (OR, 4.4; 95% CI, 2.6–7.2; P=0.01), and SIRS (OR, 16.3; 95% CI, 5.5–48.7; P=0.02) during hospital admission were associated with a higher risk of developing ARDS in patients with aSAH. Sepsis and DCI were not found to be associated with an increased risk of ARDS (Supplementary Table 3).
A total of 10 studies (n=4,922) reported the number of in-hospital survivors of aSAH. The pooled overall survival at discharge after aSAH was 80% (95% CI, 75–86; I2=96%) (Fig. 4) [8,19-26,28], and we found that the survival rate was significantly lower in the aSAH cohort with ARDS than in those without (49% vs. 79%; OR, 0.24; 95% CI, 0.17–0.33; P=0.028).
Of the 11 included studies, six (n=3,939; 78%) reported the neurological outcomes after aSAH. Good neurological outcomes at any time were achieved in 67% of the patients with aSAH (95% CI, 54.9–78.9; I2=98%) (Fig. 5) [21,23,25-27,29]. Two studies (n=947) compared the neurological outcomes between patients with and without ARDS, and the ARDS group had significantly fewer patients with good neurological outcomes at any time after aSAH (25% vs. 61%; P<0.001).
To the best of our knowledge, this is the first systematic review and meta-analysis to evaluate the prevalence, timing, risk factors, and outcomes of ARDS after acute aSAH. The results of our study, which included 6,107 adult patients with aSAH, demonstrated a high prevalence of ARDS (15%) in this population. Unsurprisingly, we found that the development of ARDS after aSAH is associated with a 4-fold increased risk of mortality.
Interestingly, the mean time of development from aSAH to ARDS was 3 days, which highlights the important connection between the brain and the lungs, suggesting that the catecholamine surge and systemic inflammatory response after the onset of aSAH may be responsible for causing ARDS shortly after the initial injury, rather than infectious complications such as pneumonia. Although preclinical studies aiming to understand the mechanisms of aSAH-associated ARDS are sparse, neurogenic edema is a known cause of acute respiratory failure following aSAH [29,30]. aSAH is a significant acute brain injury and insult, which can result in activation of the sympathoadrenal axis with or without elevated ICP, leading to the vasoconstriction of peripheral vessels, elevated systemic arterial pressure, and increased pulmonary hydrostatic pressure, resulting in acute pulmonary edema [31]. Simultaneously, the systemic inflammatory response induced by aSAH can lead to a cytokine storm, causing end-organ damage and increased vascular permeability, which subsequently worsens pulmonary edema and ARDS [6]. Similarly, this massive sympathetic surge can result in stress cardiomyopathy, which can also cause pulmonary edema and imaging findings similar to those of bilateral pulmonary infiltrates, confounding the diagnosis of ARDS. Caution should be taken to rule out cardiogenic pulmonary edema when diagnosing ARDS in conjunction with aSAH [32].
Additionally, the results of the present study indicated that the presence of high-grade aSAH upon initial presentation, as well as the presence of SIRS, were associated with a higher risk of developing ARDS, which further supports the aforementioned hypothesis relating to the interplay between the brain and the lungs. We also found that male sex and the presence of pneumonia were associated with a higher risk of ARDS in patients with aSAH, which highlights that acute brain injury may not be the sole cause for ARDS [8,22,33]. One study found that in addition to developing ARDS within the first 3 days from the onset of aSAH, patient with aSAH can also experience significant deterioration of pulmonary function after day 4 [3,34]. Worsening pulmonary function, along with aspiration due to poor mental status, prolonged intubation, and hospital-acquired pneumonia, may all play a role in the development of aSAH-associated ARDS [34-36]. The early recognition and diagnosis of ARDS in patients with aSAH, as well as the implementation of lung protective ventilation, are important in improving the outcomes of these critically ill patients [37]. The use of lung protective ventilation is often complicated in aSAH patients with elevated ICP, as they may be sensitive to changes in respiratory mechanics and the associated cerebral perfusion pressure [4,5]. Some studies, however, have suggested that positive end-expiratory pressure has no significant effect on cerebral hemodynamics [38,39]. In the present study, we were unable to accurately assess the effects of changes in ventilation strategies over the years on the outcomes of these patients, due to insufficient data [40,41]. A better understanding of and research on brain-lung interactions, especially with a focus on understanding the exact pathophysiology of ARDS following aSAH and the interaction of high ICP with lung protective ventilation, are necessary.
The present study had several limitations. First, this study showed substantial heterogeneity (I2>90%) in estimating the prevalence of ARDS after aSAH, owing to the variability of the included studies, which represents the current state of limited data available on this topic. Publication bias may also exist, due to the nature of systematic reviews and meta-analyses, which could cause an overestimation of the prevalence of ARDS after aSAH. Similarly, we were unable to account for observer bias between the clinicians’ interpretations of the imaging and ventilation data used for the diagnosis of ARDS. Bilateral pulmonary infiltration, from pulmonary edema due to stress cardiomyopathy, should be ruled out before ARDS is diagnosed, although none of the included articles discussed this information. The included studies also involved a wide range of study periods, and we were therefore unable to account for differences in the prevalence of ARDS due to changes in clinical practice in patients with aSAH and changes in the definition of ARDS over time. We found, however, that the prevalence of ARDS did not change between studies done before and after 2012. Additionally, only half of the studies included reported neurological outcomes, which may not accurately represent the entire population of patients with aSAH. The timing of the neurological outcome assessments also had variable follow-up times. Lastly, the assessment of risk factors for ARDS was limited by missing data on pre-specified risk factor variables, and caution needs to be taken when interpreting the data, due to heterogeneities across the studies. Despite these limitations, the present study serves as a foundation for reporting the common occurrence of ARDS, as well as its timing and outcomes in patients with aSAH.
In the present meta-analysis, approximately one in six patients developed ARDS shortly after aSAH, with a mean time of 3 days, and patients with high-grade aSAH were more likely to develop ARDS. The presence of ARDS is associated with higher mortality and worse neurologic outcomes in these patients. As such, further research on prevention and treatment strategies for aSAH-associated ARDS is warranted.
Supplementary materials
Supplementary materials can be found via https://doi.org/10.18700/jnc.220043.
REFERENCES
1. Virani SS, Alonso A, Aparicio HJ, Benjamin EJ, Bittencourt MS, Callaway CW, et al. Heart disease and stroke statistics: 2021 update: a report from the American Heart Association. Circulation. 2021; 143:e254–743.
2. Lovelock CE, Rinkel GJ, Rothwell PM. Time trends in outcome of subarachnoid hemorrhage: population-based study and systematic review. Neurology. 2010; 74:1494–501.


3. Solenski NJ, Haley EC Jr, Kassell NF, Kongable G, Germanson T, Truskowski L, et al. Medical complications of aneurysmal subarachnoid hemorrhage: a report of the multicenter, cooperative aneurysm study. Crit Care Med. 1995; 23:1007–17.
4. Thompson BT, Chambers RC, Liu KD. Acute respiratory distress syndrome. N Engl J Med. 2017; 377:562–72.


5. Rogers FB, Shackford SR, Trevisani GT, Davis JW, Mackersie RC, Hoyt DB. Neurogenic pulmonary edema in fatal and nonfatal head injuries. J Trauma. 1995; 39:860–8.


6. Muroi C, Keller M, Pangalu A, Fortunati M, Yonekawa Y, Keller E. Neurogenic pulmonary edema in patients with subarachnoid hemorrhage. J Neurosurg Anesthesiol. 2008; 20:188–92.


7. Friedman JA, Pichelmann MA, Piepgras DG, McIver JI, Toussaint LG 3rd, McClelland RL, et al. Pulmonary complications of aneurysmal subarachnoid hemorrhage. Neurosurgery. 2003; 52:1025–32.


8. Kahn JM, Caldwell EC, Deem S, Newell DW, Heckbert SR, Rubenfeld GD. Acute lung injury in patients with subarachnoid hemorrhage: incidence, risk factors, and outcome. Crit Care Med. 2006; 34:196–202.


9. Feigin VL, Lawes CM, Bennett DA, Barker-Collo SL, Parag V. Worldwide stroke incidence and early case fatality reported in 56 population-based studies: a systematic review. Lancet Neurol. 2009; 8:355–69.


10. Go AS, Mozaffarian D, Roger VL, Benjamin EJ, Berry JD, Blaha MJ, et al. Executive summary: heart disease and stroke statistics: 2014 update: a report from the American Heart Association. Circulation. 2014; 129:399–410.


11. Liberati A, Altman DG, Tetzlaff J, Mulrow C, Gøtzsche PC, Ioannidis JP, et al. The PRISMA statement for reporting systematic reviews and meta-analyses of studies that evaluate health care interventions: explanation and elaboration. J Clin Epidemiol. 2009; 62:e1–34.


12. Bernard GR, Artigas A, Brigham KL, Carlet J, Falke K, Hudson L, et al. The American-European Consensus Conference on ARDS: definitions, mechanisms, relevant outcomes, and clinical trial coordination. Am J Respir Crit Care Med. 1994; 149(3 Pt 1):818–24.


13. ARDS Definition Task Force, Ranieri VM, Rubenfeld GD, Thompson BT, Ferguson ND, Caldwell E, et al. Acute respiratory distress syndrome: the Berlin definition. JAMA. 2012; 307:2526–33.
14. Jennett B, Snoek J, Bond MR, Brooks N. Disability after severe head injury: observations on the use of the Glasgow Outcome Scale. J Neurol Neurosurg Psychiatry. 1981; 44:285–93.


15. Wells GA, Shea B, O’Connell D, Peterson J, Welch V, Losos MP. The Newcastle-Ottawa Scale (NOS) for assessing the quality of nonrandomised studies in meta-analyses. Ottawa: Ottawa Hospital Research Institute;2000.
16. Sidik K, Jonkman JN. A comparison of heterogeneity variance estimators in combining results of studies. Stat Med. 2007; 26:1964–81.


17. IntHout J, Ioannidis JP, Borm GF. The Hartung-Knapp-Sidik-Jonkman method for random effects meta-analysis is straightforward and considerably outperforms the standard DerSimonian-Laird method. BMC Med Res Methodol. 2014; 14:25.


18. Higgins JP, Thompson SG. Quantifying heterogeneity in a meta-analysis. Stat Med. 2002; 21:1539–58.


19. Abecassis IJ, Morton RP, McGrath L, Hanson J, Xue AH, Kelly CM, et al. Respiratory and swallowing outcomes based on aneurysm location in 360 patients with subarachnoid hemorrhage. World Neurosurg. 2017; 105:108–14.


20. Bai W, Li W, Ning YL, Li P, Zhao Y, Yang N, et al. Blood glutamate levels are closely related to acute lung injury and prognosis after stroke. Front Neurol. 2018; 8:755.


21. Gruber A, Reinprecht A, Görzer H, Fridrich P, Czech T, Illievich UM, Richling B. Pulmonary function and radiographic abnormalities related to neurological outcome after aneurysmal subarachnoid hemorrhage. J Neurosurg. 1998; 88:28–37.


22. Hoesch RE, Lin E, Young M, Gottesman RF, Altaweel L, Nyquist PA, et al. Acute lung injury in critical neurological illness. Crit Care Med. 2012; 40:587–93.


23. Kassell NF, Torner JC, Haley EC Jr, Jane JA, Adams HP, Kongable GL. the international cooperative study on the timing of aneurysm surgery. Part 1: overall management results. J Neurosurg. 1990; 73:18–36.
24. Kitamura Y, Nomura M, Shima H, Kuwana N, Kuramitsu T, Chang CC, et al. Acute lung injury associated with systemic inflammatory response syndrome following subarachnoid hemorrhage: a survey by the Shonan Neurosurgical Association. Neurol Med Chir (Tokyo). 2010; 50:456–60.


25. Kramer AH, Bleck TP, Dumont AS, Kassell NF, Olson C, Nathan B. Implications of early versus late bilateral pulmonary infiltrates in patients with aneurysmal subarachnoid hemorrhage. Neurocrit Care. 2009; 10:20–7.


26. Mazeraud A, Robba C, Rebora P, Iaquaniello C, Vargiolu A, Rass V, et al. Acute distress respiratory syndrome after subarachnoid hemorrhage: incidence and impact on the outcome in a large multicenter, retrospective cohort. Neurocrit Care. 2021; 34:1000–8.


27. Naidech AM, Bassin SL, Garg RK, Ault ML, Bendok BR, Batjer HH, et al. Cardiac troponin I and acute lung injury after subarachnoid hemorrhage. Neurocrit Care. 2009; 11:177–82.


28. Unda SR, Labagnara K, Birnbaum J, Wong M, de Silva N, Terala H, et al. Impact of hospital-acquired complications in long-term clinical outcomes after subarachnoid hemorrhage. Clin Neurol Neurosurg. 2020; 194:105945.


29. Wood James H. Cerebral blood flow. In : Carter L Phillip, Spetzler Robert F, Hamilton Mark G, editors. Neurovascular surgery. Ed 1. New York, NY: McGraw-Hill;1995. p. 127–41.
30. Stevens RD, Nyquist PA. The systemic implications of aneurysmal subarachnoid hemorrhage. J Neurol Sci. 2007; 261:143–56.


31. Sarnoff SJ, Sarnoff LC. Neurohemodynamics of pulmonary edema. II. The role of sympathetic pathways in the elevation of pulmonary and systemic vascular pressures following the intracisternal injection of fibrin. Circulation. 1952; 6:51–62.
32. Catapano JS, Ducruet AF, Frisoli FA, Nguyen CL, Louie CE, Labib MA, et al. Predictors of the development of takotsubo cardiomyopathy in aneurysmal subarachnoid hemorrhage and outcomes in patients with intra-aortic balloon pumps. J Neurosurg. 2020; Sep. 4. [Epub]. https://doi.org/10.3171/2020.5.JNS20536.


33. Veeravagu A, Chen YR, Ludwig C, Rincon F, Maltenfort M, Jallo J, et al. Acute lung injury in patients with subarachnoid hemorrhage: a nationwide inpatient sample study. World Neurosurg. 2014; 82:e235–41.


34. Gruber A, Reinprecht A, Illievich UM, Fitzgerald R, Dietrich W, Czech T, et al. Extracerebral organ dysfunction and neurologic outcome after aneurysmal subarachnoid hemorrhage. Crit Care Med. 1999; 27:505–14.


35. Acute Respiratory Distress Syndrome Network, Brower RG, Matthay MA, Morris A, Schoenfeld D, Thompson BT, et al. Ventilation with lower tidal volumes as compared with traditional tidal volumes for acute lung injury and the acute respiratory distress syndrome. N Engl J Med. 2000; 342:1301–8.


36. Amato MB, Barbas CS, Medeiros DM, Magaldi RB, Schettino GP, Lorenzi-Filho G, et al. Effect of a protective-ventilation strategy on mortality in the acute respiratory distress syndrome. N Engl J Med. 1998; 338:347–54.


37. de Haro C, Martin-Loeches I, Torrents E, Artigas A. Acute respiratory distress syndrome: prevention and early recognition. Ann Intensive Care. 2013; 3:11.


38. Caricato A, Conti G, Della Corte F, Mancino A, Santilli F, Sandroni C, et al. Effects of PEEP on the intracranial system of patients with head injury and subarachnoid hemorrhage: the role of respiratory system compliance. J Trauma. 2005; 58:571–6.


39. McGuire G, Crossley D, Richards J, Wong D. Effects of varying levels of positive end-expiratory pressure on intracranial pressure and cerebral perfusion pressure. Crit Care Med. 1997; 25:1059–62.


Fig. 1.
Study flowchart for the literature search and selection of studies. ARDS, acute respiratory distress stress; aSAH, aneurysmal subarachnoid hemorrhage.
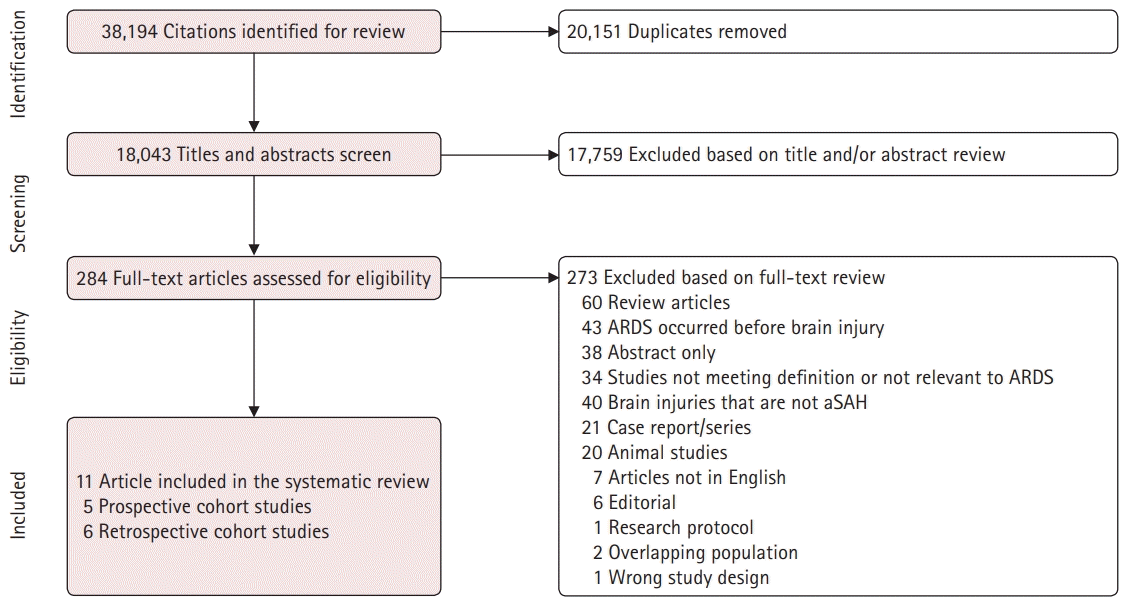
Fig. 2.
Meta-analysis of the prevalence of acute respiratory distress stress among patients with aneurysmal subarachnoid hemorrhage. I2 quantifies the degree of heterogeneity across the studies, and ranges from 0% to 100%. CI, confidence interval.
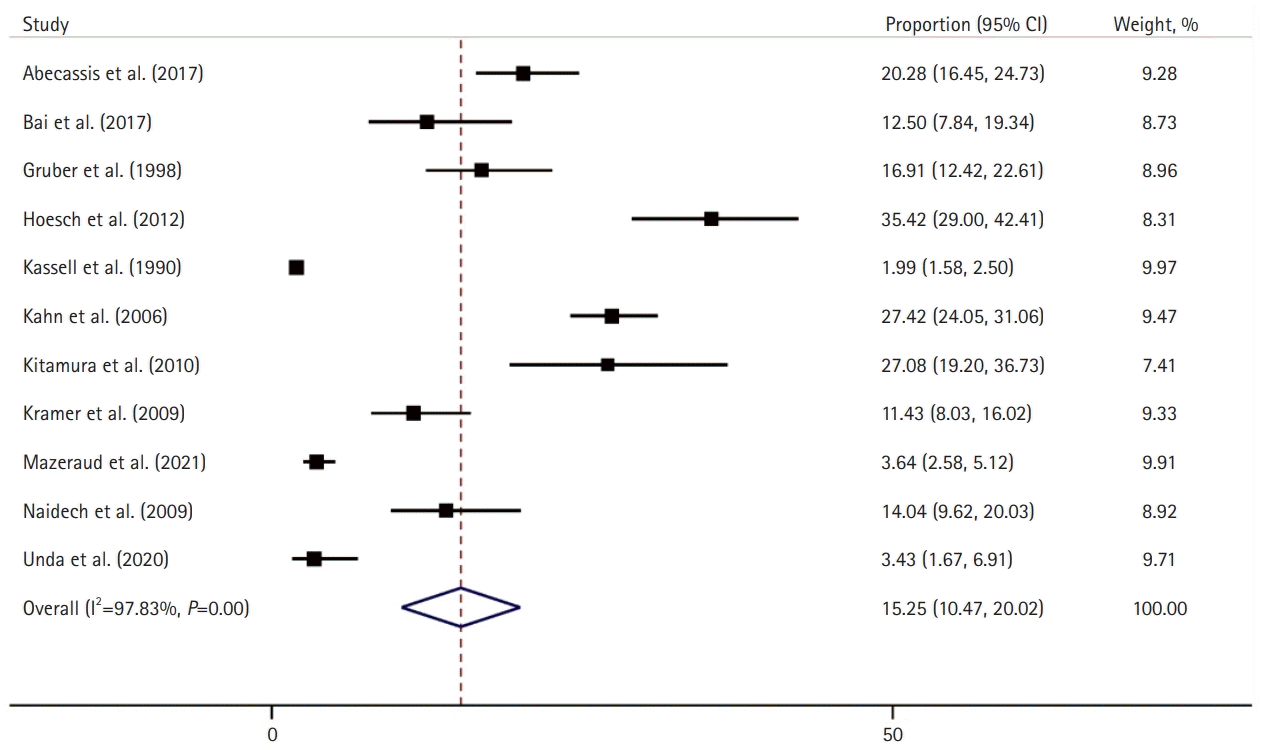
Fig. 3.
Meta-analysis of the time in days from diagnosis of aneurysmal subarachnoid hemorrhage to the onset of acute respiratory distress stress (ARDS). I2 quantifies the degree of heterogeneity across the studies, and ranges from 0% to 100%. CI, confidence interval.
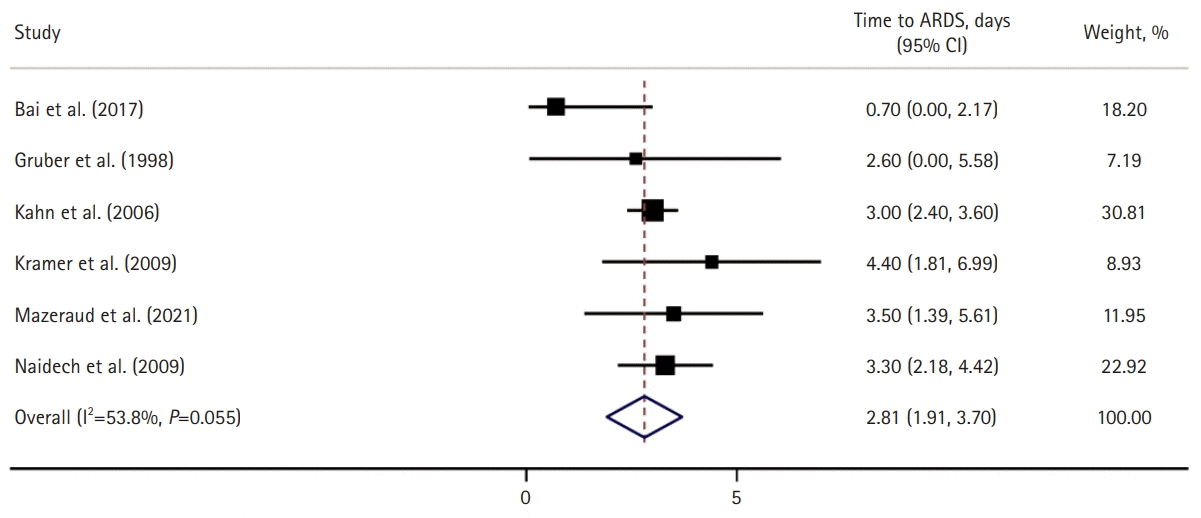