Abstract
Objective
Hydrocephalus after intracerebral hemorrhage (ICH) is known to be related to poor prognosis and mortality. We analyzed predictors of permanent hydrocephalus in the patients with surgically treated supratentorial ICH.
Methods
From 2004 to 2019, a total of 414 patients with surgically treated primary supratentorial ICH were included. We retrospectively analyzed age, sex, preexisting hypertension and diabetes, location and volume of ICH, presence and severity of intraventricular hemorrhage (IVH), and type of surgery.
Results
Forty patients (9.7%) required shunt surgery. Concomitant IVH was higher in the ‘shunt required’ group (92.5%) than in the ‘shunt not required’ group (67.9%) (p=0.001). IVH severity was worse in the ‘shunt required’ group (13.5 vs. 7.5, p=0.008). Craniectomy (47.5%) was significantly high in the ‘shunt required’ group. According to multivariable analysis, the presence of an IVH was 8.1 times more frequent and craniectomy was 8.6 times more frequent in the ‘shunt required’ group. In the comparison between craniotomy and craniectomy group, the presence of an IVH was related with a 3.9 times higher (p=0.033) possibility and craniectomies rather than craniotomies with a 7-times higher possibility of shunt surgery (p<0.001). Within the craniectomy group, an increase in the craniectomy area by 1 cm2 was correlated with a 3.2% increase in the possibility of shunt surgery (odds ratio, 1.032; 95% confidence interval, 1.005-1.061; p=0.022).
Intracerebral hemorrhages (ICHs) are a common debilitating type of stroke and accounts for approximately 7% to 20% of all stroke cases in western countries [5,12,14] and 23% to 30% of all stroke cases in Asia [12,31]. Hydrocephalus occurs in 13–46% of primary ICH patients [23,29]. ICH-related hydrocephalus correlates with a poor prognosis [4,9,22] and is an independent predictor of mortality [9]. Hydrocephalus after an ICH can be developed acutely due to disturbance of normal cerebral spinal fluid (CSF) flow. Excess accumulation of parenchymal or ventricular blood accounts for 45% of all hydrocephalus cases [37]. Permanent hydrocephalus that requires CSF diversion accounts for approximately 20% to 30% of all cases after acute ventricular drainage [36,38]. Permanent hydrocephalus is related to additional morbidities; external ventricular drainage (EVD) is repeated, risks of ventriculitis are increased and ultimately shunt surgery is required. Previous studies have reported multiple predictors of shunt-dependent hydrocephalus, such as thalamic location, posterior fossa location, presence of intraventricular hemorrhage (IVH), IVH volume, elevated intracranial pressure (ICP), or old age [23,30,36]. Surgical factors on development of hydrocephalus have been studied mostly in head trauma but few in primary ICH [11,32]. Craniectomy is known to be related to hydrocephalus in head traum [11]. In this report we want to focus on the influence of surgical type on development of shunt dependent hydrocephalus in the ICH patients.
This study was approved by the Institutional Review Board (IRB) of Konkuk University Medical Center as a retrospective observational study (IRB No. KUMC 2020-02-007). The requirement for written informed consent was waived by the IRB owing to the retrospective nature of this study. In this study, we included patients who suffered from a first primary supratentorial ICH between the years of 2004 and 2019. Specifically, we confined the analysis to the subjects who were surgically treated and were followed up more than at least 4 weeks after the initial hematoma surgery. Exclusion criteria included patients less than 20 years of age, as well as patients with infratentorial lesions, multiple lesions, secondary ICHs that developed as a result of vascular malformation, cerebral aneurysm, Moyamoya disease, tumors, trauma, thrombolysisrelated hemorrhage, or drug-related hemorrhage.
The probable factors affecting the development of shunt-dependent hydrocephalus were estimated. Patient factors were included, including age, sex, preexisting hypertension, and preexisting diabetes. For hemorrhagic factors, we estimated the location and volume of ICH and the presence and severity of IVH. Surgical factors were recorded according to the type of surgical intervention, i.e., craniectomy, craniotomy, EVD, ICH catheterization, and shunt only; in whom initial hematoma was conservatively treated but shunt surgery was required after resolution of ICH.
The ICH location was divided into two categories. ICHs located in the basal ganglia or the thalamus were considered “deep”; when they were located in subcortical or cortical areas they were considered “lobar.” Volume was calculated using the ABC/2 formula on brain computed tomography (CT) scans. The severity of IVH was scored according to the modified Graeb score [24]. The score is decided based on how much blood is filled within each ventricle; fourth ventricle (maximum score 4), third (maximum score 4), right and left lateral ventricles (maximum score 4 for each), right and left occipital horns (maximum score 2 for each), and the right and left temporal horns (maximum score 2 for each). An additional score of +1 is given to each compartment if it is expanded beyond normal anatomic limits attributable to clot. The maximum possible score is 32, in which every compartment is filled with blood and expanded. A score of 0 denotes no intraventricular blood [24]. About a surgical type conservative treatment was favored for ICHs with a volume of less than 20 mL. Catheterization and/or EVD was favored when the ICH volume was between 20 and 40 mL. Open craniotomy or craniectomy was performed for cases with larger ICH volumes, i.e., over 40 mL. The choice between a craniotomy or craniectomy was typically decided according to the surgeon’s predilection. In cases of repeated surgery, the one with the larger surgical extent was assigned as the surgical method. Cases with simultaneous parenchymal catheterization and EVD were categorized as EVD. Multiple catheters within a hematoma were counted as one catheterization surgery. Shunt surgeries were performed in the patients; who showed progressively enlarged ventricles with deteriorating or failures in improvement of neurologic state; who were not tolerable to gradual reduction of amount of CSF drainage. For patients who were treated with craniectomies, the size of the craniectomy was approximated using the formula for an ellipse : AB/4, where A is the longest anterior-posterior length as determined on the axial CT cuts, and B is calculated using CT slice thickness and the number of axial cuts containing the craniotomy [20].
Shunt related factors were compared in the ‘shunt required’ group and the ‘shunt not required’ group. To identify the influence of surgical type on the shut dependency, subgroup analysis was done in the patients who had been treated with craniotomy or craniectomy. Additional subgroup analysis was done in the craniectomy patients.
R version 3.3.3 (R Foundation for Statistical Computing, Vienna, Austria) and the T&F program version 3.0 (YooJin BioSoft, Seoul, Korea) were used for all statistical analyses. Continuous variables were expressed as mean±standard deviation or median (interquartile ranges) according to the normality of the variable. Sample numbers and percentages were computed for categorical variables. The mean difference between the shunt and no shunt subgroups, or between the craniotomy and craniectomy patients, were analyzed using Student’s t-tests or Mann-Whitney U tests according to the normality of the variable. The normality of the variable was estimated using Lilliefors’ (Kolmogorov-Smirnov) normality test and the Shapiro-Wilk normality test. Chi-squared tests were performed to analyze proportions of sample numbers in the subgroups of categorical variables. Logistic regression analysis was performed to analyze the effects of patients’ baseline factors, hemorrhage, and surgical factors on the occurrence of shunts. To analyze the independent effects of more than two risk factors on shunt occurrence, multivariable regression analysis was performed using a backward stepwise variable elimination procedure as a best-fit model selection method to minimize the Akaike information criterion. Statistical significance was defined with a p-value of <0.05.
From the year of 2004 to 2019, 2692 patients were admitted to our hospital with a supratentorial ICH. Six hundred ninety-nine patients out of the 2692 were surgically treated. According to the exclusion criteria, we excluded patients with a secondary ICH (n=101), early death or short-term follow-up (n=184). A total of 414 patients were selected as final candidates for this study. Forty patients (9.7%) out of them required shunt surgery.
The mean age of the whole patients was 59.4±13.7 years. Demographic data in each group were described in Table 1. Statistical differences in age and sex were not observed between the ‘shunt required’ and ‘shunt not required’ groups. Shunt surgery was more frequently associated with patients with preexisting hypertension (67.5% vs. 52.7%, p=0.074) and diabetes (27.5% vs. 15.5%, p=0.053); however, these differences did not reach statistical significance. The location of ICH (deep 62.5% vs. lobar 70.9%, p=0.273) and the volume of hematoma (48.2 [21.4–75.6] mL vs. 39.5 [21.7–63.1] mL, p=0.419) did not show significant difference between the ‘shunt required’ group and ‘shunt not required’ group. Concomitant IVH was more frequently observed in the ‘shunt required’ group (92.5%) than in the ‘shunt not required’ group (67.9%) (p=0.001). IVH severity was significantly worse in the ‘shunt required’ group (13.5 [5.3–19] vs. 7.5 [0–16], p=0.008). Relative to surgical method used, ‘shunt required’ group was most frequently associated with craniectomy (47.5%) followed by EVD (25.0%), craniotomy (20.0%), and catheterization (0%), while ‘shunt not required’ group was most frequently associated with craniotomy (41.7%) followed by catheterization (30.5%), EVD (14.7%), and craniectomy (13.1%) (p<0.001) (Table 1). According to multivariable analysis, the presence of an IVH and the variation of the surgical method used were meaningful factors. The presence of an IVH was 8.1 times more frequent and craniectomy was 8.6 times more frequent than craniotomy in the ‘shunt required’ group (Table 2).
Among the 232 patients treated with craniectomies or craniotomies, 68 had undergone craniectomies, and 164 had undergone craniotomies. No statistical difference was observed between the two groups according to age, sex, presence of hypertension, location of ICH, volume of ICH, and presence of an IVH. Diabetes was more frequently observed in the craniectomy group (25% vs. 13.4%, p=0.032). IVH severity scores were also worse in the craniectomy group (10 [0.7–17.7] vs. 5 [0–13], p=0.005). As shown in Table 1, significantly more shunt surgeries were performed in the craniectomy group than in the craniotomy group (27.9% vs. 4.9%, p<0.001) (Table 3). According to a multivariable logistic regression analysis in the craniectomy or craniotomy operated patients, the presence of an IVH was correlated with a 3.9 times higher possibility of shunt surgery relative to patients without an IVH (p=0.033). Craniectomies were shown to be associated with a 7-times higher possibility of shunt surgery than craniotomies (p<0.001) (Table 3). Within the craniectomy group, craniectomies size, which had a mean of 68.5 cm2, ranging from 17.1 to 120.1 cm2, was found to be the only factor affecting shunt surgery frequency. Specifically, an increase in the craniectomy area by 1 cm2 was correlated with a 3.2% increase in the possibility of shunt surgery (odds ratio, 1.032; 95% confidence interval, 1.005–1.061; p=0.022) (Table 4). Cases of shunt-dependent hydrocephalus after craniotomies or craniectomies were illustrated differentially in Fig. 1.
Understanding the complexities involved in the management of parenchymal cerebral hemorrhage remains challenging, thereby offering many areas for further investigation. Several predictors of ICH-related hydrocephalus have been reported, including low-presenting GCS [21], the thalamic location of ICH [36], elevated ICP [4,9,23,36], old age, and hypertension and slow CSF circulation [19]. In our study, the presence of IVH, the severity of IVH, decompressive craniectomy, and larger craniectomy showed association with the development of shunt dependent hydrocephalus.
IVHs are a well-known risk factor for the development of hydrocephalus and are associated with poor prognosis after ICH [4,8]. Intraventricular blood and its breakdown products cause inflammation of the ependymal layer and subependymal brain tissue. Blood clots cause inflammation and fibrosis of the arachnoid granulations, leading to delayed communicating hydrocephalus. The presence of an IVH occurs in about 45% of ICH patients at presentation [33]. In our study, an IVH was noted in 70.3% of patients. This high rate of association is likely due to the inclusion of only surgically managed patients.
The effective or early removal of an IVH and the restoration of CSF circulation has been attempted due to the unfavorable influence of an IVH on the development of hydrocephalus and neurologic prognosis. Some attempts have been reported to reduce shunt dependency. Endoscopic surgical evacuation of an IVH and ventriculostomy was reported to reduce permanent CSF diversion compared to EVD [3,7,34,39]. Also, early lumbar drainage following the removal of third and fourth ventricular obstructions after an ICH has shown a reduction in the rate of ICH-mediated shunt dependency [17,18,28]. At our institution, endoscopic removal was not available and lumbar drainage was not attempted during the acute period of ICH development. However, when large ventricular blood clots were noted, simultaneous bilateral catheters were usually inserted into both frontal horns. Previous studies suggest that IVH volume is an important determinant in supratentorial ICH outcome [30]. Endoscopic removal of ventricular blood has been shown to reduced mortality [39]. Additionally, the intraventricular administration of fibrinolytic agents, including urokinase, streptokinase, and recombinant tissue-type plasminogen activator (rtPA) in IVHs may reduce morbidity and mortality by accelerating blood clearance and clot lysis [6,13,25,26]. Removal of blood clots, as well as intracranial pressure relief, should be considered as treatments for the management of IVHs.
A decompressive craniectomy is usually performed to treat uncontrollable intracranial hypertension that may be due to a variety of causes. The benefits of this procedure are related to reductions in ICP, which improve brain oxygenation, cerebral perfusion, and compliance. However, decompressive craniectomy can be associated with multiple risks, such as subdural effusion with an incidence from 11.2–62% [19], syndromes of the trephined (incidence of 7–11%) [27], herniation of the cortex through the bone defect (14.6–51%), seizure (3.4–22%), hydrocephalus (7.9–14%), and infection (4.5–15.7%) [2,10,15,16,35]. Many of these complications are thought to be related to a disruption of normal pathophysiological changes among ICP, CSF circulation and CBF following the removal of a large area of the skull.
Hypotheses have been presented regarding the development of hydrocephalus or intracranial fluid collection after craniectomy. Assuming that arachnoidal granulations function as pressure-dependent one-way valves from the subarachnoid space to drain the venous sinuses, Waziri et al. [32] hypothesized that a disruption of the pulsatile ICP dynamics secondary to the cranial defect may result in decreased CSF outflow. A “flattening” of the normally dicrotic ICP waveform after hemicraniectomy was observed while monitoring the ICP. This flattening was considered a result of the transmission of the pressure pulse out of the open cranium [32]. Akins and Guppy [1] summarized that the loss of brain pulsatility after decompressive craniectomy causes impaired cerebral blood flow, CSF hydrodynamics, loss of pulsatile CSF circulation, and reduced glymphatic circulation. These effects lead to an accumulation of cranial fluid within the ventricles, the subarachnoid or subdural space, and the subgaleal compartments [1]. Our result also demonstrated that choice of craniectomy and larger craniectomy size were related to the development of shunt dependent hydrocephalus. ICH develops in focal brain areas, which means if the ICH were well removed and the increased brain pressure were relieved, additional decompression via the craniectomy may not be necessary. Overcorrection of IICP in ICH patients seems to lead an impairments of CSF circulation and subsequent shunt dependency which could have been avoidable. The increasing size of craniectomy was related with increasing rate of shunt requirement in this study. This result is supportive that the craniectomy is contributing the development of shunt dependent hydrocephalus.
Limitations of this study include the exclusion of patients who died within 4 weeks of surgery. Many of these patients were believed to have large volume hematomas that were treated by craniectomy. Thus, the results of our study can only be applied to survivors of ICHs.
Notes
References
1. Akins PT, Guppy KH. Are hygromas and hydrocephalus after decompressive craniectomy caused by impaired brain pulsatility, cerebrospinal fluid hydrodynamics, and glymphatic drainage? Literature overview and illustrative cases. World Neurosurg. 130:e941–e952. 2019.


2. Ban SP, Son YJ, Yang HJ, Chung YS, Lee SH, Han DH. Analysis of complications following decompressive craniectomy for traumatic brain injury. J Korean Neurosurg Soc. 48:244–250. 2010.


3. Basaldella L, Marton E, Fiorindi A, Scarpa B, Badreddine H, Longatti P. External ventricular drainage alone versus endoscopic surgery for severe intraventricular hemorrhage: a comparative retrospective analysis on outcome and shunt dependency. Neurosurg Focus. 32:E4. 2012.


4. Bhattathiri PS, Gregson B, Prasad KS, Mendelow AD, STICH Investigators. Intraventricular hemorrhage and hydrocephalus after spontaneous intracerebral hemorrhage: results from the STICH trial. Acta Neurochir Suppl. 96:65–68. 2006.


5. Broderick JP, Adams HP Jr, Barsan W, Feinberg W, Feldmann E, Grotta J, et al. Guidelines for the management of spontaneous intracerebral hemorrhage: a statement for healthcare professionals from a special writing group of the Stroke Council, American Heart Association. Stroke. 30:905–915. 1999.


6. Castaño Ávila S, Corral Lozano E, Vallejo De La Cueva A, Maynar Moliner J, Martín López A, Fonseca San Miguel F, et al. Intraventricular hemorrhage treated with intraventricular fibrinolysis. A 10-year experience. Med Intensiva. 37:61–66. 2013.


7. Chen CC, Liu CL, Tung YN, Lee HC, Chuang HC, Lin SZ, et al. Endoscopic surgery for intraventricular hemorrhage (IVH) caused by thalamic hemorrhage: comparisons of endoscopic surgery and external ventricular drainage (EVD) surgery. World Neurosurg. 75:264–268. 2011.


8. Dastur CK, Yu W. Current management of spontaneous intracerebral haemorrhage. Stroke Vasc Neurol. 24:21–29. 2017.


9. Diringer MN, Edwards DF, Zazulia AR. Hydrocephalus: a previously unrecognized predictor of poor outcome from supratentorial intracerebral hemorrhage. Stroke. 29:1352–1387. 1998.


10. Faleiro RM, Faleiro LC, Caetano E, Gomide I, Pita C, Coelho G, et al. Decompressive craniotomy: prognostic factors and complications in 89 patients. Arq Neuropsiquiatr. 66:369–373. 2008.


11. Fattahian R, Bagheri SR, Sadeghi M. Development of posttraumatic hydrocephalus requiring ventriculoperitoneal shunt after decompressive craniectomy for traumatic brain injury: a systematic review and meta-analysis of retrospective studies. Med Arch. 72:214–219. 2018.


12. Feigin VL, Lawes CM, Bennett DA, Anderson CS. Stroke epidemiology: a review of population-based studies of incidence, prevalence, and case-fatality in the late 20th century. Lancet Neurol. 2:43–53. 2003.


13. Gaberel T, Magheru C, Parienti JJ, Huttner HB, Vivien D, Emery E. Intraventricular fibrinolysis versus external ventricular drainage alone in intraventricular hemorrhage: a meta-analysis. Stroke. 42:2776–2781. 2011.


14. Hänggi D, Steiger HJ. Spontaneous intracerebral haemorrhage in adults: a literature overview. Acta Neurochir (Wien). 150:371–379. 2008.


15. Honeybul S. Complications of decompressive craniectomy for head injury. J Clin Neurosci. 17:430–435. 2010.


16. Honeybul S, Ho KM. Long-term complications of decompressive craniectomy for head injury. J Neurotrauma. 28:929–935. 2011.


17. Huttner HB, Schwab S, Bardutzky J. Lumbar drainage for communicating hydrocephalus after ICH with ventricular hemorrhage. Neurocrit Care. 5:193–196. 2006.


18. Huttner HB, Nagel S, Tognoni E, Köhrmann M, Jüttler E, Orakcioglu B, et al. Intracerebral hemorrhage with severe ventricular involvement: lumbar drainage for communicating hydrocephalus. Stroke. 38:183–187. 2007.


19. Kaen A, Jimenez-Roldan L, Alday R, Gomez PA, Lagares A, Alén JF, et al. Interhemispheric hygroma after decompressive craniectomy: does it predict posttraumatic hydrocephalus? J Neurosurg. 113:1287–1293. 2010.


20. Kim BO, Kim JY, Whang K, Cho SM, Oh JW, Koo YM, et al. The risk factors of subdural hygroma after decompressive craniectomy. Korean J Neurotrauma. 14:93–98. 2018.


21. Mahta A, Katz PM, Kamel H, Azizi SA. Intracerebral hemorrhage with intraventricular extension and no hydrocephalus may not increase mortality or severe disability. J Clin Neurosci. 30:56–59. 2016.


22. Mendelow AD, Gregson BA, Mitchell PM, Murray GD, Rowan EN, Gholkar AR, et al. Surgical trial in lobar intracerebral haemorrhage (STICH II) protocol. Trials. 12:124. 2011.


23. Miller C, Tsivgoulis G, Nakaji P. Predictors of ventriculoperitoneal shunting after spontaneous intraparenchymal hemorrhage. Neurocrit Care. 8:235–240. 2008.


24. Morgan TC, Dawson J, Spengler D, Lees KR, Aldrich C, Mishra NK, et al. The modified graeb score: an enhanced tool for intraventricular hemorrhage measurement and prediction of functional outcome. Stroke. 44:635–641. 2013.
25. Naff NJ, Hanley DF, Keyl PM, Tuhrim S, Kraut M, Bederson J, et al. Intraventricular thrombolysis speeds blood clot resolution: results of a pilot, prospective, randomized, double-blind, controlled trial. Neurosurgery. 54:577–583. 2004.


26. Pang D, Sclabassi RJ, Horton JA. Lysis of intraventricular blood clot with urokinase in a canine model: part 3. Effects of intraventricular urokinase on clot lysis and posthemorrhagic hydrocephalus. Neurosurgery. 19:553–572. 1986.


27. Sarov M, Guichard JP, Chibarro S, Guettard E, Godin O, Yelnik A, et al. Sinking skin flap syndrome and paradoxical herniation after hemicraniectomy for malignant hemispheric infarction. Stroke. 41:560–562. 2010.


28. Staykov D, Kuramatsu JB, Bardutzky J, Volbers B, Gerner ST, Kloska SP, et al. Efficacy and safety of combined intraventricular fibrinolysis with lumbar drainage for prevention of permanent shunt dependency after intracerebral hemorrhage with severe ventricular involvement: a randomized trial and individual patient data meta-analysis. Ann Neurol. 81:93–103. 2017.


29. Sumer MM, Açikgöz B, Akpinar G. External ventricular drainage for acute obstructive hydrocephalus developing following spontaneous intracerebral haemorrhages. Neurol Sci. 23:29–33. 2002.


30. Tuhrim S, Horowitz DR, Sacher M, Godbold JH. Volume of ventricular blood is an important determinant of outcome in supratentorial intracerebral hemorrhage. Crit Care Med. 27:617–621. 1999.


31. Wang W, Jiang B, Sun H, Ru X, Sun D, Wang L, et al. Prevalence, incidence, and mortality of stroke in China: results from a nationwide population-based survey of 480 687 adults. Circulation. 135:759–771. 2017.


32. Waziri A, Fusco D, Mayer SA, McKhann GM 2nd, Connolly ES Jr. Postoperative hydrocephalus in patients undergoing decompressive hemicraniectomy for ischemic or hemorrhagic stroke. Neurosurgery. 61:489–493. 2007.


33. Xi G, Strahle J, Hua Y, Keep RF. Progress in translational research on intracerebral hemorrhage: is there an end in sight? Prog Neurobiol. 115:45–63. 2014.


34. Yadav YR, Mukerji G, Shenoy R, Basoor A, Jain G, Nelson A. Endoscopic management of hypertensive intraventricular haemorrhage with obstructive hydrocephalus. BMC Neurol. 7:1. 2007.


35. Yang XF, Wen L, Shen F, Li G, Lou R, Liu WG, et al. Surgical complications secondary to decompressive craniectomy in patients with a head injury: a series of 108 consecutive cases. Acta Neurochir (Wien). 150:1241–1247. 2008.


36. Zacharia BE, Vaughan KA, Hickman ZL, Bruce SS, Carpenter AM, Petersen NH, et al. Predictors of long-term shunt-dependent hydrocephalus in patients with intracerebral hemorrhage requiring emergency cerebrospinal fluid diversion. Neurosurg Focus. 32:E5. 2012.


Fig. 1.
Illustrative cases of shunt-dependent hydrocephalus in patients with intracerebral hemorrhage (ICH). A : A 50-year-old male presented with right putaminal hemorrhage (left column). After a craniectomy, the ICH was removed completely (middle column), and hydrocephalus developed at 2 months after ICH (right column). B : A 54-year-old male presented with right posterior putaminal hemorrhage (left column). After a craniotomy, the ICH was removed completely (middle column), and he was without signs of hydrocephalus at 2 months after ICH (right column). C : A 70-year-old female presented with a right frontal cortical hematoma (left column). After a craniectomy, the ICH was removed completely (middle column), and hydrocephalus developed at 2 weeks after surgery (right column). D : A 74-year-old female presented with right occipital cortical hemorrhage with ventricular extension (left column). With a craniotomy, the ICH and IVH were removed (middle column), and she was without any sign of hydrocephalus at 2 months after ICH (right column).
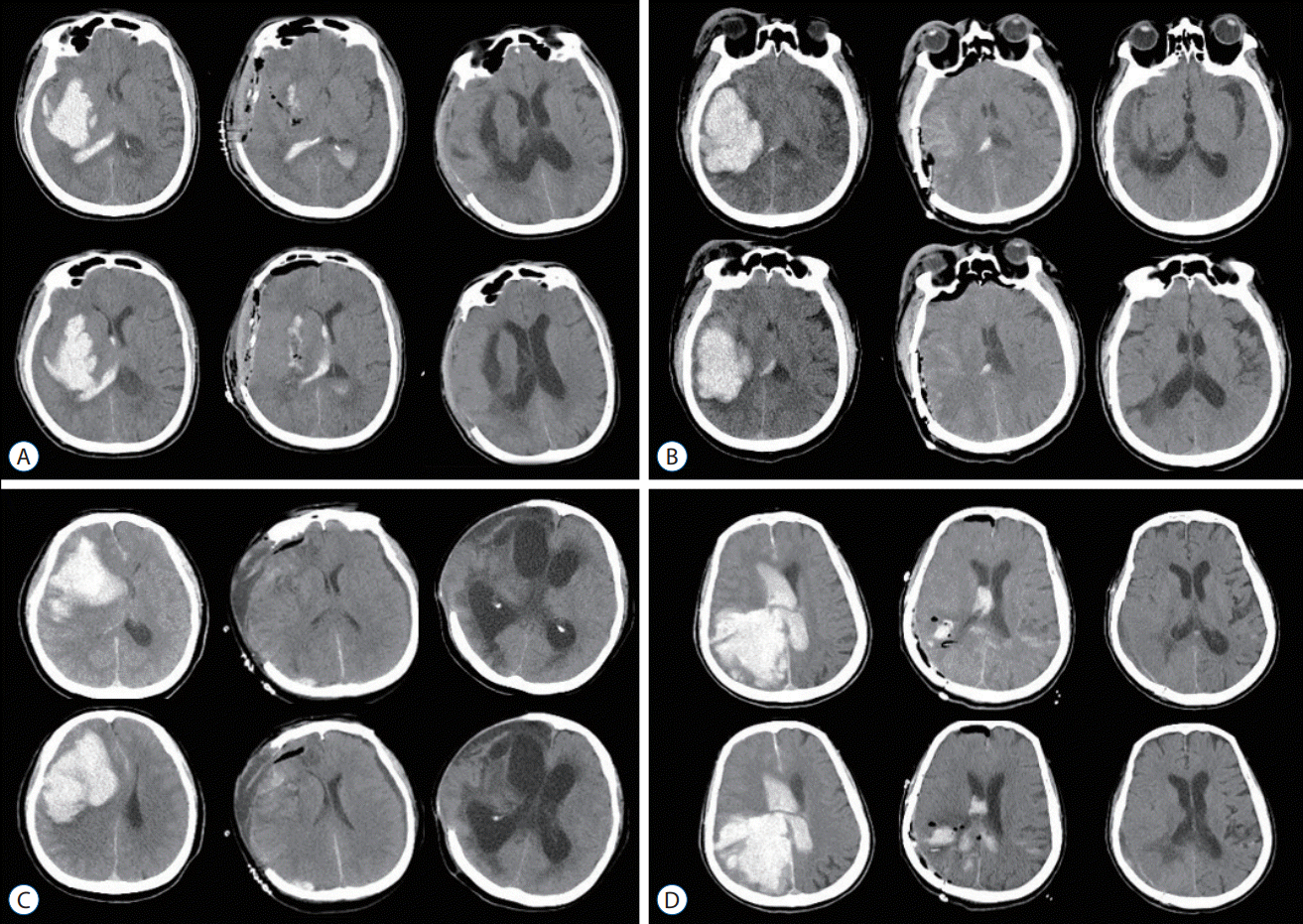
Table 1.
Baseline characteristics of the variables
Table 2.
Result of multivariable binary logistic regression analysis on shunt surgery
Predictor (response=shunt) |
Multivariable |
p-value |
---|---|---|
OR (95% CI) | ||
Age | 1.007 (0.977–1.038) | 0.636 |
Sex, male vs. female | 1.054 (0.487–2.281) | 0.893 |
Hypertension, yes vs. no | 1.857 (0.854–4.038) | 0.118 |
Diabetes, yes vs. no | 1.263 (0.537–2.972) | 0.593 |
ICH location | ||
Deep vs. lobar | 2.234 (0.89–5.605) | 0.087 |
ICH volume | 0.988 (0.975–1.002) | 0.082 |
IVH | ||
Yes vs. no | 8.149 (1.798–36.921) | 0.007 |
IVH severity | 0.994 (0.934–1.058) | 0.845 |
Operation | ||
Craniectomy vs. craniotomy | 8.636 (3.343–22.31) | <0.001 |
Others* vs. craniotomy | 1.199 (0.413–3.483) | 0.739 |
Table 3.
Distribution of variables between craniotomy and craniectomy
Variable | Craniectomy (n=68) | Craniotomy (n=164) | p-value | OR (95% CI)* |
---|---|---|---|---|
Age (years) | 58.9±13.7 | 61.5±13.2 | 0.179 | - |
Sex | 0.892 | - | ||
Male | 45 (66.2) | 107 (65.2) | ||
Female | 23 (33.8) | 57 (34.8) | ||
Hypertension | 0.917 | - | ||
Yes | 37 (54.4) | 88 (53.7) | ||
Diabetes | 0.032 | - | ||
Yes | 17 (25) | 22 (13.4) | ||
ICH location | 0.190 | - | ||
Deep | 40 (58.8) | 81 (49.4) | ||
Lobar | 28 (41.2) | 83 (50.6) | ||
ICH volume (mL) | 62.8 (43.7–82.5) | 52.7 (35.9–77.8) | 0.086 | - |
IVH | 0.073 | 3.971 (1.118–14.097) | ||
Yes | 51 (75.0) | 103 (62.8) | ||
IVH severity | 10 (0.7–17.7) | 5 (0–13) | 0.005 | - |
Shunt | <0.001 | 7.001 (2.854–17.177) | ||
Yes | 19 (27.9) | 8 (4.9) |
Table 4.
Result logistic regression analysis on shunt in the craniectomy group