Abstract
Objective
Neuropathic pain causes patients feel indescribable pain. Deep Brain Stimulation (DBS) is one of the treatment methods in neuropathic pain but the action mechanism is still unclear. To study the effect and mechanism of analgesic effects from DBS in neuropathic pain and to enhance the analgesic effect of DBS, we stimulated the ventral posterolateral nucleus (VPL) in rats.
Methods
To observe the effect from VPL stimulation, we established 3 groups : normal group (Normal group), neuropathic pain group (Pain group) and neuropathic pain+DBS group (DBS group). Rats in DBS group subjected to electrical stimulation and the target is VPL.
Results
We observed the behavioral changes by DBS in VPL (VPL-DBS) on neuropathic pain rats. In our study, the pain score which is by conventional test method was effectively decreased. In specific, the time of showing withdrawal response from painful stimulation which is not used measuring method in our animal model was also decreased by DBS.
Conclusion
The VPL is an effective target on pain modulation. Specifically we could demonstrate changes of pain response duration which is not used, and it was also significantly meaningful. We thought that this study would be helpful in understanding the relation between VPL-DBS and neuropathic pain.
Neuropathic pain is one of the most painful diseases for patients. It is an abnormal pain and is induced by direct or indirect nerve damage. Furthermore, the cause of indirect nerve damage is still not clearly identified3). Patients with neuropathic pain feel mechanical allodynia, and it causes patients to feel pain from innocuous mechanical stimulation.
For alleviating neuropathic pain, Deep Brain Stimulation (DBS) is used in clinic. DBS is one of methods for treatment of neurodegenerative diseases by electrical stimulation into the specific brain target1,14,19). DBS modulates the function of the disease-related brain structure, thereby, alleviating or enhancing the symptoms of diseases. DBS would be an effective medication rather than conventional therapies such as drug treatment and neurectomy because drug treatment may lead to drug abuse or drug addiction, and neurectomies raises the risk of tissue damage4,6,8,10,16). There are many studies on DBS for neuropathic pain in clinic. However, there is still lack of studies for comprehending the exact action mechanism of DBS on neuropathic pain. Therefore, more studies on investigating how DBS acts in pain modulation or how neuropathic pain occurs are required in basic research fields. However, it is also difficult for finding basic research studies for understanding neuropathic pain and DBS because the assessment methods and animals models that mimicking neuropathic pain are not varied so far. In animal studies, to measure tactile allodynia, calculating the behavioral response rate or calculate the sensory threshold is the only way to determine onset of neuropathic pain.
In this study, we established an altered behavior test method that has not introduced before. We measured not only the extent of pain but also the duration of pain for evaluating a variety of aspects of neuropathic pain by our method introduced here. From a previous report, the measuring response duration is considered to an inappropriate method for assessing neuropathic pain in our tibial and sural nerve transection (TST) model5). In our study, however, we believe that measuring the duration of showing painful response would be sufficient for assessment of neuropathic pain. In studies of neuropathic pain and its mechanism, it is important to make an animal neuropathic pain model as similar as that of humans because patients with neuropathic pain show case-by-case symptoms and grades13). Because of these differences in pain measuring between humans and experimental animals, it could be important for neuropathic pain studies using animals. Therefore, recording duration of mechanical allodynia in TST model could be meaningful for DBS experiments on neuropathic pain.
15 male Sprague-Dawley rats, weighing 170-190 g, were used. They were housed in groups of five per cage with food and water available ad libitum under a light-dark cycle of 12/12 hr. All experiments were performed during the light phase. Animals were allowed to acclimate for at least a week before surgery and behavioral testing. This study was conducted according to the guidelines of the Ethical Committee of International Association for the Study of Pain21) and the Institution Animal Care and Use Committee of Yonsei University. There are 3 groups in this study : normal group (Normal group, n=3), neuropathic pain group (Pain group, n=5), and neuropathic pain+DBS group (DBS group, n=7).
For inducing neuropathic pain in animals, we used TST method. Rats were anesthetized using phentobarbital sodium (50 mg/kg), and the left sciatic nerve was exposed. Under a surgical microscope (Olympus, Tokyo, Japan), the three major divisions of the sciatic nerve (tibial, sural, and common peroneal nerves) were clearly separated. To generate an efficient neuropathic pain model, the tibial and sural nerves were completely ligated and transected except peroneal nerve. Hemostasis was completed and the cut was closed with muscle and skin sutures11).
Tungsten electrodes (120 µm in diameter) were used for applying electrical stimulation in this study. We made the electrodes using tungsten rods (100 µm in diameter) coated with parylene-C (20 µm in diameter). The tip was exposed in 1 mm long. The coordinates of the ventral posterolateral nucleus (VPL) were AP : -2.2 mm (from bregma), ML : 2.8 mm (right side from bregma), and DV : -6.0 mm (from skull)9,15). The electrodes were firmly secured with glue9,20).
To measure the pain response rate on mechanical allodynia, rats were placed inside acrylic cages (8×10×20 cm) on a wire mesh grid, which allows clinician to access the rat paws. After 30 min of adaptation, innocuous mechanical stimulation was applied with a von Frey filament (8 mN bending force) 10 times to the lateral edge of the left hind paw. We counted the total number of times each animal lifted its ipsilateral hind paw11).
To measure the felt pain duration on mechanical allodynia, rats were placed inside acrylic cages (8×10×20 cm) on top of a wire mesh grid. After 30 min of adaptation, innocuous mechanical stimulation was applied with a von Frey filament (8 mN bending force) 10 times to the lateral edge of the left hind paw. The stimulation interval was minimum 5 minutes. We recorded the time from when the rats started to show a withdrawal response such as lifting, biting, shaking its left hind paw to when the rats laid down its left hind paw, and averaging the results.
The stimulator delivered biphasic pulses in 130 Hz, 20 µs, and 2.5 Volts, and the stimulation parameters were checked using a real time basis with an oscilloscope (HDS1022M-N, Lilliput Technology Co., Ltd, Fujian, China). Behavior tests were carried out at three time points : first was at 1 hour before stimulation in order to measure baseline levels (Pre); second was at 1 hour after the start of stimulation, and the stimulation was applied throughout the behavior test (ON); and the last was at 1 hour after stimulation was aborted (OFF), as a verification phase (Fig. 1).
To verify the location of electrode, we prepared the electrodes which were soaked in fluorescence dye (D282, Invitrogen, Grand Island, NY, USA). After electrode insertion, rats were purfused with normal saline and fixed with 4% paraformaldehyde in PBS (pH 7.4). The brain was carefully removed and prepared for frozen section. Coronal sections of 50 um were obtained using a microtome with deep freezer. Fluorescence sample images and normal sample images were obtained using a fluorescence microscope (Olympus, Tokyo, Japan), and each images from a tissue sample were merged using Adobe photoshop (Adobe systems, San Jose, CA, USA).
Data are reported as means±SEM. Statistical analysis was performed using paired t-tested for comparing the behavioral changes by DBS. In comparing the difference between each groups, we performed the Kruskal-Wallis one-way analysis of variance (ANOVA) was used, followed by Tukey's post hoc and Fisher's least significant difference (LSD) post hoc comparisons. The p-values of <0.001 and <0.05 were considered significant. All statistical analyses were performed using SPSS (version 11.5, SPSS Inc., Chicago, IL, USA).
We inserted the microelectrode into VPL. From fluorescence image, we verified that the electrode was exactly located in VPL (Fig. 2). After electrode insertion, there were no abnormal behavioral changes or morphological changes in the DBS group.
We carried out DBS for 15 days post operation in the DBS group. Behavior tests for measuring pain was carried out at 3 time points : first was at 1 hour before stimulation into order to measure a baseline; second was at 1 hour after the start of stimulation, and stimulation was applied throughout the behavior test; and the last was 1 hour after stimulation was aborted. In the behavior test, we measured the rate of demonstrating a pain response and the duration of showing a pain response. After 15 days upon follow up, the baseline pain response rate score was 9.8-10 (10 being the maximum value) and the duration of showing a pain response was 10-17 seconds. During DBS, the pain score decreased to 4.8-6.8 and duration also decreased to 1.4-6.8 seconds. In the stimulation phase, pain score and duration decreased in comparison to those of the baseline. After abortion of stimulation, the pain score increased to 9.4-10, and duration also increased to 7.7-21.8 seconds. After stimulation, the pain response score and duration reverted to their baseline value (Fig. 3).
After the stimulation, we compared the effects from DBS and that of Normal and Pain groups. In the Pain group, the pain score and duration were significantly increased when compared with those of the Normal group (score : p<0.05, duration : p<0.001, one-way ANOVA test using Tukey as post hoc). In the DBS group, the score was decreased at 40% to that of the Pain group (p<0.001, one-way ANOVA test using Tukey as post hoc), and duration was decreased at 75% to that of the Pain group (p<0.001, one-way ANOVA test using Tukey as post hoc) (Fig. 4).
In the stimulation phase, the pain response rate and duration were decreased by DBS in DBS group. We compared the pain response score and duration at each time point in the stimulation phase to decide whether stimulation decreased pain directly.
After measuring the pain response rate, the baseline score in DBS group was 9.98±0.01 (Fig. 5A, Pre). During stimulation, the score in DBS group decreased to 6.08±0.32 (Fig. 5A, ON). Upon abortion of stimulation, the score in DBS group reversed to 9.75±0.07 (Fig. 5A, OFF).
In measuring duration, the baseline score in DBS group was 16.20±0.81 seconds (Fig. 5B, Pre). During stimulation, the duration in DBS group decreased to 2.92±0.28 seconds (Fig. 5B, ON). After abortion of stimulation, the duration in DBS group reversed to 17.18±1.21 seconds (Fig. 5B, OFF).
What is commonly seen from this result is that both the pain response rate and felt pain duration decreased with VPL stimulation. Furthermore, it reversed to baseline values when stimulation ceased. Especially, the ratio of the decrease in duration is higher than that of the pain score by VPL stimulation.
In scientific studies using experimental animals, there are many types of animal disease models that mimicking that of humans. However, many limitations are still there. In this study, we designed an animal study that can give us more detailed information in neuropathic pain.
First of all, we confirmed the location of every stimulation electrodes in animals for verifying target insertion. After electrical stimulation phase, we took brain samples from animals, and confirmed that each electrode was located at the intended target, VPL. The electrodes were precisely inserted in VPL (Fig. 1), and there were no abnormal behavioral, morphological and histological changes. Hence, we decided that the electrical stimulation was applied to the right place and was appropriately applied to animals.
Second, we focused on the effect of DBS in neuropathic pain using conventional experiment method11). VPL-DBS effectively decreased pain score, and it gradually reversed when electrical stimulation was ceased. In electrical stimulation, pain score got started dropping after the electrical stimulation started, and reached maximum level within 30 minutes (data not shown). Furthermore, this analgesic effect lasted for a while and it gradually reversed to baseline levels in 30 minutes after stimulation ceased. It is similar with another latest report12). Therefore, we think that it takes several minutes for the patients or animals with neuropathic pain for having maximum analgesic effect by electrical stimulation, but once the stimulation applied, the analgesic effect is stable.
Third, in our animal study, we had a sense of difference in time of showing withdrawal response by electrical stimulation. Therefore we measured the duration of showing withdrawal response from mechanical allodynia. Practically, previous studies on establishing optimal behavioral test methods in each animal neuropathic pain models says that measuring the latency on mechanical stimulation using von Frey is not suitable in our TST model5). They said that pinprick test is an appropriate method for measuring pain response duration for measuring hyperalgesia, not allodynia. Furthermore, they said that this was not significant in TST models11). However, in our study, we measured the time of showing withdrawal response. Procedures were the same as that of pinprick tests2), and to maintain the stimulation force uniformly, we used only one von Frey hair which has 8 mN of bending force, and this von Frey hair is also used in conventional scoring method9,11). The main difference between ours and that of the pinprick is that von Frey hair was used or not. In our study, the duration of showing pain response decreased by VPL-DBS, and it is well correlated with results in changes of pain score.
In several electrophysiological studies, when action potential is induced by peripheral nerve stimulation, the week excitation lasts for a long time7). We think, therefore, this could be an explanation of lasting excitation by painful stimulation. Thus, we considered VPL-DBS as a possible modulator on neuropathic pain treatment in level (or threshold) of pain and extent (similarly enduring time) of pain.
We considered that the changes of painful response duration were resulted from functional role of VPL. It is likely to consider that there are the mechanical and emotional parts in pain18). In a clinical report, they reported a patient who had DBS surgery on cold pain. The DBS stimulation, finally, failed by electrode failure but the patient wanted to turn his stimulator on. They said that it could be by placebo effect17). Therefore, we thought that pain is a very complicate phenomenon, and our behavior test method could be helpful on our sight of neuropathic pain.
As we described above, animal studies have many limitations in understanding the relation between disease and patients' pain by disease. Therefore, it is important to develop various animal disease models that allow researchers to study in detail by suggesting various criteria on pain diagnosis. In this respect, we think that our altered measuring method of the duration has an advantage in pain study that could be gives us more clues in understanding neuropathic pain by measuring not only response duration but measuring conventional one.
In our study, there is a limitation; it requires more evidences in describing the duration reduction by VPL-DBS because it could not be stated that the pain feeling duration was decreased by VPL-DBS only. As described above, there are many possible signal pathways or components of neuropathic pain. Therefore, we are now carrying on further studies that how VPL-DBS can reduce pain response rate and duration using electrophysiology and imaging studies.
In summary, VPL-DBS is effective in neuropathic pain. Specifically, it reduces not only the pain response score but also duration of showing painful response in neuropathic pain. Moreover, the method for measuring the duration of feeling pain would be beneficial to experimenters in animal studies.
Acknowledgements
This study was financially supported by the grant from the Industrial Source Technology Development Program (no. 10033812 and 10033657) and Global Frontier Project (SIRC-2011-0031866) of the Ministry of Science, ICT and Future Planning of Korea.
We also would like to thank Kyung Won Chang for his laboratory assistance.
References
1. Blomstedt P, Sandvik U, Fytagoridis A, Tisch S. The posterior subthalamic area in the treatment of movement disorders : past, present, and future. Neurosurgery. 2009; 64:1029–1038. discussion 1038-1042. PMID: 19487881.
2. Choi Y, Yoon YW, Na HS, Kim SH, Chung JM. Behavioral signs of ongoing pain and cold allodynia in a rat model of neuropathic pain. Pain. 1994; 59:369–376. PMID: 7708411.


3. DaSilva AF, Granziera C, Snyder J, Hadjikhani N. Thickening in the somatosensory cortex of patients with migraine. Neurology. 2007; 69:1990–1995. PMID: 18025393.


4. Deniau JM, Degos B, Bosch C, Maurice N. Deep brain stimulation mechanisms : beyond the concept of local functional inhibition. Eur J Neurosci. 2010; 32:1080–1091. PMID: 21039947.


5. Dowdall T, Robinson I, Meert TF. Comparison of five different rat models of peripheral nerve injury. Pharmacol Biochem Behav. 2005; 80:93–108. PMID: 15652385.


6. Gubellini P, Salin P, Kerkerian-Le Goff L, Baunez C. Deep brain stimulation in neurological diseases and experimental models : from molecule to complex behavior. Prog Neurobiol. 2009; 89:79–123. PMID: 19559747.


7. Hubscher CH, Johnson RD. Chronic spinal cord injury induced changes in the responses of thalamic neurons. Exp Neurol. 2006; 197:177–188. PMID: 16266704.


8. Kahane P, Depaulis A. Deep brain stimulation in epilepsy : what is next. Curr Opin Neurol. 2010; 23:177–182. PMID: 20125010.
9. Kim J, Kim J, Min KS, Lee SE, Kim SJ, Chang JW. VPL-DBS on neuropathic pain rat model is effective in mechanical allodynia than cold allodynia. Neurol Sci. 2012; 33:1265–1270. PMID: 22562402.


10. Kringelbach ML, Jenkinson N, Green AL, Owen SL, Hansen PC, Cornelissen PL. Deep brain stimulation for chronic pain investigated with magnetoencephalography. Neuroreport. 2007; 18:223–228. PMID: 17314661.


11. Lee BH, Won R, Baik EJ, Lee SH, Moon CH. An animal model of neuropathic pain employing injury to the sciatic nerve branches. Neuroreport. 2000; 11:657–661. PMID: 10757496.


12. Lucas JM, Ji Y, Masri R. Motor cortex stimulation reduces hyperalgesia in an animal model of central pain. Pain. 2011; 152:1398–1407. PMID: 21396776.


13. Merskey H, Bogduk N. Classification of chronic pain : Descriptions of chronic pain syndromes and definitions of pain terms. ed 2. Seattle: IASP Press;1994.
14. Nandi D, Aziz TZ. Deep brain stimulation in the management of neuropathic pain and multiple sclerosis tremor. J Clin Neurophysiol. 2004; 21:31–39. PMID: 15097292.


15. Paxinos G, Watson C. The Rat Brain in Stereotaxic Coordinates. ed 4. New York: Academic Press;1988.
16. Perlmutter JS, Mink JW. Deep brain stimulation. Annu Rev Neurosci. 2006; 29:229–257. PMID: 16776585.


17. Pickering AE, Thornton SR, Love-Jones SJ, Steeds C, Patel NK. Analgesia in conjunction with normalisation of thermal sensation following deep brain stimulation for central post-stroke pain. Pain. 2009; 147:299–304. PMID: 19833434.


18. Seminowicz DA, Laferriere AL, Millecamps M, Yu JS, Coderre TJ. MRI structural brain changes associated with sensory and emotional function in a rat model of long-term neuropathic pain. Neuroimage. 2009; 47:1007–1014. PMID: 19497372.


19. Shirvalkar P, Seth M, Schiff ND, Herrera DG. Cognitive enhancement with central thalamic electrical stimulation. Proc Natl Acad Sci U S A. 2006; 103:17007–17012. PMID: 17065322.


20. Wu C, Wais M, Sheppy E, del Campo M, Zhang L. A glue-based, screw-free method for implantation of intra-cranial electrodes in young mice. J Neurosci Methods. 2008; 171:126–131. PMID: 18420280.


21. Zimmermann M. Ethical guidelines for investigations of experimental pain in conscious animals. Pain. 1983; 16:109–110. PMID: 6877845.


Fig. 2
Confirmation of location of electrode insertion. The position of electrode is verified by brain slice samples. Electrode insertion track glows on brain slice (a thick white line means electrode).

Fig. 3
The results from VPL-DBS for 15 days post operation. A : Pain response rate score. B : Duration of felt pain. During stimulation, pain response score and duration were reduced compared with those before stimulation, and it reversed after stimulation was aborted. ◊ : before stimulation, ▪ : during stimulation, ▵ : 1 hour after stimulation was aborted. VPL : ventral posterolateral nucleus, DBS : Deep Brain Stimulation.
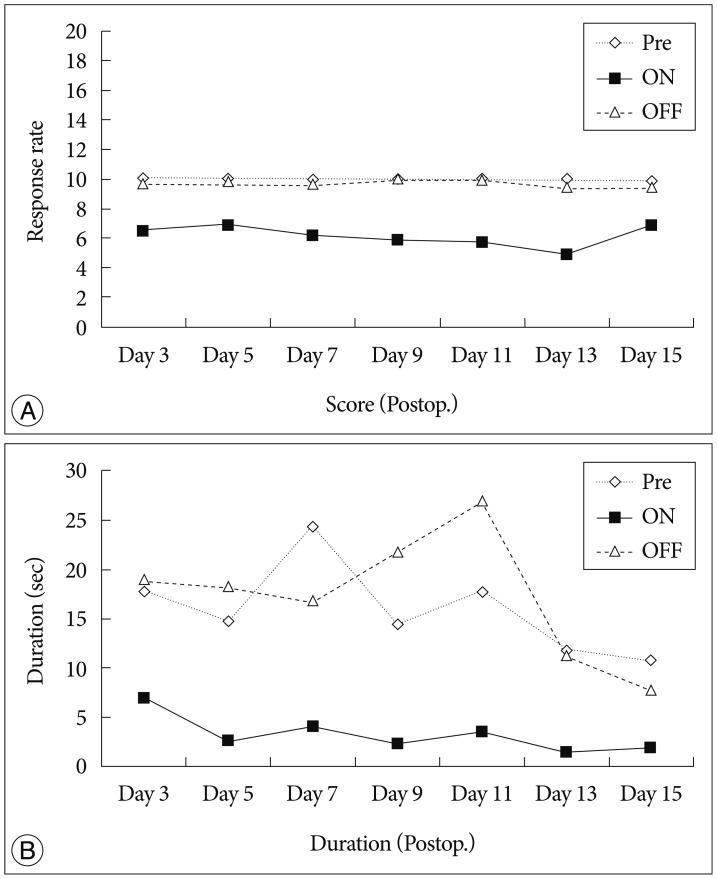
Fig. 4
The results from VPL-DBS. A : Pain response rate. B : Duration of felt pain. In the neuropathic pain group, pain score and duration were increased compared with that of the normal group. In the DBS group, score and duration was decreased compared with that of the neuropathic pain group (**p<0.05, ***p<0.001, one-way ANOVA test using Tukey post hoc). Normal : normal group, Pain : neuropathic pain group, DBS : DBS group. VPL : ventral posterolateral nucleus, DBS : Deep Brain Stimulation, ANOVA : analysis of variance.
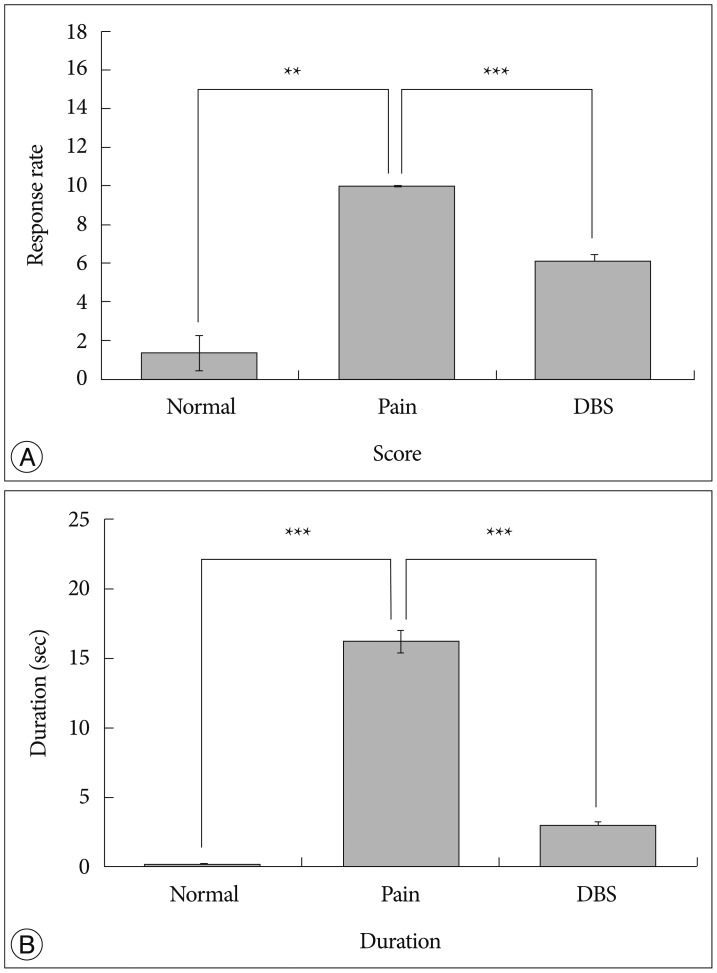
Fig. 5
Verification of DBS effect. A : Pain response rate. B : Duration of felt pain. "Pre" represents before stimulation, "ON" represents during stimulation and "OFF" represents 1 hour after stimulation was aborted. Pain was reduced by VPL-DBS, and it reversed to baseline levels when stimulation was aborted (***p<0.001, using paired t-test). VPL : ventral posterolateral nucleus, DBS : Deep Brain Stimulation.
