Abstract
Notes
Fund
This study was supported by the National Research Foundation of Korea as subject number ‘NRF-2020R1F1A1071619’.
Ethics Statement
The approval of the institutional ethics committee and informed consents were waived since its nature lies on literature review. Since the consent for publication was not available for the patients mentioned in the figures, patient’s information was anonymized by removing the sex and specific age.
Author Contribution
Concept and design: HJK and HGR. Analysis and interpretation: HJK. Data collection: HJK and HGR. Writing the article: HJK. Critical revision of the article: HJK and HGR. Final approval of the article: HJK and HGR. Statistical analysis: HJK. Obtained funding: HJK. Overall responsibility: HJK and HGR.
REFERENCES









































Fig. 1.
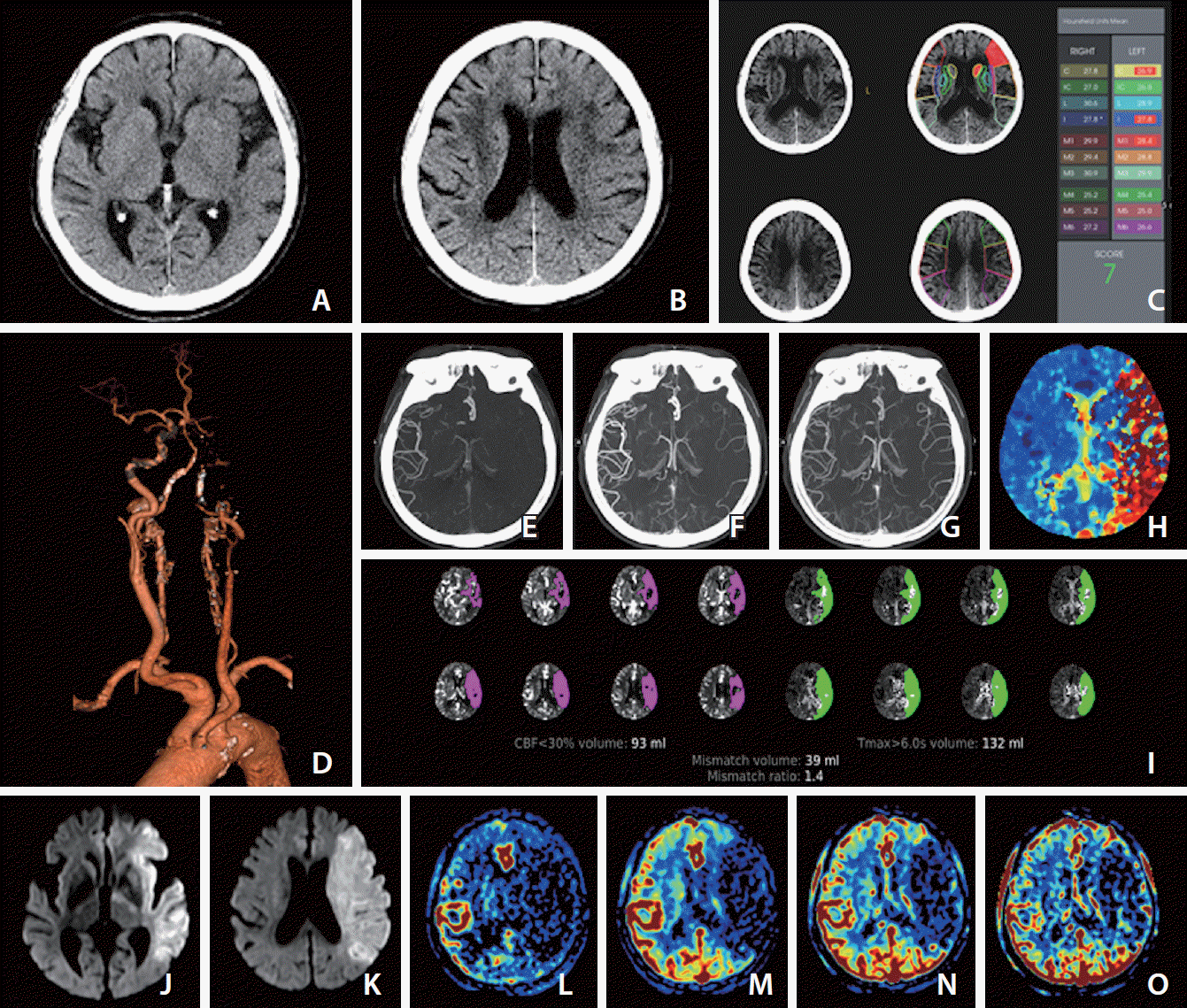
Fig. 2.
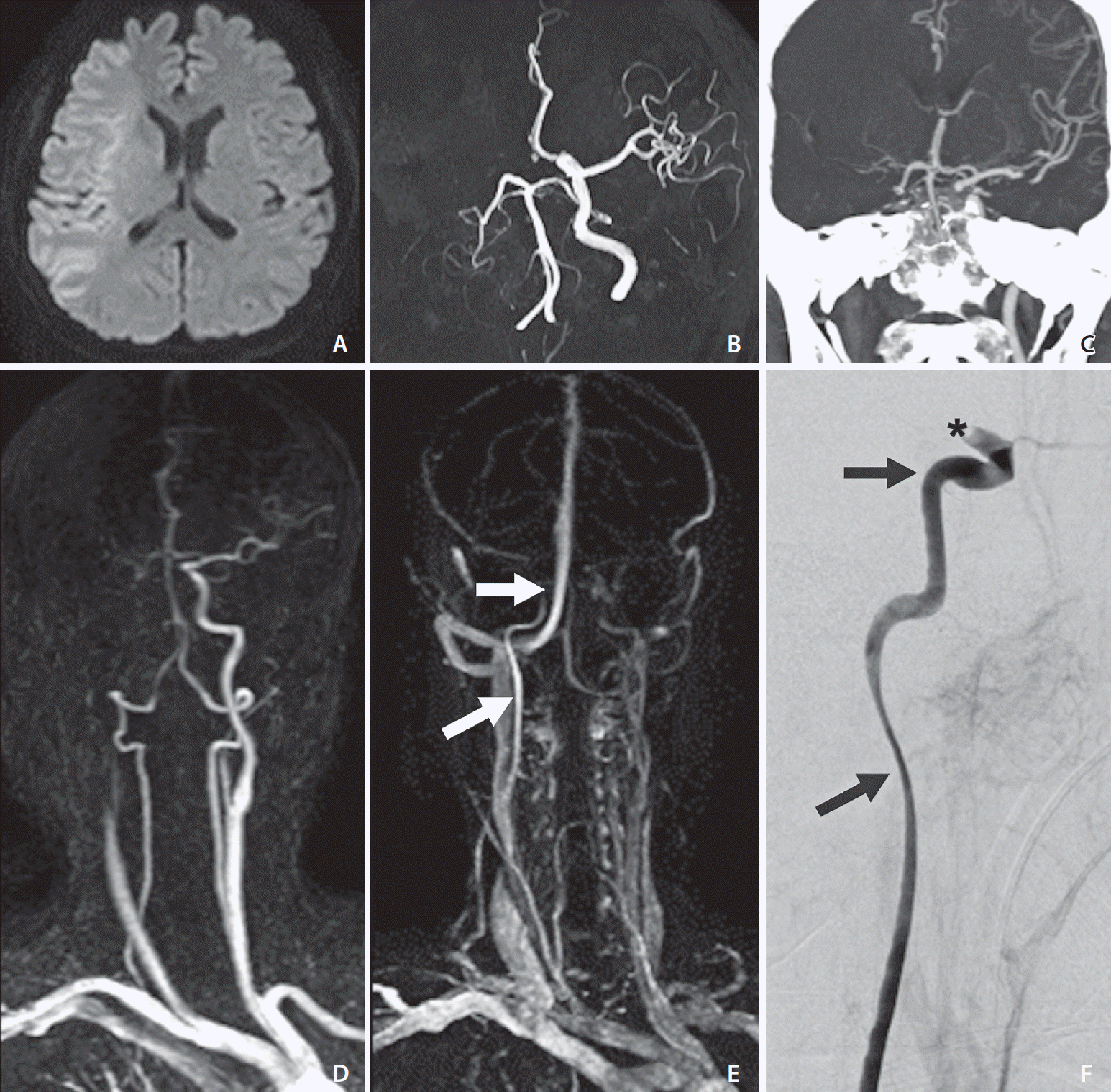
Fig. 3.
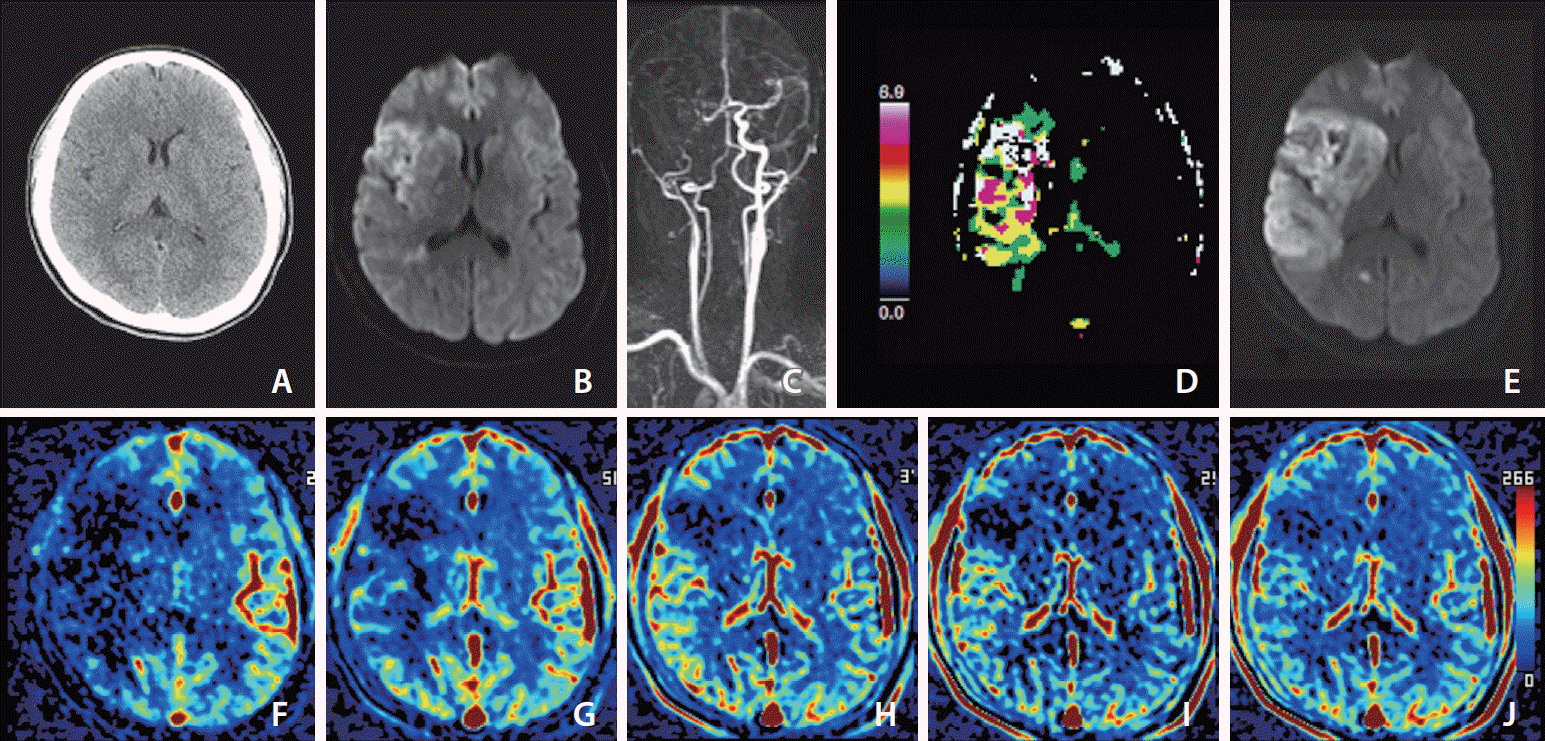
Fig. 4.
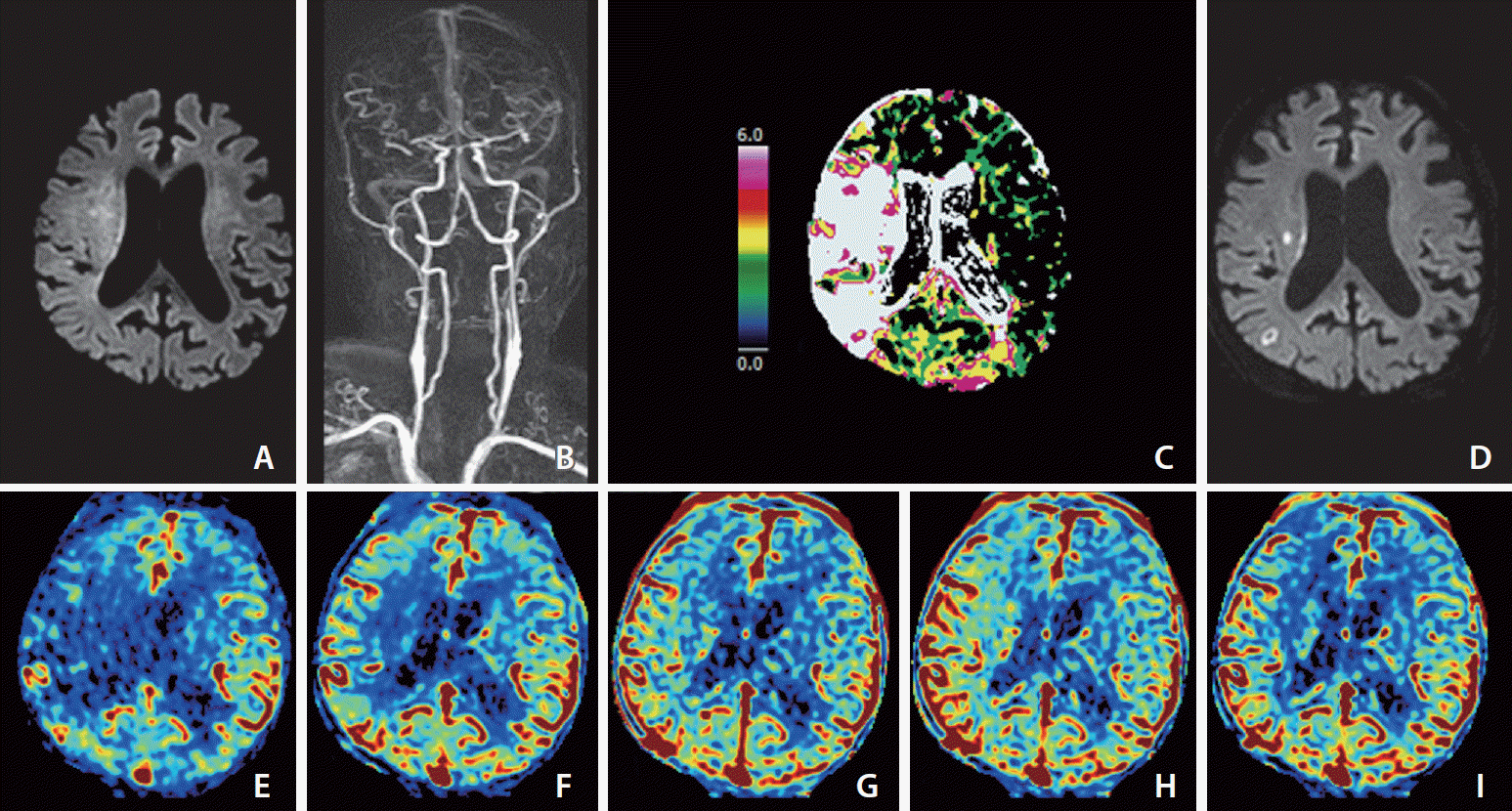
Table 1.
Indications | |
---|---|
Within 3 hours† (COR I) * | • Age ≥18 years |
• NIHSS score ≥6 | |
3 to 4.5 hours† (COR I)* | • Age ≥18 years and ≤80 years |
• Without a history of both diabetes mellitus and prior stroke | |
• NIHSS score ≥6 and ≤25 | |
• Not taking any OACs | |
• Without imaging evidence of ischemic injury involving more than one-third of the MCA territory: IVT is recommended in the setting of early ischemic changes on NCCT of mild to moderate extent (other than frank hypodensity) | |
Wake-up stroke or unclear time of onset >4.5 hours from last known normal state or at baseline state (COR IIa)* | IVT can be beneficial when DWI lesion is smaller than one-third of the MCA territory and no visible signal change on FLAIR |
CMB (COR IIa)* | • Eligible patients who have previously had a small number (1–10) of CMBs demonstrated on MRI, IVT is reasonable |
• Eligible patients who have previously had a high burden of CMBs (>10) demonstrated on MRI, IVT may be associated with an increased risk of symptomatic ICH, and the benefits of treatment are uncertain. IVT may be reasonable if there is the potential for substantial benefit | |
Contraindication | |
• NIHSS score 0–5 (COR III: no benefit)* | |
• NCCT: extensive regions of frank hypodensity (COR III: no benefit)* | |
• Acute ICH (COR III: harm)* | |
• Ischemic stroke within 3 months (COR III: harm)* | |
• Severe head trauma within 3 months (COR III: harm)* | |
• Posttraumatic infarction that occurs during the acute in-hospital phase (COR III: harm)* | |
• Intracranial/intraspinal surgery within 3 months (COR III: harm)* | |
• History of ICH (COR III: harm)* | |
• Symptoms and signs most consistent with a subarachnoid hemorrhage (COR III: harm)* | |
• Gastrointestinal malignancy (COR III: harm)* | |
• Gastrointestinal bleeding within 21 days (COR III: harm)* | |
• Coagulopathy: platelet count <100,000/mm3, INR >1.7, aPTT >40 s, or PT >15 s (COR III: harm)* | |
• Infective endocarditis (COR III: harm)* | |
• Aortic arch dissection (COR III: harm)* | |
• Intra-axial intracranial neoplasm (COR III: harm)* | |
• Full treatment dose of LMWH within the previous 24 hours (COR III: harm)* | |
• Patients taking direct thrombin inhibitors or direct factor Xa inhibitors (COR III: harm)* | |
• Abciximab should not be administered concurrently with IV rt-PA (COR III: harm)* | |
• IV aspirin should not be administered within 90 minutes after the start of IV rt-PA (COR III: harm)* |
AHA, American Heart Association; ASA, American Stroke Association; COR, class of recommendation; MRI, magnetic resonance imaging; rt-PA, recombinant tissue plasminogen activator; IV, intravenous; aPTT, activated partial prothrombin time; CMB, cerebral microbleed; DWI, diffusion-weighted magnetic resonance imaging; FLAIR, fluid-attenuated inversion recovery; ICH, intracranial hemorrhage; INR, international normalized ratio; IVT, intravenous thrombolysis; LMWH, low-molecular-weight heparin; MCA, middle cerebral artery; NCCT, noncontrast computed tomography; NIHSS, National Institutes of Health Stroke Scale; OAC, oral anticoagulant; PT, prothrombin time.
* COR amended to conform with American College of Cardiology/AHA 2015 Recommendation Classification System: COR I (strong), benefit>>>risk; COR IIa (moderate), benefit>>risk; COR IIb (weak), benefit≥risk; COR III (no benefit), benefit=risk; COR III (harm), risk>benefit. [8]
Table 2.
Indications | |||
---|---|---|---|
Within 6 hours† (COR I)* | • Prestroke mRS score of 0 to 1 | ||
• Causative occlusion of the ICA or MCA segment 1 (M1) | |||
• Age ≥18 years | |||
• NIHSS score ≥6 | |||
• ASPECTS of ≥6 | |||
• Treatment can be initiated (groin puncture) within 6 hours of symptom onset | |||
Within 6 hours† (COR IIb)* | • Causative occlusion of the MCA segment 2 (M2) or MCA segment 3 (M3) | ||
• mRS score >1, ASPECTS <6, or NIHSS score <6, and causative occlusion of the ICA or M1 | |||
• Causative occlusion of the anterior cerebral arteries, vertebral arteries, basilar artery, or posterior cerebral arteries | |||
Concomitant with IVT (COR I)* | • Patients eligible for IVT should receive IV rt-PA even if EVT is being considered (COR I)* | ||
• In patients under consideration for EVT, observation after IVT to assess for clinical response should not be performed (COR III: harm)* | |||
6 to 16 hours of last known normal state (COR I)* | • Follow DAWN12 or DEFUSE 313 eligibility criteria | ||
• DAWN eligibility criteria | |||
- 6–24 hours after last known normal state | |||
- Age ≥18 years | |||
- NIHSS score ≥10 | |||
- Prestroke mRS score of 0 or 1 | |||
- Infarct involving <1/3 MCA territory by CT or DWI | |||
- Occlusion of the ICA and/or MCA (M1) | |||
- Mismatch between the severity of the clinical deficit and the infarct volume | |||
A. Age ≥80 years, NIHSS score ≥10 and infarct volume <21 mL | |||
B. Age <80 years, NIHSS score ≥10 and infarct volume <31 mL | |||
C. Age <80 years, NIHSS score ≥20 and infarct volume <51 mL | |||
• DEFUSE 3 eligibility criteria | |||
- 6–16 hours after last known normal state | |||
- Age 18–90 years | |||
- NIHSS score ≥6 | |||
- Prestroke mRS score ≤2 | |||
- Infarct involving <1/3 MCA territory by CT or DWI | |||
- Occlusion of the ICA and/or MCA (M1) | |||
- Imaging criteria | |||
A. Volume of core <70 mL | |||
B. Ratio of volume of penumbra to core >1.8 | |||
C. Volume of penumbra >15 mL | |||
16 to 24 hours of last known normal state (COR IIa)* | • Follow DAWN12 eligibility criteria |
AHA, American Heart Association; ASA, American Stroke Association; EVT, endovascular thrombectomy; COR, class of recommendation; mRS, modified Rankin Scale; rt-PA, recombinant tissue plasminogen activator; IV, intravenous; ASPECTS, Alberta Stroke Program Early CT Score; CT, computed tomography; DWI, diffusion-weighted magnetic resonance imaging; ICA, internal carotid artery; IVT, intravenous thrombolysis; MCA, middle cerebral artery; NIHSS, National Institutes of Health Stroke Scale.
* COR amended to conform with American College of Cardiology/AHA 2015 Recommendation Classification System: COR I (strong), benefit>>>risk; COR IIa (moderate), benefit>>risk; COR IIb (weak), benefit≥risk; COR III (no benefit), benefit=risk; COR III (harm), risk>benefit. [8]
Table 3.
Brain imaging | COR* | New, revised, or unchanged | |
---|---|---|---|
Initial imaging | |||
1. All patients with suspected acute stroke should receive an emergency brain imaging evaluation on first arrival to a hospital before initiating any specific therapy to treat AIS | I | Reworded for clarity from 2013 AIS guidelines† | |
2. Systems should be established so that brain imaging studies can be performed as quickly as possible in patients who may be candidates for IVT or EVT or both | I | Revised from 2013 guidelines† | |
3. NCCT is effective to exclude ICH before IVT | I | Revised from 2013 guidelines† | |
4. MRI is effective to exclude ICH before IVT | I | New recommendation | |
5. CTA with CTP or MRA with DWI with or without MRP is recommended for certain patients | |||
IVT eligibility | |||
1. Administration of rt-PA in eligible patients without first obtaining MRI to exclude CMBs is recommended | I | New recommendation | |
2. In patients eligible for IVT, because the benefit of therapy is time-dependent, treatment should be initiated as quickly as possible and not delayed for additional multimodal neuroimaging, such as CTP and MRP | I | New recommendation | |
3. In patients with AIS who awoke with stroke symptoms or have unclear time of onset >4.5 hours from last known normal state or at baseline state, MRI to identify DWI-positive FLAIR- negative lesions can be useful for selecting those who can benefit from IVT within 4.5 hours of stroke symptom recognition | IIa | New recommendation | |
EVT eligibility-vessel imaging | |||
1. For patients who otherwise meet criteria for EVT, noninvasive vessel imaging of the intracranial arteries is recommended during the initial imaging evaluation | I | Reworded for clarity from 2015 endovascular‡ | |
2. For patients with suspected LVO who have not had noninvasive vessel imaging as part of their initial imaging assessment for stroke, noninvasive vessel imaging should then be obtained as quickly as possible (e.g., during rt-PA infusion if feasible) | I | Revised from 2015 endovascular‡ | |
3. In patients with suspected intracranial LVO and no history of renal impairment, who otherwise meet criteria for EVT, it is reasonable to proceed with CTA if indicated before obtaining a serum creatinine concentration | IIa | New recommendation | |
4. In patients who are potential candidates for EVT, imaging of the extracranial carotid and vertebral arteries, in addition to the intracranial circulation, may be reasonable to provide useful information on patient eligibility and endovascular procedural planning | IIa | New recommendation | |
5. It may be reasonable to incorporate collateral flow status into clinical decision-making in some candidates to determine eligibility for EVT | IIb | Revised from 2015 endovascular‡ | |
EVT eligibility-multimodal imaging | |||
1. When selecting patients with AIS within 6 to 24 hours of last known normal status who have LVO in the anterior circulation, obtaining CTP or DWI, with or without MRP, is recommended to aid in patient selection for EVT, but only when patients meet other eligibility criteria from one of the RCTs that showed benefit from EVT in this extended time window | I | New recommendation | |
2. When evaluating patients with AIS within 6 hours of last known normal status with LVO and an ASPECTS of ≥6, selection for EVT based on CT and CTA or MRI and MRA is recommended compared to performance of additional imaging such as CTP or MRP | I | New recommendation |
AHA, American Heart Association; ASA, American Stroke Association; COR, class of recommendation; rt-PA, recombinant tissue plasminogen activator; AIS, acute ischemic stroke; ASPECTS, Alberta Stroke Program Early CT Score; CMB, cerebral microbleed; CT, computed tomography; CTA, computed tomography angiography; CTP, computed tomography perfusion imaging; DWI, diffusionweighted magnetic resonance imaging; EVT, endovascular thrombectomy; FLAIR, fluid attenuated inversion recovery; ICH, intracranial hemorrhage; IVT, intravenous thrombolysis; LVO, large vessel occlusion; MR, magnetic resonance; MRA, magnetic resonance angiography; MRI, magnetic resonance imaging; MRP, magnetic resonance perfusion imaging; NCCT, noncontrast computed tomography; RCT, randomized clinical trial.
* COR amended to conform with American College of Cardiology/AHA 2015 Recommendation Classification System: COR I (strong), benefit>>>risk; COR IIa (moderate), benefit>>risk; COR IIb (weak), benefit≥risk; COR III (no benefit), benefit=risk; COR III (harm), risk>benefit. [8]