Abstract
The newly identified frontal aslant tract (FAT) that connects the posterior Broca’s area to the supplementary motor area is known to be involved in speech and language functions. We successfully intraoperatively monitored FAT using cortico-cortical evoked potentials generated by single-pulse electrical cortical stimulation in a patient with oligodendroglioma.
We used intraoperative neurophysiological monitoring (IONM) to minimize neurological damage from surgery, which can identify important neural structures in the operative field, enabling early detection and intervention.1 Although motor evoked potentials (MEPs) and somatosensory evoked potentials (SSEPs) are widely adapted to protect sensorimotor function, IONM techniques have not recently been used to monitor language function. Cortico-cortical evoked potentials (CCEPs) generated by single-pulse electrical cortical stimulation are known to be useful in the mapping and real-time monitoring of arcuate fasciculus, which is the preestablished language system.2
The frontal aslant tract (FAT) has recently been recognized as a white-matter tract connecting the supplementary motor area (SMA) to the pars opercularis of the inferior frontal gyrus (IFG). This fiber is assumed to be related to language function, especially speech initiation and spontaneity.3 Ookawa et al.4 demonstrated the feasibility of CCEPs for FAT in eight patients who received surgery for epilepsy or brain tumors. We used CCEPs to monitor FAT in a patent with oligodendroglioma in the left frontal cortex.
A 28-year-old male first presented with seizure. A postictal neurological examination did not reveal any neurological deficits such as in language function. Brain magnetic resonance imaging (MRI) revealed a mass in the left SMA, suggesting a low-grade glioma. Functional MRI showed increased blood-oxygen-level-dependent (BOLD) signals in the left frontal cortex adjacent to the mass during verbal language tasks (Fig. 1). During the brain tumor removal, multiple IONM modalities were performed under total intravenous anesthesia using propofol, remifentanil, rocuronium, and phenylephrine. In addition to transcranial MEPs, we also used cortical SSEP phase reversal to delineate the motor cortex. We also used direct cortical stimulation on MEPs to monitor motor function. Finally, we monitored CCEPs at the FAT to prevent language deterioration.
CCEPs were recorded during tumor resection. Each 4 × 5 subdural grid was placed on either the SMA or IFG. Electrical stimulation (ES) was delivered to the SMA or IFG via two adjacent electrodes in a bipolar manner. In each trial, electrocorticograms were time-locked to stimuli, and the average of 20–30 iterations was used to determine CCEP responses. The ES consisted of a constant current square pulse at 1 Hz and 10–15 mA (single pulse, alternating polarity, and 0.3-ms duration). To confirm the reproducibility of each response, we performed two or three trials, which presented the same latencies and amplitudes. We initially applied ES to SMA using an electrocorticogram on the IFG to obtain CCEPSMA→IFG. Similarly, we also applied ES to IFG to obtaining CCEPIFG→SMA (Fig. 2).
During the tumor resection, we monitored FAT using the most prominent CCEPs. The surgery was completed without any abnormal CCEP N1 response (Fig. 3). After surgery, the patient had no neurological deficits such as in verbal or language function.
To the best of our knowledge, this is the first reported patient in South Korea in whom FAT was monitored using CCEPs to preserve their language function.
FAT is a recently recognized intralobar tract that connects the posterior Broca’s area to the SMA and pre-SMA. FAT is involved in speech and language functions such as verbal fluency, speech initiation and inhibition, sentence production, and lexical decisions.5 Ookawa et al.4 studied the FAT using CCEPs.
The language area of the brain is traditionally localized intraoperatively using an ‘asleep-awake-asleep’ craniotomy,1 which can test all of the multiple networks involved in language function simultaneously. However, this surgery has many limitations such as temporal restrictions, consciousness level, and cooperativeness, because various language tasks need to be performed during the procedure. In contrast, CCEPs can test specific functional networks for language function during surgery without the demands of an extended time awake or cooperation from the patients.
In the present case, functional MRI showed increased BOLD signals adjacent to the border of the tumor located on the SMA, which suggests the presence of FAT. Under general anesthesia, we were able to monitor intraoperative CCEPs with two grids on SMA and IFG without major problems regardless of the level of patient cooperation.
In our study, the N1 latencies of CCEPSMA→IFG were stimulated, and ranged from 30.5 to 33 ms (median, 31.6 ms), which was similar to those found in a previous study (24.4–69.5 ms).5 However, the N1 latency of CCEPIFG→SMA was 3 ms, which were significantly shorter than those reported previously (19.0–48.4 ms). The N1 amplitude range was 103–280 mV (median, 214.8 mV) in the IFG after SMA stimulation, and 13.7–22.3 mV (median, 17.9 mV) in the SMA after IFG stimulation. Similar to the previous study, we also found that CCEPSMA→IFG tended to be larger and have a longer latency than CCEPIFG→SMA. The mechanisms underlying CCEP responses are still unidentified. Previous studies have suggested that the following two mediators can transmit discharges to elicit CCEPs in the adjacent or remote cortices. Direct cortical ES seems to generate both direct and indirect discharges at the stimulation site via (1) a direct cortico-cortical pathway and (2) an indirect cortico-subcorticocortical pathway.2 The short CCEPIFG→SMA latencies in our study therefore suggest that this tract is the direct pathway. On the other hand, the more-delayed response of CCEPSMA→IFG might be transmitted via the indirect pathway.
Since it is performed during time-sensitive surgery, it is necessary to select the optimal CCEPs quickly by setting up the test, selecting the location in advance, and stimulating it rapidly. In our case, CCEP monitoring took about 1 hour, followed by tumor removal surgery taking 1 hour and 25 minutes. During tumor removal, the CCEP waveform remained good and there were no language problems, as would have been expected with intraoperative CCEP monitoring.
This case supports the feasibility and usefulness of CCEPs for intraoperatively detecting language function.
ACKNOWLEDGMENTS
We want to thank Se Keun Oh for his help in preparing the figures. This study was supported by 2014 short training funds of The Korean Society of Clinical Neurophysiology, two grants (2020IE0013 & 2022IE0001) from the Asan Institute for Life Sciences, Asan Medical Center, Seoul, Korea, and a grant from the Korean EEG study Group in 2020.
REFERENCES
1. Kim SM, Kim SH, Seo DW, Lee KW. Intraoperative neurophysiologic monitoring: basic principles and recent update. J Korean Med Sci. 2013; 28:1261–1269.


2. Matsumoto R, Nair DR, LaPresto E, Najm I, Bingaman W, Shibasaki H, et al. Functional connectivity in the human language system: a cortico-cortical evoked potential study. Brain. 2004; 127:2316–2330.


3. Fujii M, Maesawa S, Motomura K, Futamura M, Hayashi Y, Koba I, et al. Intraoperative subcortical mapping of a language-associated deep frontal tract connecting the superior frontal gyrus to Broca’s area in the dominant hemisphere of patients with glioma. J Neurosurg. 2015; 122:1390–1396.


4. Ookawa S, Enatsu R, Kanno A, Ochi S, Akiyama Y, Kobayashi T, et al. Frontal fibers connecting the superior frontal gyrus to Broca area: a corticocortical evoked potential study. World Neurosurg. 2017; 107:239–248.


Fig. 1.
Brain magnetic resonance imaging (MRI) and functional MRI of the patient. Fluid attenuated inversion recovery axial (A) and T2-weighted sagittal (B) MRI revealed a mass in the left supplementary motor area, suggestive of low-grade glioma. Functional MRI (C, D) showed increased blood-oxygen-level-dependent signals in the left frontal cortex adjacent to the mass during verbal language tasks.
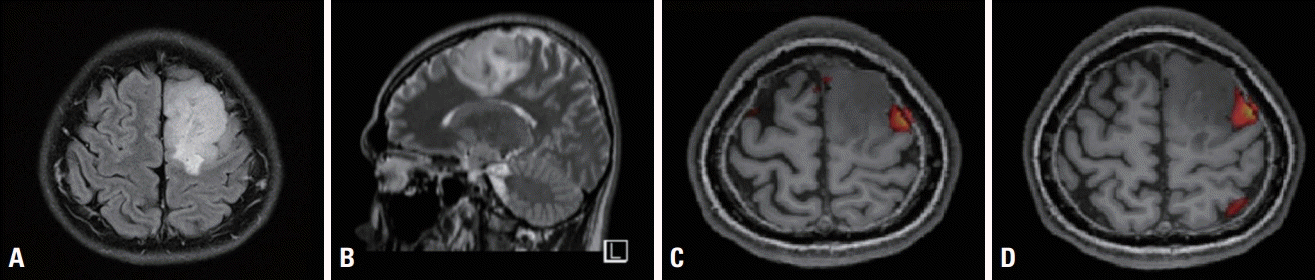
Fig. 2.
Schema of intraoperative CCEPs. (A) Single-pulse electrical stimulation of the SMA (black circle pair) produced CCEPs in inferior frontal gyrus (CCEPSMA→IFG). The latencies and amplitudes of the maximal N1 response (white circles with black rim) in CCEPSMA→IFG were 33 ms and 192 mV. (B) By stimulating corresponding pairs of electrodes on the IFG, the optimal CCEP pathways could be confirmed. The latencies and amplitudes of the maximal N1 response of the main CCEPIFG→SMA were 13 ms and 17.5 mV, which had a shorter latency and were smaller than for CCEPSMA→IFG. CCEPs generally show an early sharp negative potential (N1: peak latency 10–50 ms) and a sequential slow negative potential (N2: peak latency 50–300 ms).6 SMA, supplementary motor area; CCEPs, cortico-cortical evoked potentials; IFG, inferior frontal gyrus.
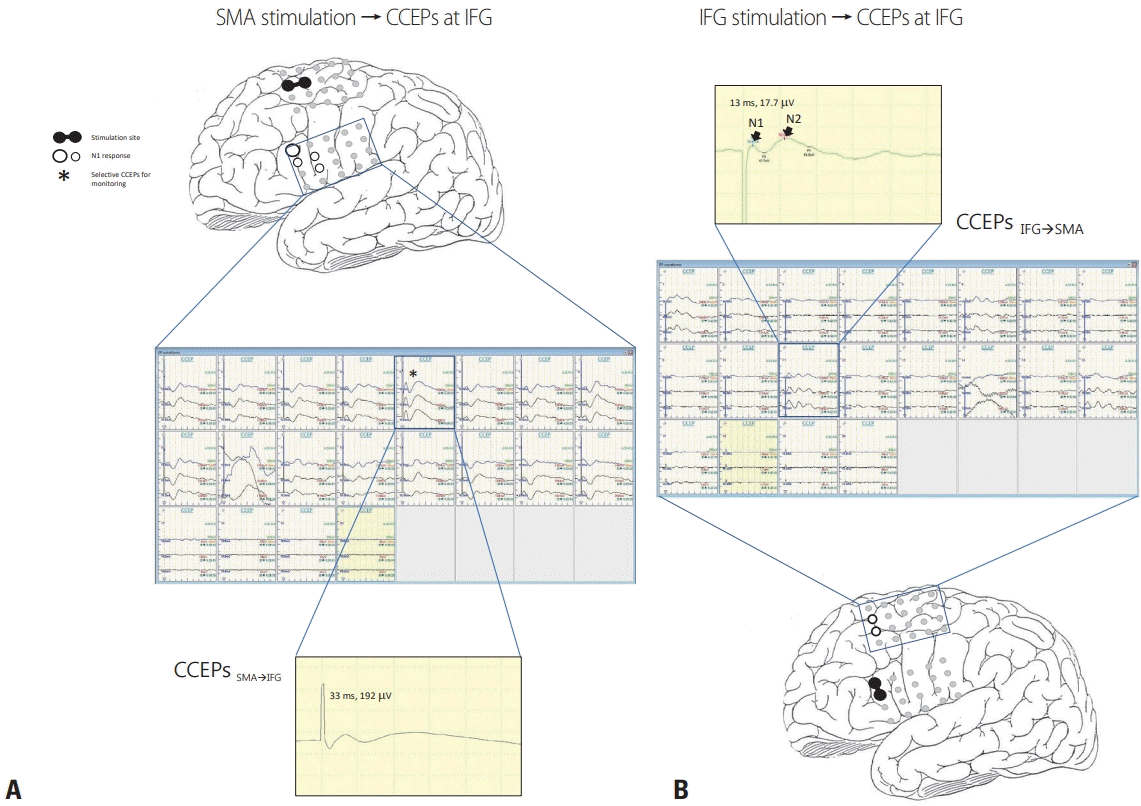