Abstract
Spatial insight into intracranial pathology and structure is important for neurosurgeons to perform safe and successful surgeries. Three-dimensional (3D) printing technology in the medical field has made it possible to produce intuitive models that can help with spatial perception. Recent advances in 3D-printed disease models have removed barriers to entering the clinical field and medical market, such as precision and texture reality, speed of production, and cost. The 3D-printed disease model is now ready to be actively applied to daily clinical practice in neurosurgical planning, simulation, and training. In this review, the development of 3D-printed neurosurgical disease models and their application are summarized and discussed.
Spatial perception of brain structures is a key attribute of competent neurosurgeons. Predicting the location of the lesion in three-dimensional (3D) space before surgery is more important for neurosurgeons than other surgeons because brain lesions have to be approached through a difficult process called craniotomy, and it is almost impossible to search for lesions during surgery by recklessly scouring brain tissue. In addition, all anatomical information of neurosurgical patients is obtained from sophisticated neuroimaging modalities, such as computed tomography (CT) or magnetic resonance imaging, which are usually presented in two-dimensional images of each axis. To succeed in brain surgery in a safe and efficient way, neurosurgeons must spatially reconstruct and simulate brain structures and lesions in their head based on neuroimages. This ability of spatial perception of brain structures can be acquired through repeated training of image interpretation and virtual reconstruction, followed by real-world verification at the surgical site of the patient. However, it is difficult for trainees to be given sufficient opportunities to practice in such a harsh recent training environment. Traditionally, surgical training using cadaver dissection has been preferred to compensate for the lack of clinical experience, but even this is becoming increasingly difficult due to problems with the supply and demand of cadavers as well as ethical and biosafety issues.
Recent advancements in 3D printing technology in medicine provide an opportunity to alleviate these difficulties. The medical 3D printing field can be divided into four main fields [60] : 1) anatomical models for surgical planning, simulation, and training; 2) medical devices including bioimplants, orthoses/prostheses, and surgical instruments including guide templates; 3) tissue engineering devices or bioprinting organs/tissue models; and 4) drug formulations. However, the scope of application of 3D printing technology in the medical field is growing rapidly to novel applications. In this review, the focus will be on the current development and application of 3D-printed disease models for surgical planning, simulation, and training in the neurosurgical field.
The origin of the current 3D printing technology concept dates back to the early 1980s, allowing the manufacturing of objects by depositing materials layer by layer using a stereolithography apparatus (SLA) [44]. The first reported human anatomical model using an SLA was a skull model constructed from CT images in 1990 [67]. However, it was in the early 2000s that 3D printing technology began to be applied to various medical fields [47]. Thanks to technical advances in 3D printing methods, diversification of source materials, and easy availability of hardware, an appropriate cost-effectiveness of 3D printing have been achieved, and public interest exploded in the 2010s.
In the field of neurosurgery, early efforts to create 3D-printed disease models for surgical planning or educational purposes were attempted in spinal disease [19] and cerebral aneurysm [20]. Approximately 150 papers covering neurosurgical disease models built for surgical planning, simulation and training have been published in the English literature to date (Fig. 1). Most of the models were developed for the purpose of presurgical simulation of complex and difficult cases, or education/training of surgical techniques and anatomy. The three major limitations of the early 3D-printed disease models were precision and texture reality, speed of production, and cost acceptability [81]. However, with the continued development of technology, these limitations have been resolved, and barriers to entering the clinical field and medical market have almost been completely removed.
From a developer's point of view, the 3D-printed neurosurgical disease model can be divided into two categories. One is a patient-specific model, and the other is a standardized model (Table 1). The patient-specific model is customized one for the specific case, which is usually a complex, rare and challenging disease form to be treated surgically. The purpose of the patient-specific model is to improve the clinical outcomes by enabling preoperative surgical planning and simulation as well as by aiding communication. The production of patient-specific models must be completed in a short time with a single order, and its production pipeline must be maintained to continuously handle multiple orders. High-risk surgical diseases, such as deep-seated brain tumors and complex cerebrovascular or spinal diseases, are expected to be frequent indications for patient-specific models. The representative patient-specific 3D-printed disease models are shown in Fig. 2. On the other hand, the standardized model is developed according to an event or educational program, and then copies are produced and supplied. Therefore, it is not necessary to continuously maintain the production pipeline of the model if mass production is performed at once. Moreover, the standardized model can lower the price compared to the patient-specific model due to cost reduction by mass production Since the purpose is training and education, the target disease condition for standardized models is usually a representative and general. The standardized model is expected to be utilized in the fields of skull base surgery, endoscopic surgery, endovascular surgery, spinal instrumentation, and functional neurosurgery, where a hands-on workshop or cadaver dissection course is actively held.
Spinal disease is the most active field in neurosurgery in which 3D printing technology is vigorously applied [28]. In 1999, the first 3D-printed spinal disease model using an SLA was introduced for the surgical planning and simulation of complex spinal disease [19]. Since then, more than 50 papers have repeatedly confirmed the benefit of 3D-printed spinal disease models in the planning and execution of surgery by providing insights into optimum screw trajectories, appropriate instrument or implant selection, detailed information on anatomical structures, geometrical measurement of deformity degrees, and establishment of surgical goals. Moreover, several studies have demonstrated a reductions in operation time and perioperative blood loss by using 3D-printed spinal disease models [29,59,111,114]. The material used to manufacture spinal disease models continued to be developed through various attempts starting from plastic, so that the texture can be similar to the actual spine bone [19,39,89,96]. The model extends its scope to physiological or flexible models [14,108]. The majority of 3D-printed spinal disease models have been patient-specific models applied in real clinical practice to improve clinical outcomes. Similar to other 3D-printed disease models, 3D-printed spinal disease models have been shown to improve patient communication and education [48,119,120]. Patient-specific templates for the guidance of instrumentation are another active field of the application of 3D printing technology in spine surgery. However, a standardized model of spinal disease has been actively developed relatively recently and is well utilized in training and education [5-7,9,15,16,38,79,110].
The earliest and most common target disease for the 3D-printed cerebrovascular disease model is intracranial aneurysm [1,4,20,25,27,49,50,57,90,97,112]. The texture and elasticity of the developed aneurysm model were realistic enough to simulate surgery [2,55,58,69,84]. The 3D-printed aneurysm model was developed not only for conventional surgical planning but also for endovascular treatment [53,74]. Another major target disease for 3D-printed cerebrovascular disease models is arteriovenous malformations, which may benefit from reduced operation time by helping presurgical planning [20,86,93,106,115]. Other cerebrovascular models, such as dural venous sinus or cavernous angioma models, were also attempted [34,95]. For efficient presurgical planning, all models that include adjacent structures as well as vascular lesions have been shown to be more useful for surgical planning than regional models constructed with only vascular structures [2,98]. The 3D-printed cerebrovascular disease model is also actively used for simulation experiments. One good example is cerebrovascular stenosis or occlusion models, which have successfully demonstrated meaningful results in a variety of experimental settings [11-13,23,65,70,113]. The standardized model of cerebrovascular disease for educational purposes has been attempted from the relatively early stage of development [24,46,56,73,99,103]. From an educational standpoint, it has been reported that virtual reality models are superior to 3D-printed models in terms of resolution, zoom capability, and model durability, but 3D-printed models have significant advantages in depth perception and ease of manipulation compared to virtual reality models [3].
The 3D-printed brain tumor model is a latecomer compared to spinal or cerebrovascular disease models. This is because brain tumors are less stereotypical and individual variances in tumor location, size, and texture are so great that only case-by-case experiences are meaningful. Therefore, the fabrication process for individualized complex patient-specific models has been less cost-effective to readily enter clinical practice. However, it is obvious that presurgical planning using 3D-printed brain tumor models can help ensure safe and successful surgeries especially for less experienced neurosurgeons [76,78]. The cost-effectiveness limit of the customized model is gradually being solved with the development of technology [21,88]. The patient-specific 3D-printed brain tumor model production platform that can cope with the speed of urgent clinical practice has been developed and is now ready to use [22]. The use of multiple and transparent materials for the realization of complex structures and the brain has enabled much more realistic and useful results for 3D-printed brain tumor models [22,36,51,102,117]. A functional navigator approach that applies tractography results to a 3D-printed brain tumor model has also been attempted [30,33,94].
On the other hand, skull base surgery is the most favorable field for both patient-specific and standardized 3D-printed disease models [37,62,82]. As many skull base surgery workshops are being held worldwide, skull base surgery is a field where standardized models are actively produced and applied for education and training [63,103]. The development of a model capable of drilling bones such as real bone plays a significant role in invigoration [52,54,77]. The 3D-printed skull base tumor model has been actively used for endoscopic skull base surgery training as well, where the keyhole anatomy is a steep barrier of the learning curve [42,64,71,75,87,92,101,109,118]. Moreover, evidence has shown that its application to endoscopic brain tumor surgery enhances surgical outcomes by aiding in reconstruction or by reducing the operation time, blood loss, and complication rate [26,43].
Surgical planning for reconstructive cranioplasty after craniofacial resection, skull anomaly correction, and tumor resection can be greatly supported by 3D-printed models [18,31,40,72]. Endoscopic surgery for ventricular lesions, including ventriculostomy, is another popular subject of training programs using 3D-printed models [8,32,41,61,85,91,104,107]. The patient-specific 3D-printed myelomeningocele model was fabricated to plan the correction of spinal deformity [45]. Models of other types of congenital anomalies, such as meningoencephalocele model, were also created for surgical planning [17]. The 3D-printed model could be helpful for repairing cerebrospinal fluid leakage sites by designing an appropriate flap to cover [35]. The quality assurance of radiosurgery could be tested by a 3D-printed patient-specific phantom [66]. More realistic models with special effects have been developed for training various neurosurgical skills [10,68,80,107,116].
Despite the rapid development and popularization of 3D printing technology, there is still no consensus on its usefulness for neurosurgical planning, simulation, and training in the clinical field. The clinical entry of 3D printing technology is strictly regulated by medical administrative authorities of each country and only partially allowed officially for minor subjects. This is because there is a lack of large-scale studies that can prove its clinical effectiveness expressed by general clinical variables, which is practically difficult to implement because it targets complex and uncommon cases. However, the real value of the 3D-printed disease model is to lower the entry barrier for high-level surgery by allowing neurosurgeons with less experience to perform difficult surgeries more safely and successfully. This is more important in the current training environment with limited opportunities. Continuing efforts to shorten the learning curve for high-level neurosurgeries using a 3D-printed model will also help resolve the trend of medical care concentration in high-volume hospitals.
Th increasing affordability and convenience of hardware have fueled advances in biotechnological 3D printing. Their application in daily clinical practice in neurosurgery for the purpose of presurgical planning, simulation and training is now feasible [22,83]. The accuracy of the full-scale model is comparable to that of a navigation system [100,105]. The 3D-printed disease model is expected to play mediating role between the current conventional navigation system and the future augmented reality-based navigation system. The clinical experience accumulated with the 3D-printed disease model will be a valuable basis for the development of futuristic navigation systems.
Notes
Conflicts of interest
Chul-Kee Park has been editorial board of JKNS since September 2020. He was not involved in the review process of this original article. No potential conflict of interest relevant to this article was reported.
ACKNOWLEDGMENTS
This work was supported by a Korea Medical Device Development Fund Grant funded by the Korean government (Ministry of Science and ICT; Ministry of Trade, Industry and Energy; Ministry of Health & Welfare; and Ministry of Food and Drug Safety) (project number : 202012E08). The photos of the 3D-printed disease model used in this paper were kindly provided by Pfs. In Sup Choi (Incheon Sejong Hospital, Incheon, Korea) and Sang Joon Park (MEDICALIP. Co. Ltd).
References
1. Andereggen L, Gralla J, Andres RH, Weber S, Schroth G, Beck J, et al. Stereolithographic models in the interdisciplinary planning of treatment for complex intracranial aneurysms. Acta Neurochir (Wien). 158:1711–1720. 2016.


2. Bae JW, Lee DY, Pang CH, Kim JE, Park CK, Lee D, et al. Clinical application of 3D virtual and printed models for cerebrovascular diseases. Clin Neurol Neurosurg. 206:106719. 2021.


3. Bairamian D, Liu S, Eftekhar B. Virtual reality angiogram vs 3-dimensional printed angiogram as an educational tool-a comparative study. Neurosurgery. 85:E343–E349. 2019.


4. Blaszczyk M, Jabbar R, Szmyd B, Radek M. 3D printing of rapid, low-cost and patient-specific models of brain vasculature for use in preoperative planning in clipping of intracranial aneurysms. J Clin Med. 10:1201. 2021.


5. Bohl MA, Mauria R, Zhou JJ, Mooney MA, DiDomenico JD, McBryan S, et al. The barrow biomimetic spine: face, content, and construct validity of a 3D-printed spine model for freehand and minimally invasive pedicle screw insertion. Global Spine J. 9:635–641. 2019.


6. Bortman J, Baribeau Y, Jeganathan J, Amador Y, Mahmood F, Shnider M, et al. Improving clinical proficiency using a 3-dimensionally printed and patient-specific thoracic spine model as a haptic task trainer. Reg Anesth Pain Med. 43:819–824. 2018.


7. Bow H, Zuckerman SL, Griffith B, Lewis S, McGruder C, Pruthi S, et al. A 3D-printed simulator and teaching module for placing S2-alar iliac screws. Oper Neurosurg (Hagerstown). 18:339–346. 2020.


8. Breimer GE, Haji FA, Bodani V, Cunningham MS, Lopez-Rios AL, Okrainec A, et al. Simulation-based education for endoscopic third ventriculostomy: a comparison between virtual and physical training models. Oper Neurosurg (Hagerstown). 13:89–95. 2017.


9. Burkhard M, Fürnstahl P, Farshad M. Three-dimensionally printed vertebrae with different bone densities for surgical training. Eur Spine J. 28:798–806. 2019.


10. Byvaltsev V, Polkin R, Bereznyak D, Giers MB, Hernandez PA, Shepelev V, et al. 3D-printed cranial models simulating operative field depth for microvascular training in neurosurgery. Surg Neurol Int. 12:213. 2021.


11. Chueh JY, Kühn AL, Puri AS, Wilson SD, Wakhloo AK, Gounis MJ. Reduction in distal emboli with proximal flow control during mechanical thrombectomy: a quantitative in vitro study. Stroke. 44:1396–1401. 2013.


12. Chueh JY, Puri AS, Wakhloo AK, Gounis MJ. Risk of distal embolization with stent retriever thrombectomy and ADAPT. J Neurointerv Surg. 8:197–202. 2016.


13. Chueh JY, Wakhloo AK, Gounis MJ. Effectiveness of mechanical endovascular thrombectomy in a model system of cerebrovascular occlusion. AJNR Am J Neuroradiol. 33:1998–2003. 2012.


14. Clifton W, Damon A, Soares C, Nottmeier E, Pichelmann M. Investigation of a three-dimensional printed dynamic cervical spine model for anatomy and physiology education. Clin Anat. 34:30–39. 2021.


15. Clifton W, Damon A, Stein R, Pichelmann M, Nottmeier E. Biomimetic 3-dimensional-printed posterior cervical laminectomy and fusion simulation: advancements in education tools for trainee instruction. World Neurosurg. 135:308. 2020.


16. Clifton W, Nottmeier E, Edwards S, Damon A, Dove C, Refaey K, et al. Development of a novel 3D printed phantom for teaching neurosurgical trainees the freehand technique of C2 laminar screw placement. World Neurosurg. 129:e812–e820. 2019.


17. Coelho G, Chaves TMF, Goes AF, Del Massa EC, Moraes O, Yoshida M. Multimaterial 3D printing preoperative planning for frontoethmoidal meningoencephalocele surgery. Childs Nerv Syst. 34:749–756. 2018.


18. Coelho G, Warf B, Lyra M, Zanon N. Anatomical pediatric model for craniosynostosis surgical training. Childs Nerv Syst. 30:2009–2014. 2014.


19. D’Urso PS, Askin G, Earwaker JS, Merry GS, Thompson RG, Barker TM, et al. Spinal biomodeling. Spine (Phila Pa 1976). 24:1247–1251. 1999.
20. D’Urso PS, Thompson RG, Atkinson RL, Weidmann MJ, Redmond MJ, Hall BI, et al. Cerebrovascular biomodelling: a technical note. Surg Neurol. 52:490–500. 1999.


21. Damon A, Clifton W, Valero-Moreno F, Quinones-Hinojosa A. Cost-effective method for 3-dimensional printing dynamic multiobject and patient-specific brain tumor models: technical note. World Neurosurg. 140:173–179. 2020.


22. Dho YS, Lee D, Ha T, Ji SY, Kim KM, Kang H, et al. Clinical application of patient-specific 3D printing brain tumor model production system for neurosurgery. Sci Rep. 11:7005. 2021.


23. Dholakia RJ, Kappel AD, Pagano A, Woo HH, Lieber BB, Fiorella DJ, et al. In vitro angiographic comparison of the flow-diversion performance of five neurovascular stents. Interv Neuroradiol. 24:150–161. 2018.


24. Dong M, Chen G, Li J, Qin K, Ding X, Peng C, et al. Three-dimensional brain arteriovenous malformation models for clinical use and resident training. Medicine (Baltimore). 97:e9516. 2018.


25. Erbano BO, Opolski AC, Olandoski M, Foggiatto JA, Kubrusly LF, Dietz UA, et al. Rapid prototyping of three-dimensional biomodels as an adjuvant in the surgical planning for intracranial aneurysms. Acta Cir Bras. 28:756–761. 2013.


26. Essayed WI, Unadkat P, Hosny A, Frisken S, Rassi MS, Mukundan S, et al. 3D printing and intraoperative neuronavigation tailoring for skull base reconstruction after extended endoscopic endonasal surgery: proof of concept. J Neurosurg. 130:248–255. 2018.


27. Faraj MK, Hoz SS, Mohammad AJ. The use of three-dimensional anatomical patient-specific printed models in surgical clipping of intracranial aneurysm: a pilot study. Surg Neurol Int. 11:381. 2020.


28. Fiani B, Newhouse A, Cathel A, Sarhadi K, Soula M. Implications of 3-dimensional printed spinal implants on the outcomes in spine surgery. J Korean Neurosurg Soc. 64:495–504. 2021.


29. Galvez M, Asahi T, Baar A, Carcuro G, Cuchacovich N, Fuentes JA, et al. Use of three-dimensional printing in orthopaedic surgical planning. J Am Acad Orthop Surg Glob Res Rev. 2:e071. 2018.


30. Gargiulo P, Árnadóttir Í, Gíslason M, Edmunds K, Ólafsson I. New directions in 3D medical modeling: 3D-printing anatomy and functions in neurosurgical planning. J Healthc Eng. 2017:1439643. 2017.


31. Ghizoni E, De Souza JPSAS, Raposo-Amaral CE, Denadai R, De Aquino HB, Raposo-Amaral CA, et al. 3D-printed craniosynostosis model: new simulation surgical tool. World Neurosurg. 109:356–361. 2018.


32. Gholampour S, Bahmani M. Hydrodynamic comparison of shunt and endoscopic third ventriculostomy in adult hydrocephalus using in vitro models and fluid-structure interaction simulation. Comput Methods Programs Biomed. 204:106049. 2021.


33. Gomez-Feria J, Narros JL, Ciriza GG, Roldan-Lora F, Schrader IM, Martin-Rodríguez JF, et al. 3D printing of diffuse low-grade gliomas involving eloquent cortical areas and subcortical functional pathways: technical note. World Neurosurg. 147:164–171. 2021.


34. Govsa F, Karakas AB, Ozer MA, Eraslan C. Development of life-size patient-specific 3D-printed dural venous models for preoperative planning. World Neurosurg. 110:e141–e149. 2018.


35. Grau S, Kellermann S, Faust M, Perrech M, Beutner D, Drzezga A, et al. Repair of cerebrospinal fluid leakage using a transfrontal, radial adipofascial flap: an individual approach supported by three-dimensional printing for surgical planning. World Neurosurg. 110:315–318. 2018.


36. Grosch AS, Schröder T, Schröder T, Onken J, Picht T. Development and initial evaluation of a novel simulation model for comprehensive brain tumor surgery training. Acta Neurochir (Wien). 162:1957–1965. 2020.


37. Guo XY, He ZQ, Duan H, Lin FH, Zhang GH, Zhang XH, et al. The utility of 3-dimensional-printed models for skull base meningioma surgery. Ann Transl Med. 8:370. 2020.


38. Han M, Portnova AA, Lester M, Johnson M. A do-it-yourself 3D-printed thoracic spine model for anesthesia resident simulation. PLoS One. 15:e0228665. 2020.


39. Hao J, Nangunoori R, Wu YY, Rajaraman M, Cook D, Yu A, et al. Material characterization and selection for 3D-printed spine models. 3D Print Med. 4:8. 2018.


40. Hicdonmez T, Parsak T, Cobanoglu S. Simulation of surgery for craniosynostosis: a training model in a fresh cadaveric sheep cranium. Technical note. J Neurosurg. 105(2 Suppl):150–152. 2006.


41. Hooten KG, Lister JR, Lombard G, Lizdas DE, Lampotang S, Rajon DA, et al. Mixed reality ventriculostomy simulation: experience in neurosurgical residency. Neurosurgery 10 Suppl. 4:576–581. 2014.
42. Hsieh TY, Cervenka B, Dedhia R, Strong EB, Steele T. Assessment of a patient-specific, 3-dimensionally printed endoscopic sinus and skull base surgical model. JAMA Otolaryngol Head Neck Surg. 144:574–579. 2018.


43. Huang X, Liu Z, Wang X, Li XD, Cheng K, Zhou Y, et al. A small 3D-printing model of macroadenomas for endoscopic endonasal surgery. Pituitary. 22:46–53. 2019.


44. Hull CW. The birth of 3D printing. Res Technol Manag. 58:25–30. 2015.
45. Karlin L, Weinstock P, Hedequist D, Prabhu SP. The surgical treatment of spinal deformity in children with myelomeningocele: the role of personalized three-dimensional printed models. J Pediatr Orthop B. 26:375–382. 2017.


46. Khan IS, Kelly PD, Singer RJ. Prototyping of cerebral vasculature physical models. Surg Neurol Int. 5:11. 2014.


47. Kim GB, Lee S, Kim H, Yang DH, Kim YH, Kyung YS, et al. Three-dimensional printing: basic principles and applications in medicine and radiology. Korean J Radiol. 17:182–197. 2016.


48. Kim MP, Ta AH, Ellsworth WA 4th, Marco RA, Gaur P, Miller JS. Three dimensional model for surgical planning in resection of thoracic tumors. Int J Surg Case Rep. 16:127–129. 2015.


49. Kim PS, Choi CH, Han IH, Lee JH, Choi HJ, Lee JI. Obtaining informed consent using patient specific 3D printing cerebral aneurysm model. J Korean Neurosurg Soc. 62:398–404. 2019.


50. Kimura T, Morita A, Nishimura K, Aiyama H, Itoh H, Fukaya S, et al. Simulation of and training for cerebral aneurysm clipping with 3-dimensional models. Neurosurgery. 65:719–725. 2009.


51. Kondo K, Harada N, Masuda H, Sugo N, Terazono S, Okonogi S, et al. A neurosurgical simulation of skull base tumors using a 3D printed rapid prototyping model containing mesh structures. Acta Neurochir (Wien). 158:1213–1219. 2016.


52. Kondo K, Nemoto M, Harada N, Masuda H, Ando S, Kubota S, et al. Three-dimensional printed model for surgical simulation of combined transpetrosal approach. World Neurosurg. 127:e609–e616. 2019.


53. Kono K, Shintani A, Okada H, Terada T. Preoperative simulations of endovascular treatment for a cerebral aneurysm using a patient-specific vascular silicone model. Neurol Med Chir (Tokyo). 53:347–351. 2013.


54. Kosterhon M, Neufurth M, Neulen A, Schäfer L, Conrad J, Kantelhardt SR, et al. Multicolor 3D printing of complex intracranial tumors in neurosurgery. J Vis Exp. 2020; [Epub ahead of print].


55. Lan Q, Chen A, Zhang T, Li G, Zhu Q, Fan X, et al. Development of three-dimensional printed craniocerebral models for simulated neurosurgery. World Neurosurg. 91:434–442. 2016.


56. Lan Q, Zhu Q, Xu L, Xu T. Application of 3D-printed craniocerebral model in simulated surgery for complex intracranial lesions. World Neurosurg. 134:e761–e770. 2020.


57. Leal A, Souza M, Nohama P. Additive manufacturing of 3D biomodels as adjuvant in intracranial aneurysm clipping. Artif Organs. 43:E9–E15. 2019.


58. Leal AG, Mori YT, Nohama P, De Souza MA. Three-dimensional hollow elastic models for intracranial aneurysm clipping election - a case study. Annu Int Conf IEEE Eng Med Biol Soc. 2019:4137–4140. 2019.


59. Li C, Yang M, Xie Y, Chen Z, Wang C, Bai Y, et al. Application of the polystyrene model made by 3-D printing rapid prototyping technology for operation planning in revision lumbar discectomy. J Orthop Sci. 20:475–480. 2015.


60. Liaw CY, Guvendiren M. Current and emerging applications of 3D printing in medicine. Biofabrication. 9:024102. 2017.


61. Licci M, Thieringer FM, Guzman R, Soleman J. Development and validation of a synthetic 3D-printed simulator for training in neuroendoscopic ventricular lesion removal. Neurosurg Focus. 48:E18. 2020.


62. Lin J, Zhou Z, Guan J, Zhu Y, Liu Y, Yang Z, et al. Using three dimensional printing to create individualized cranial nerve models for skull base tumor surgery. World Neurosurg. 120:e142–e152. 2018.
63. Lin QS, Lin YX, Wu XY, Yao PS, Chen P, Kang DZ. Utility of 3-dimensional-printed models in enhancing the learning curve of surgery of tuberculum sellae meningioma. World Neurosurg. 113:e222–e231. 2018.


64. London NR Jr, Rangel GG, VanKoevering K, Zhang A, Powell AR, Prevedello DM, et al. Simulation of pediatric anterior skull base anatomy using a 3D printed model. World Neurosurg. 147:e405–e410. 2021.


65. Machi P, Jourdan F, Ambard D, Reynaud C, Lobotesis K, Sanchez M, et al. Experimental evaluation of stent retrievers’ mechanical properties and effectiveness. J Neurointerv Surg. 9:257–263. 2017.


66. Makris DN, Pappas EP, Zoros E, Papanikolaou N, Saenz DL, Kalaitzakis G, et al. Characterization of a novel 3D printed patient specific phantom for quality assurance in cranial stereotactic radiosurgery applications. Phys Med Biol. 64:105009. 2019.


67. Mankovich NJ, Cheeseman AM, Stoker NG. The display of three-dimensional anatomy with stereolithographic models. J Digit Imaging. 3:200–203. 1990.


68. Mashiko T, Konno T, Kaneko N, Watanabe E. Training in brain retraction using a self-made three-dimensional model. World Neurosurg. 84:585–590. 2015.


69. Mashiko T, Otani K, Kawano R, Konno T, Kaneko N, Ito Y, et al. Development of three-dimensional hollow elastic model for cerebral aneurysm clipping simulation enabling rapid and low cost prototyping. World Neurosurg. 83:351–361. 2015.


70. Mokin M, Setlur Nagesh SV, Ionita CN, Mocco J, Siddiqui AH. Stent retriever thrombectomy with the cover accessory device versus proximal protection with a balloon guide catheter: in vitro stroke model comparison. J Neurointerv Surg. 8:413–417. 2016.


71. Mooney MA, Cavallo C, Zhou JJ, Bohl MA, Belykh E, Gandhi S, et al. Three-dimensional printed models for lateral skull base surgical training: anatomy and simulation of the transtemporal approaches. Oper Neurosurg (Hagerstown). 18:193–201. 2020.


72. Müller A, Krishnan KG, Uhl E, Mast G. The application of rapid prototyping techniques in cranial reconstruction and preoperative planning in neurosurgery. J Craniofac Surg. 14:899–914. 2003.


73. Nagassa RG, McMenamin PG, Adams JW, Quayle MR, Rosenfeld JV. Advanced 3D printed model of middle cerebral artery aneurysms for neurosurgery simulation. 3D Print Med. 5:11. 2019.


74. Namba K, Higaki A, Kaneko N, Mashiko T, Nemoto S, Watanabe E. Microcatheter shaping for intracranial aneurysm coiling using the 3-dimensional printing rapid prototyping technology: preliminary result in the first 10 consecutive cases. World Neurosurg. 84:178–186. 2015.


75. Narayanan V, Narayanan P, Rajagopalan R, Karuppiah R, Rahman ZA, Wormald PJ, et al. Endoscopic skull base training using 3D printed models with pre-existing pathology. Eur Arch Otorhinolaryngol. 272:753–757. 2015.


76. Oishi M, Fukuda M, Yajima N, Yoshida K, Takahashi M, Hiraishi T, et al. Interactive presurgical simulation applying advanced 3D imaging and modeling techniques for skull base and deep tumors. J Neurosurg. 119:94–105. 2013.


77. Okonogi S, Kondo K, Harada N, Masuda H, Nemoto M, Sugo N. Operative simulation of anterior clinoidectomy using a rapid prototyping model molded by a three-dimensional printer. Acta Neurochir (Wien). 159:1619–1626. 2017.


78. Panesar SS, Magnetta M, Mukherjee D, Abhinav K, Branstetter BF, Gardner PA, et al. Patient-specific 3-dimensionally printed models for neurosurgical planning and education. Neurosurg Focus. 47:E12. 2019.


79. Park HJ, Wang C, Choi KH, Kim HN. Use of a life-size three-dimensional-printed spine model for pedicle screw instrumentation training. J Orthop Surg Res. 13:86. 2018.


80. Ploch CC, Mansi CSSA, Jayamohan J, Kuhl E. Using 3D printing to create personalized brain models for neurosurgical training and preoperative planning. World Neurosurg. 90:668–674. 2016.


81. Pucci JU, Christophe BR, Sisti JA, Connolly ES Jr. Three-dimensional printing: technologies, applications, and limitations in neurosurgery. Biotechnol Adv. 35:521–529. 2017.


82. Ritacco LE, Di Lella F, Mancino A, Gonzalez Bernaldo de Quiros F, Boccio C, Milano FE. 3D printed models and navigation for skull base surgery: case report and virtual validation. Stud Health Technol Inform. 216:1025. 2015.
83. Romero-Garcia R, Erez Y, Oliver G, Owen M, Merali S, Poologaindran A, et al. Practical application of networks in neurosurgery: combined 3-dimensional printing, neuronavigation, and preoperative surgical planning. World Neurosurg. 137:e126–e137. 2020.


84. Ryan JR, Almefty KK, Nakaji P, Frakes DH. Cerebral aneurysm clipping surgery simulation using patient-specific 3D printing and silicone casting. World Neurosurg. 88:175–181. 2016.


85. Ryan JR, Chen T, Nakaji P, Frakes DH, Gonzalez LF. Ventriculostomy simulation using patient-specific ventricular anatomy, 3D printing, and hydrogel casting. World Neurosur. 84:1333–1339. 2015.


86. Shah A, Jankharia B, Goel A. Three-dimensional model printing for surgery on arteriovenous malformations. Neurol India. 65:1350–1354. 2017.


87. Shah KJ, Peterson JC, Beahm DD, Camarata PJ, Chamoun RB. Threedimensional printed model used to teach skull base anatomy through a transsphenoidal approach for neurosurgery residents. Oper Neurosurg (Hagerstown). 12:326–329. 2016.


88. Shen Z, Xie Y, Shang X, Xiong G, Chen S, Yao Y, et al. The manufacturing procedure of 3D printed models for endoscopic endonasal transsphenoidal pituitary surgery. Technol Health Care. 28:131–150. 2020.


89. Stefan P, Pfandler M, Lazarovici M, Weigl M, Navab N, Euler E, et al. Three-dimensional-printed computed tomography-based bone models for spine surgery simulation. Simul Healthc. 15:61–66. 2020.


90. Sullivan S, Aguilar-Salinas P, Santos R, Beier AD, Hanel RA. Three-dimensional printing and neuroendovascular simulation for the treatment of a pediatric intracranial aneurysm: case report. J Neurosurg Pediatr. 22:672–677. 2018.


91. Tai BL, Rooney D, Stephenson F, Liao PS, Sagher O, Shih AJ, et al. Development of a 3D-printed external ventricular drain placement simulator: technical note. J Neurosurg. 123:1070–1076. 2015.


92. Tai BL, Wang AC, Joseph JR, Wang PI, Sullivan SE, McKean EL, et al. A physical simulator for endoscopic endonasal drilling techniques: technical note. J Neurosurg. 124:811–816. 2016.


93. Thawani JP, Pisapia JM, Singh N, Petrov D, Schuster JM, Hurst RW, et al. Three-dimensional printed modeling of an arteriovenous malformation including blood flow. World Neurosurg. 90:675–683. 2016.


94. Thawani JP, Singh N, Pisapia JM, Abdullah KG, Parker D, Pukenas BA, et al. Three-dimensional printed modeling of diffuse low-grade gliomas and associated white matter tract anatomy. Neurosurgery. 80:635–645. 2017.


95. Umemura T, Nishizawa S, Miyachi H, Yamamoto J. Removal of double cavernous angioma of the frontal lobe using a three-dimensional printed model: a case report. J UOEH. 42:217–222. 2020.


96. Van Dijk M, Smit TH, Jiya TU, Wuisman PI. Polyurethane real-size models used in planning complex spinal surgery. Spine (Phila Pa 1976). 26:1920–1926. 2001.


97. Wang JL, Yuan ZG, Qian GL, Bao WQ, Jin GL. 3D printing of intracranial aneurysm based on intracranial digital subtraction angiography and its clinical application. Medicine (Baltimore). 97:e11103. 2018.


98. Wang L, Ye X, Hao Q, Chen Y, Chen X, Wang H, et al. Comparison of two three-dimensional printed models of complex intracranial aneurysms for surgical simulation. World Neurosurg. 103:671–679. 2017.


99. Wang L, Ye X, Hao Q, Ma L, Chen X, Wang H, et al. Three-dimensional intracranial middle cerebral artery aneurysm models for aneurysm surgery and training. J Clin Neurosci. 50:77–82. 2018.


100. Waran V, Devaraj P, Hari Chandran T, Muthusamy KA, Rathinam AK, Balakrishnan YK, et al. Three-dimensional anatomical accuracy of cranial models created by rapid prototyping techniques validated using a neuronavigation station. J Clin Neurosci. 19:574–577. 2012.


101. Waran V, Menon R, Pancharatnam D, Rathinam AK, Balakrishnan YK, Tung TS, et al. The creation and verification of cranial models using three-dimensional rapid prototyping technology in field of transnasal sphenoid endoscopy. Am J Rhinol Allergy. 26:e132–e136. 2012.


102. Waran V, Narayanan V, Karuppiah R, Owen SL, Aziz T. Utility of multimaterial 3D printers in creating models with pathological entities to enhance the training experience of neurosurgeons. J Neurosurg. 120:489–492. 2014.


103. Waran V, Narayanan V, Karuppiah R, Pancharatnam D, Chandran H, Raman R, et al. Injecting realism in surgical training-initial simulation experience with custom 3D models. J Surg Educ. 71:193–197. 2014.


104. Waran V, Narayanan V, Karuppiah R, Thambynayagam HC, Muthusamy KA, Rahman ZA, et al. Neurosurgical endoscopic training via a realistic 3-dimensional model with pathology. Simul Healthc. 10:43–48. 2015.


105. Waran V, Pancharatnam D, Thambinayagam HC, Raman R, Rathinam AK, Balakrishnan YK, et al. The utilization of cranial models created using rapid prototyping techniques in the development of models for navigation training. J Neurol Surg A Cent Eur Neurosurg. 75:12–15. 2014.


106. Weinstock P, Prabhu SP, Flynn K, Orbach DB, Smith E. Optimizing cerebrovascular surgical and endovascular procedures in children via personalized 3D printing. J Neurosurg Pediatr. 16:584–589. 2015.


107. Weinstock P, Rehder R, Prabhu SP, Forbes PW, Roussin CJ, Cohen AR. Creation of a novel simulator for minimally invasive neurosurgery: fusion of 3D printing and special effects. J Neurosurg Pediatr. 20:1–9. 2017.


108. Weiss MY, Melnyk R, Mix D, Ghazi A, Vates GE, Stone JJ. Design and validation of a cervical laminectomy simulator using 3D printing and hydrogel phantoms. Oper Neurosurg (Hagerstown). 18:202–208. 2020.


109. Wen G, Cong Z, Liu K, Tang C, Zhong C, Li L, et al. A practical 3D printed simulator for endoscopic endonasal transsphenoidal surgery to improve basic operational skills. Childs Nerv Syst. 32:1109–1116. 2016.


110. Wu AM, Wang K, Wang JS, Chen CH, Yang XD, Ni WF, et al. The addition of 3D printed models to enhance the teaching and learning of bone spatial anatomy and fractures for undergraduate students: a randomized controlled study. Ann Transl Med. 6:403. 2018.


111. Wu ZX, Huang LY, Sang HX, Ma ZS, Wan SY, Cui G, et al. Accuracy and safety assessment of pedicle screw placement using the rapid prototyping technique in severe congenital scoliosis. J Spinal Disord Tech. 24:444–450. 2011.


112. Wurm G, Tomancok B, Pogady P, Holl K, Trenkler J. Cerebrovascular stereolithographic biomodeling for aneurysm surgery. Technical note. J Neurosurg. 100:139–145. 2004.
113. Xu WH, Liu J, Li ML, Sun ZY, Chen J, Wu JH. 3D printing of intracranial artery stenosis based on the source images of magnetic resonance angiograph. Ann Transl Med. 2:74. 2014.
114. Yang M, Li C, Li Y, Zhao Y, Wei X, Zhang G, et al. Application of 3D rapid prototyping technology in posterior corrective surgery for Lenke 1 adolescent idiopathic scoliosis patients. Medicine (Baltimore). 94:e582. 2015.


115. Ye X, Wang L, Li K, Hao Q, Lu J, Chen X, et al. A three-dimensional color-printed system allowing complete modeling of arteriovenous malformations for surgical simulations. J Clin Neurosci. 77:134–141. 2020.


116. Yi Z, He B, Liu Y, Huang S, Hong W. Development and evaluation of a craniocerebral model with tactile-realistic feature and intracranial pressure for neurosurgical training. J Neurointerv Surg. 12:94–97. 2020.


117. Zheng JP, Li CZ, Chen GQ. Multimaterial and multicolor 3D-printed model in training of transnasal endoscopic surgery for pituitary adenoma. Neurosurg Focus. 47:E21. 2019.


118. Zheng JP, Li CZ, Chen GQ, Song GD, Zhang YZ. Three-dimensional printed skull base simulation for transnasal endoscopic surgical training. World Neurosurg. 111:e773–e782. 2018.


Fig. 1.
Annual distribution of the number of papers on three-dimensional (3D)-printed disease models for surgical planning, simulation, and training in the neurosurgical field published in English literature. *From January to August.
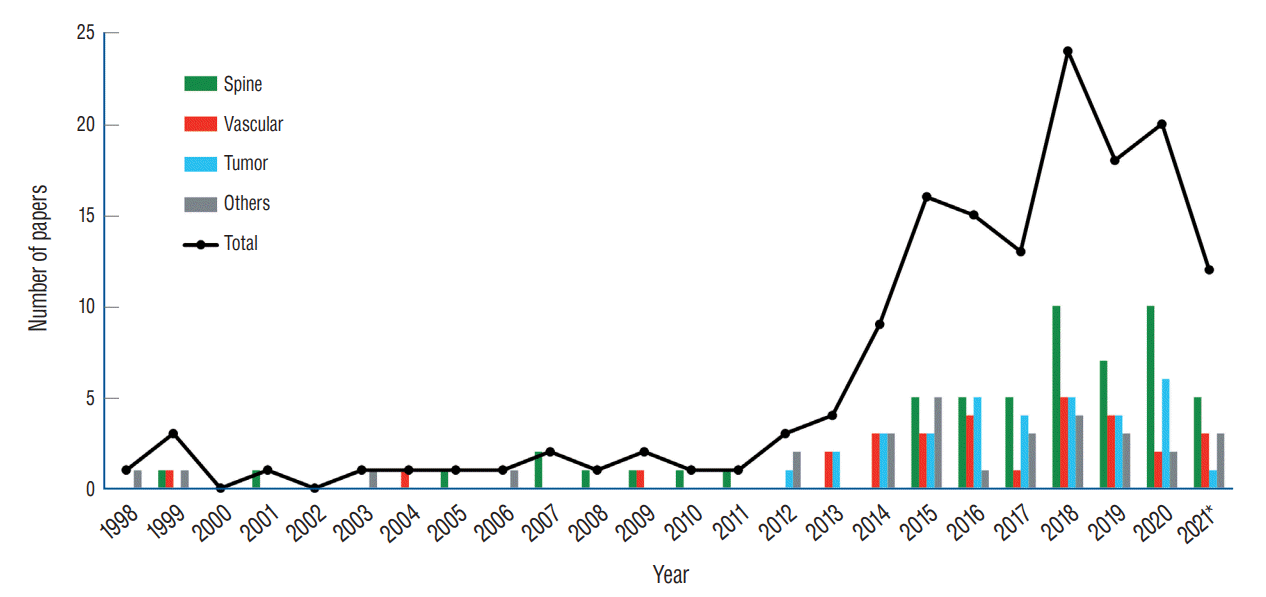
Fig. 2.
The representative patient-specific three-dimensional (3D)-printed disease models. A : Spinal disease model of lumbar stenosis. B : Cerebrovascular disease model of arteriovenous malformation. C : Brain tumor model of insular glioma.
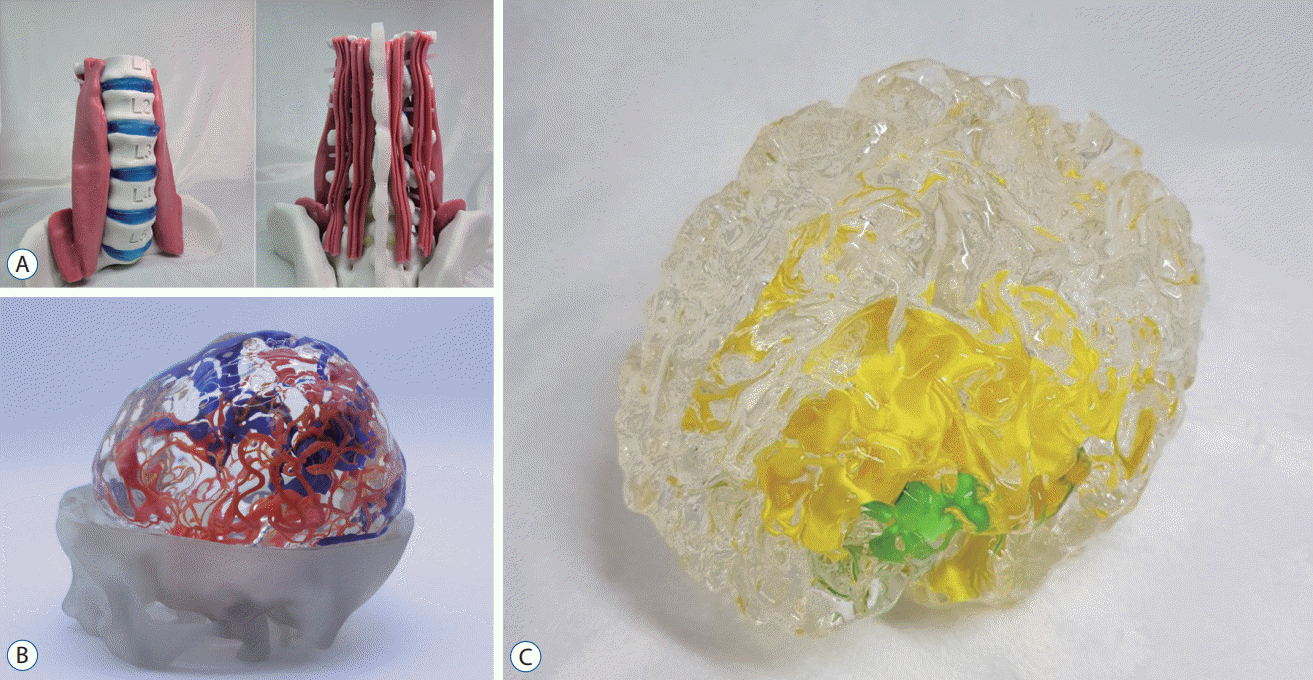
Table 1.
Classification and characteristics according to the purpose of use of the 3D-printed neurosurgical disease models