Abstract
Objective
The potassium disturbance associated with thiopental continuous infusion in neurosurgical patients is well known. However, the effect of propofol continuous infusion on serum potassium levels has not been investigated extensively.
Methods
We reviewed the medical records of 60 consecutive patients who received coma therapy or deep sedation for intracranial pressure control using either thiopental or propofol between January 2010 and January 2012.
Results
The overall incidence of hypokalemia (K<3.5 mmol/L) was comparable between thiopental and propofol groups (89.2% vs. 82.6%). But, the incidence of moderate to severe hypokalemia (K<3.0 mmol/L) was significantly higher in thiopental group (51.4% vs. 13.0%, p=0.003). The lowest potassium level (2.9 mmol/L vs. 3.2 mmol/L, p=0.020) was lower in thiopental group. The patients in the thiopental group required greater potassium replacement than the propofol group patients (0.08 mmol/kg/h vs. 0.02 mmol/kg/h, p<0.001). On multivariate analysis, thiopental [odds ratio, 95% confidence interval, 7.31 (1.78-27.81); p=0.005] was associated with moderate to severe hypokalemia during continuous infusion. The incidence of rebound hyperkalemia (K>5.0 mmol/L, 32.4% vs. 4.3%, p=0.010) and the peak potassium concentration (4.8 mmol/L vs. 4.2 mmol/L, p=0.037) after the cessation of therapy were higher in thiopental group. On multivariate analysis, thiopental [8.82 (1.00-77.81); p=0.049] and duration of continuous infusion [1.02 (1.00-1.04); p=0.016] were associated with rebound hyperkalemia once therapy was discontinued.
Barbiturates are widely used to control refractory intracranial hypertension. They indirectly lower intracranial pressure (ICP) via a coupled reduction in cerebral metabolic rate of oxygen (CMRO2) and cerebral blood flow. The maximum decrease (roughly 50%) in CMRO2 occurs when the electroencephalographic readings become isoelectric2,17). Barbiturate therapy, however, is associated with adverse effects, including hypotension and immunosuppression24). Additionally, barbiturates can induce hypokalemia after the induction of coma therapy and cause rebound hyperkalemia after the cessation of therapy4,16,19).
The incidence of hypokalemia after thiopental induction ranges between 82% and 89.4%1,18,19). A case series reported that 34% of patients had rebound hyperkalemia after stopping thiopental coma therapy18). There have also been several reports of serious adverse events due to hypokalemia and rebound hyperkalemia16,25). Dyskalemia is life-threatening and a major obstacle to barbiturate coma therapy.
Propofol has been demonstrated to be an effective drug for the regulation of ICP10,22). It decreases ICP in normal subjects and as well as in patients with an elevated ICP10). It reduces the cerebral metabolic rate by 40% in a dose-dependent fashion and decreases cerebral blood flow by metabolism-flow coupling26,27). Propofol is known to cause hypotension, hypertriglyceridemia, and propofol-related infusion syndrome (PRIS)13,20). Unlike thiopental, however, research has not fully evaluated the effect of propofol continuous infusion on serum potassium levels, especially when used for the regulation of ICP.
The aim of this study was to determine the effect of thiopental and propofol continuous infusion on serum potassium levels when used for ICP control.
This retrospective study was approved by the Institutional Review Board of Seoul National University Hospital (IRB no. H-1307-047-504) and was in accordance with the Declaration of Helsinki. Patients who were consecutively admitted to the surgical intensive care unit (ICU) to receive coma therapy for the treatment of refractory intracranial hypertension or deep sedation for the prevention of a further increase in ICP from January 2010 to January 2012 were included. Patients without ICP monitoring were excluded from the analysis.
In this study, head elevation, deep sedation, analgesia, use of osmotic agents, and cerebrospinal fluid drainage were used as the first-tier methods to control ICP in patients with elevated ICP. Coma therapy was used when the first-tier therapies failed to reduce ICP to 25 mm Hg.
The patients received one of two regimens for the regulation of ICP, including thiopental (n=37) or propofol (n=23). In those using thiopental continuous infusions, a loading dose (250 mg) was administrated for about 30 min, followed by a continuous infusion of 3-7 mg/kg/h. In those using propofol continuous infusions, a loading dose (100-150 mg) was administrated for about 30 min, followed by a continuous infusion of 3-7 mg/kg/h. During both treatments, the cerebral perfusion pressure was maintained at 60-80 mm Hg. Once no further increases in ICP from baseline were detected for 9-18 h in patients with a baseline ICP of <25 mm Hg, in whom deep sedation was needed for ICP control, or if the ICP decreased to ≤24 mm Hg and the decrease in ICP was maintained for 24-36 h in patients with refractory intracranial hypertension, both treatments were slowly weaned over 3-12 h.
Hypokalemia (defined as a serum potassium level <3.5 mmol/L) was managed by potassium replacement (target serum potassium >3.0 mmol/L), while hyperkalemia (defined as a serum potassium level >5.0 mmol/L) was managed with conservative methods such as sodium bicarbonate, insulin, and diuretics. Mild hypokalemia was defined as a serum potassium level of 3.0-3.5 mmol/L. Moderate hypokalemia was defined as a serum potassium level of 2.5-3.0 mmol/L and severe hypokalemia was defined as a level less than 2.5 mmol/L. Hypothermia was defined as a core temperature <35℃. Refractory intracranial hypertension was defined as ICP >25 mm Hg despite the use of first-tier therapies for ICP control.
In all patients, serum potassium was measured before therapy. Serum potassium was measured with various intervals (3-8 h) during and after therapy. The same protocol was applied to both groups. The primary outcome in this study was the incidence of hypokalemia during thiopental or propofol continuous infusion. The secondary outcome was the incidence of hyperkalemia after the cessation of thiopental or propofol therapy.
Electronic medical records were used to extract and analyze the following parameters : patient's age, sex, past medical history, duration and dose of both therapies, serum potassium level, ICP, Glasgow Coma Scale score prior to therapy, acute physiology and chronic health evaluation II (APACHE II) score, light reflex prior to therapy, the length of ICU stay, ICU mortality, and duration of mechanical ventilation. Variables that possibly contributed to dyskalemia, including urine output, body temperature, vasopressors, inotropes, insulin, mannitol, diuretics, and transfusion, were also extracted and analyzed.
Statistical analyses were performed using SPSS 19.0 (SPSS Inc., Chicago, IL, USA). All numerical data are summarized as the mean (standard deviation) or median (interquartile range). Categorical data were compared using the chi-square or Fisher's exact test, if appropriate. Student's t-test was used to compare continuous data. To determine risk factors for hypokalemia and rebound hyperkalemia, univariate analyses were performed and only variables with p<0.2 were entered into the binary logistic analysis with a forward stepwise condition. All tests were two-tailed; p<0.05 was considered statistically significant.
Seventy-one patients were admitted to the surgical ICU during the study period for coma therapy to control refractory intracranial hypertension or for deep sedation to prevent a further increase in ICP. A total of 11 patients without ICP monitoring were excluded; thus, 60 patients were included in this study. Thiopental was used in 37 patients and propofol was used in 23 patients.
There were no statistically significant differences between the two patient groups with respect to age, sex, weight, APACHE II score, neurologic status before therapy, ICP before therapy, and the number of patients having refractory intracranial hypertension (Table 1). A total of 23 (62.2%) patients in the thiopental group had a vascular problem and 15 (65.2%) patients in the propofol group had a tumor.
The overall changes in serum potassium level during thiopental or propofol continuous infusion are shown in Fig. 1. The initial serum potassium level prior to therapy (3.9±0.6 mmol/L vs. 3.9±0.5 mmol/L, p=0.800), total dose administered (5.3±1.5 mg/kg/h vs. 5.3±1.8 mg/kg/h), and therapy duration (60.2±46.3 h vs. 44.1±32.3 h, p=0.150) were comparable between thiopental and propofol group. A total of 11 patients had initial serum potassium level less than 3.5 mmol/L (8 in thiopental group vs. 3 in propofol group, p=0.506). The incidence of hypokalemia following the induction of therapy was not significantly differed in the thiopental group and propofol group (Table 2). Meanwhile, the incidence of moderate to severe hypokalemia following the induction of therapy was significantly higher in the thiopental group than in the propofol group (51.4% vs. 13.0%, p=0.003). The average lowest serum potassium level was lower in the thiopental group than in the propofol group (2.9±0.6 mmol/L vs. 3.2±0.4 mmol/L, p=0.020). Also, the average lowest potassium level in patients showing hypokalemia was lower in the thiopental group than in the propofol group (p=0.009). The time to the lowest serum potassium concentration was 21.7±13.4 h in thiopental group and 15.2±12.9 h in propofol group. The patients in the thiopental group required greater potassium replacement than the propofol group patients (p<0.001).
When therapy was discontinued, the serum potassium levels were 3.5±0.8 mmol/L in the thiopental group and 3.6±0.5 mmol/L in the propofol group (Fig. 2). After continuous infusion was stopped, twelve patients in the thiopental group and one patient in propofol group experienced rebound hyperkalemia (p=0.010) (Table 2). Two patients in the thiopental group suffered from ventricular fibrillation (potassium level 8.4 mmol/L) and non-sustained ventricular tachycardia (potassium level 7.4 mmol/L) due to hyperkalemia. The average peak serum potassium level was higher in the thiopental group than in the propofol group (4.8±1.1 mmol/L vs. 4.2±1.1 mmol/L, p=0.038). But, the average peak serum potassium level in patients showing hyperkalemia was comparable in both groups. The time to the peak serum potassium concentration was 39.3±24.8 h in thiopental group.
Clinical outcomes such as ICU mortality, duration of the ICU stay, and the duration of mechanical ventilation were similar in both groups (Table 3).
Based on a univariate analysis, only urine output [odds ratio, 95% confidence interval, 2.31 (1.06-5.02); p=0.035] was associated with hypokalemia during continuous infusion. Thiopental and refractory intracranial hypertension were associated with moderate to severe hypokalemia during continuous infusion in univariate analysis (Table 4). Only thiopental [odds ratio, 95% confidence interval, 7.04 (1.78-27.81); p=0.005] was predictive of moderate to severe hypokalemia in binary logistic regression analysis after adjusting for the type of injury, refractory intracranial hypertension, beta agonist, hypothermia. A univariate analysis for rebound hyperkalemia development after stopping continuous infusion is shown in Table 5. According to a binary logistic regression analysis, thiopental [8.82 (1.00-77.81); p=0.049] and duration of continuous infusion [1.02 (1.00-1.04); p=0.016] were associated with rebound hyperkalemia after adjusting for the type of injury, the amount of potassium replaced during the therapy, beta agonist, mannitol, hypothermia.
The present study demonstrates that the incidence of hypokalemia was similar in patients receiving thiopental continuous infusion and in patients receiving propofol continuous infusion. However, the incidence of moderate to severe hypokalemia and rebound hyperkalemia were significantly higher in the thiopental group patients. Only thiopental was associated with moderate to severe hypokalemia and rebound hyperkalemia.
In a recent study, the incidence of hypokalemia was 7.5% in patients admitted to an ICU after brain surgery5), whereas the incidence of hypokalemia was 80-90% in patients receiving thiopental coma therapy19,24). In the present study, hypokalemia developed in 89.2% of patients receiving thiopental therapy which was comparable with what has been previously reported. Interestingly, this study showed similar incidence of hypokalemia in thiopental and propofol-infused patients. However, the incidence of moderate to severe hypokalemia was lower in propofol-infused patients than in thiopental-infused patients.
Propofol has been demonstrated to be an effective drug for the regulation of ICP10,22). It has a short elimination half-life of <1 h and permits frequent and rapid titration. Also, propofol offers faster, more predictable awakenings, and earlier extubation than thiopental or other sedative agents7,9). Thus, it may be a useful agent for serial neurological examinations in patients with intracranial hypertension. However, the effect of propofol administration on serum potassium levels has not been widely researched, and results are inconsistent. For example, a previous study reported that the serum potassium level fell by 0.04 mmol/L at 5 min after induction with a propofol bolus injection (2.5 mg/kg), but recovered to the pre-induction level within 10 min15). In contrast, a previous case report showed that a sudden cardiac arrest due to hyperkalemia occurred after propofol single administration14). Hyperkalemia has been reported as one of clinical manifestations in PRIS patients12,28). PRIS is commonly associated with rhabdomyolysis, and hyperkalemia may have been due to rhabdomyolysis12). Propofol can decrease beta-adrenoceptor responsiveness29). Therefore, it may block the effect of beta agonists on the intracellular potassium shift. In this study, when propofol was continuously administered for ICP control, hypokalemia was common but the severity was low. We think that other factors rather than propofol caused hypokalemia in propofol continuous infusion group.
There are several possible causes of hypokalemia in neurosurgical patients. First, it is well known that hypokalemia is related to the sympathetic stress response and catecholamine surge in patients with a brain injury3,21,23). This study also showed that refractory intracranial hypertension was associated with moderate to severe hypokalemia in univariate analysis, although it was not an independent factor on binary logistic analysis. Second, hypokalemia can be induced by urinary potassium loss due to mannitol, diuretics or central diabetes insipidus. Our results also showed that urine output during continuous therapy was associated with hypokalemia. Urinary potassium loss and its combination with thiopental infusion can result in more severe hypokalemia. Third, vasopressor therapy, an insulin infusion, and hypothermia can also cause hypokalemia. But, these confounding variables were not associated with hypokalemia in this study.
In the present study, patients receiving thiopental had greater potassium supplementation than those receiving propofol during the infusion therapies. However, the lowest serum potassium level was still significantly lower in patients receiving thiopental and the incidence of moderate to severe hypokalemia was significantly higher in this group. Such finding confirms the association between thiopental and hypokalemia. There are several mechanisms that may explain the hypokalemia development in patients receiving thiopental continuous infusion. First, thiopental inhibits the voltage-dependent potassium current via sodium/potassium ATPase activity and induces the intracellular sequestration of potassium8). Second, thiopental inhibits phosphofructokinase and reduces the intracellular production of pyruvate and lactate, thereby increasing the intracellular pH and inducing an extracellular to intracellular potassium shift6).
The potassium disturbance associated with thiopental follows a relatively consistent biphasic pattern consisting of hypokalemia after induction and hyperkalemia after the cessation of therapy4,19). In the previous studies, rebound hyperkalemia occurred within 6 h with sudden cessation, but occurred 31 h after cessation with gradual weaning of over 12-24 h4,19). It is believed that the intracellular accumulation of potassium during thiopental therapy causes rebound hyperkalemia after the cessation of therapy11). Our study showed that the incidence of rebound hyperkalemia was significantly higher in thiopental-infused patients than in propofol-infused patients. Thiopental and the duration of therapy were significant predictors of rebound hyperkalemia after the cessation of therapy.
Dyskalemia associated with barbiturates can be life-threatening4,16,18). Both hypokalemia and hyperkalemia are potentially fatal. In this study, one patient in the thiopental group suffered from ventricular fibrillation and another patient suffered from non-sustained ventricular tachycardia caused by rebound hyperkalemia. When thiopental is used to control ICP, clinicians should be aware of dyskalemia associated with barbiturate therapy, and serum potassium levels should be closely monitored.
There were several limitations to this study. Since this study was performed retrospectively, data collection was a limitation. The sample size was small. The type of surgery was also heterogeneous. But, our results showed that the type of surgery was not associated with hypokalemia development on the binary logistic analysis. In this study, we focused on serum potassium disturbances during and after the infusion of thiopental or propofol instead of clinical outcomes, because patients with refractory intracranial hypertension as well as patients with a baseline ICP of <25 mm Hg were included in the inclusion criteria.
When continuous infusion was used to control intracranial pressure, propofol was less frequently associated with moderate to severe hypokalemia after induction and rebound hyperkalemia after cessation of therapy than thiopental. Whenever thiopental is used to control intracranial hypertension, serum potassium levels should be closely monitored with at least 6-h intervals.
References
1. An HS, Cho BM, Kang JH, Kim MK, Oh SM, Park SH. Efficacy of low dose barbiturate coma therapy for the patients with intractable intracranial hypertension using the bispectral index monitoring. J Korean Neurosurg Soc. 2010; 47:252–257. PMID: 20461164.


2. Astrup J, Rosenørn J, Cold GE, Bendtsen A, Møller Sørensen P. Minimum cerebral blood flow and metabolism during craniotomy. Effect of thiopental loading. Acta Anaesthesiol Scand. 1984; 28:478–481. PMID: 6496004.


3. Beal AL, Scheltema KE, Beilman GJ, Deuser WE. Hypokalemia following trauma. Shock. 2002; 18:107–110. PMID: 12166770.


4. Cairns CJ, Thomas B, Fletcher S, Parr MJ, Finfer SR. Life-threatening hyperkalaemia following therapeutic barbiturate coma. Intensive Care Med. 2002; 28:1357–1360. PMID: 12209290.


5. Capparelli FJ, Abello M, Patricio Maskin L, Arista E, Hlavnicka A, Diaz MF, et al. QTc prolongation after brain surgery. Neurol Res. 2013; 35:159–162. PMID: 23452578.


6. Carlsson C, Harp JR, Siesjö BK. Metabolic changes in the cerebral cortex of the rat induced by intravenous pentothalsodium. Acta Anaesthesiol Scand Suppl. 1975; 57:1–17. PMID: 128981.


7. Fogarty DJ, McCleane GJ. Propofol and serum potassium. Anaesthesia. 1992; 47:63–64. PMID: 1536410.


8. Friederich P, Urban BW. Interaction of intravenous anesthetics with human neuronal potassium currents in relation to clinical concentrations. Anesthesiology. 1999; 91:1853–1860. PMID: 10598630.


9. Hall RI, Sandham D, Cardinal P, Tweeddale M, Moher D, Wang X, et al. Propofol vs midazolam for ICU sedation : a Canadian multicenter randomized trial. Chest. 2001; 119:1151–1159. PMID: 11296183.
10. Hartung HJ. [Intracranial pressure in patients with craniocerebral trauma after administration of propofol and thiopental]. Anaesthesist. 1987; 36:285–287. PMID: 3498377.
11. Jung JY, Lee C, Ro H, Kim HS, Joo KW, Kim Y, et al. Sequential occurrence of life-threatening hypokalemia and rebound hyperkalemia associated with barbiturate coma therapy. Clin Nephrol. 2009; 71:333–337. PMID: 19281748.


13. Kelly DF, Goodale DB, Williams J, Herr DL, Chappell ET, Rosner MJ, et al. Propofol in the treatment of moderate and severe head injury : a randomized, prospective double-blinded pilot trial. J Neurosurg. 1999; 90:1042–1052. PMID: 10350250.


14. Lee JH, Ko YS, Shin HJ, Yi JH, Han SW, Kim HJ. Is There a Relationship between Hyperkalemia and Propofol? Electrolyte Blood Press. 2011; 9:27–31. PMID: 21998604.


15. Lee JI, Kim YZ, Kim JS, Choi YC, Kim KH. Clinical comparison between barbiturate and propofol coma therapy in the patients with severe traumatic brain injury. J Korean Neurotraumatol Soc. 2008; 4:24–30.


16. Machata AM, Gonano C, Bîrsan T, Zimpfer M, Spiss CK. Rare but dangerous adverse effects of propofol and thiopental in intensive care. J Trauma. 2005; 58:643–645. PMID: 15761368.


17. Michenfelder JD. The interdependency of cerebral functional and metabolic effects following massive doses of thiopental in the dog. Anesthesiology. 1974; 41:231–236. PMID: 4854910.


18. Neil MJ, Dale MC. Hypokalaemia with severe rebound hyperkalaemia after therapeutic barbiturate coma. Anesth Analg. 2009; 108:1867–1868. PMID: 19448214.


19. Ng SY, Chin KJ, Kwek TK. Dyskalaemia associated with thiopentone barbiturate coma for refractory intracranial hypertension : a case series. Intensive Care Med. 2011; 37:1285–1289. PMID: 21567112.


20. Otterspoor LC, Kalkman CJ, Cremer OL. Update on the propofol infusion syndrome in ICU management of patients with head injury. Curr Opin Anaesthesiol. 2008; 21:544–551. PMID: 18784477.


21. Pomeranz S, Constantini S, Rappaport ZH. Hypokalaemia in severe head trauma. Acta Neurochir (Wien). 1989; 97:62–66. PMID: 2718795.


22. Ravussin P, Guinard JP, Ralley F, Thorin D. Effect of propofol on cerebrospinal fluid pressure and cerebral perfusion pressure in patients undergoing craniotomy. Anaesthesia. 1988; 43(Suppl):37–41. PMID: 3259094.


23. Schaefer M, Link J, Hannemann L, Rudolph KH. Excessive hypokalemia and hyperkalemia following head injury. Intensive Care Med. 1995; 21:235–237. PMID: 7790611.


24. Schalén W, Messeter K, Nordström CH. Complications and side effects during thiopentone therapy in patients with severe head injuries. Acta Anaesthesiol Scand. 1992; 36:369–377. PMID: 1595344.


25. Shin IW, Sohn JT, Choi JY, Lee HK, Lee CH, Chung YK. Cardiac arrest due to Severe hypokalemia during barbiturate coma therapy in a patient with severe acute head injury : a case report. Korean J Anesthesiol. 2006; 50:S71–S73.
26. Stephan H, Sonntag H, Schenk HD, Kohlhausen S. [Effect of Disoprivan (propofol) on the circulation and oxygen consumption of the brain and CO2 reactivity of brain vessels in the human]. Anaesthesist. 1987; 36:60–65. PMID: 3107419.
27. Van Hemelrijck J, Fitch W, Mattheussen M, Van Aken H, Plets C, Lauwers T. Effect of propofol on cerebral circulation and autoregulation in the baboon. Anesth Analg. 1990; 71:49–54. PMID: 2363528.


28. Vernooy K, Delhaas T, Cremer OL, Di Diego JM, Oliva A, Timmermans C, et al. Electrocardiographic changes predicting sudden death in propofol-related infusion syndrome. Heart Rhythm. 2006; 3:131–137. PMID: 16443524.


29. Zhou W, Fontenot HJ, Wang SN, Kennedy RH. Propofol-induced alterations in myocardial beta-adrenoceptor binding and responsiveness. Anesth Analg. 1999; 89:604–648. PMID: 10475288.


Fig. 1
Mean (standard deviation) serum potassium against time after induction of thiopental or propofol continuous infusion.
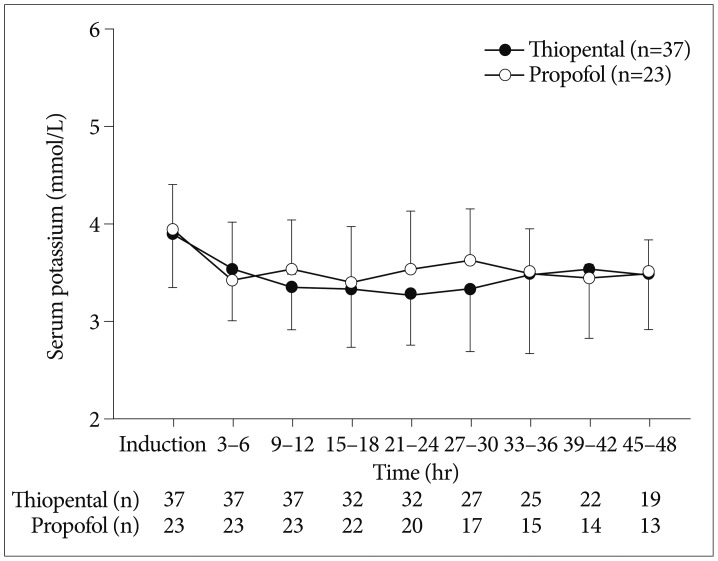
Fig. 2
Mean (standard deviation) serum potassium against time after cessation of thiopental or propofol continuous infusion.
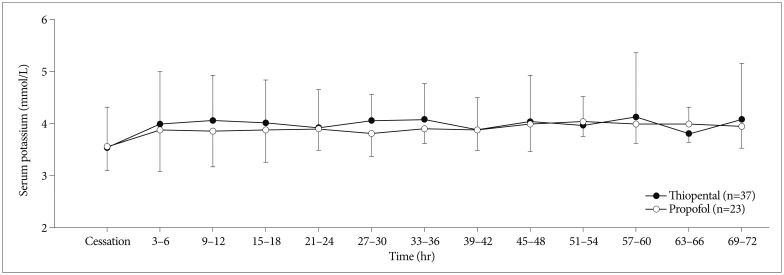
Table 3
Clinical outcomes of patients receiving thiopental or propofol continuous infusion for intracranial pressure regulation
