Abstract
Objective
With the growing interests of bacteria as a targeting vector for cancer treatment, diverse genetically engineered Salmonella has been reported to be capable of targeting primary or metastatic tumor regions after intravenous injection into mouse tumor models. The purpose of this study was to investigate the capability of the genetically engineered Salmonella typhimurium (S. typhimurium) to access the glioma xenograft, which was monitored in mouse brain tumor models using optical bioluminescence imaging technique.
Methods
U87 malignant glioma cells (U87-MG) stably transfected with firefly luciferase (Fluc) were implanted into BALB/cAnN nude mice by stereotactic injection into the striatum. After tumor formation, attenuated S. typhimurium expressing bacterial luciferase (Lux) was injected into the tail vein. Bioluminescence signals from transfected cells or bacteria were monitored using a cooled charge-coupled device camera to identify the tumor location or to trace the bacterial migration. Immunofluorescence staining was also performed in frozen sections of mouse glioma xenograft.
Of the myriad cancer types, malignant gliomas are probably the most devastating and difficult-to-treat cancer. Although enormous advances have been made in the past decade in treating other solid cancers, such as lung and breast cancer, the median survival for the glioblastoma, the most malignant type of gliomas, stayed nearly the same with average about 1 year2). Regardless of advances in surgical and imaging techniques, we still face numerous obstacles when treating brain tumors.
In brain tumors, the blood-brain barrier, the blood-cerebrospinal fluid barrier and the blood-tumor barrier may act as obstacles in drug delivery. One of the main reasons for the continuing dismal outlook in treatment of malignant gliomas is the lack of effective methods for drug delivery. Most conventional methods for delivering drug molecules, such as oral administration or intravenous injection, fail to achieve therapeutic concentrations of drugs within intracranial tumors, despite reaching toxic systemic levels.
Live bacteria were first used in the treatment of cancer about 150 years ago. Today, bacterial strains have been demonstrated specifically targeting in cancers, and supported by genomic sequencing and genetic engineering, there is new interest in the use of bacteria as a vector for cancer treatment7,8). A number of recent publications have revealed that bacteria are capable of targeting both primary cancers and metastases, enabling them to be imaged noninvasively in mouse tumor models3,4,12,13,14). Salmonella grow under both aerobic and anaerobic conditions, and so are able to colonize small and large tumors, respectively. Our review of prior publications revealed evidence that attenuated salmonella hinder tumor growth in a broad range of human and mouse tumors implanted in mice.
In the present study, we engineered an attenuated Salmonella typhimurium (S. typhimurium) defective in guanosine 5'-diphosphate-3'-diphosphate (ppGpp) synthesis (ΔppGpp strain), which expresses the bacterial luciferase gene (Lux) as a means of providing an imaging signal. The lux operon was integrated into the salmonella chromosome; therefore the attenuated bacteria produce bioluminescence which does not require an exogenous source of substrate. And then, we established mouse glioma xenograft models by stereotactic injection of glioma cell line, which could be visualized using bioluminescence to evaluate tumor growth, and investigated the targeting of the glioma xenograft by the genetically engineered S. typhimurium. The bioluminescence emitted from the salmonella was optically detected in the mouse glioma xenograft models.
U87 malignant glioma cells (U87-MG) cells were grown in high-glucose Dulbecco's modified Eagle's medium containing 10% fetal bovine serum and 1% penicillin-streptomycin. U87-MG cell line was transfected with firefly luciferase (Fluc), as shown in previous paper6).
S. typhimurium-ΔppGpp-Lux has been previously described6). Bacterial doses were 3×107 colony-forming units per mouse suspended in 100 µL of phosphate buffered saline and injected into the tail vein of each mouse.
The brains of sacrificed mice were removed, fixed in formalin, and embedded in paraffin. For immunofluorescent staining, the primary and secondary antibody was rabbit anti-salmonella (Abcam, Cambridge, MA, USA) and Alexa Fluor 488 chicken anti-rabbit (1:100; Invitrogen, Carlsbad, CA, USA), respectively. The samples were mounted with 4',6-diamidino-2-phenylindole/Antifade (1:200; Invitrogen, Carlsbad, CA, USA). Images of fluorescently immunolabeled sections were acquired using an 80i microscope (Nikon, Tokyo, Japan). Images were captured using Cell-P imaging software (Olympus, Tokyo, Japan) in both the fluorescein isothiocyanate and tetramethylrhodamine isothiocyanate channels.
All animal care and procedures were approved by Animal Care and Use Committees of our institute. U87-Fluc was implanted in 6-week old male BALB/cAnN athymic nu-/nu- mice (Orient Bio, Seoul, Korea) by stereotaxic injection into the left striatum. Mice were anesthetized with isoflurane (1.5% in air) and secured in a stereotaxic apparatus (Stoelting Europe, Dublin, Ireland). The skull was exposed by a midline scalp incision. A small hole (0.5 mm diameter) was drilled 3 mm posterior to and 3 mm left from the bregma. U87-Fluc cells (5×105) respectively dissolved in 5 µL of minimum essential medium were injected with a 10 µL Hamilton syringe into the left hemisphere at a depth of 3.5 mm below the brain surface. On retreat of the injection needle, the hole in the skull was sealed with bone wax and the scalp was sutured. Tumor growth was followed using the IVIS100-system (Caliper, Hopkinton, MA, USA) until the region of interest (ROI) reached around 2×107 (photons/s/cm2/sr) (about day 15 in U87-Fluc-bearing nude mouse), whereupon S. typhimurium-ΔppGp-pLux intravenously into the tail vein. RIO signal was analyzed at IVIS-system every day.
To obtain images of bacterial bioluminescence, anesthetized animals were placed in a light-tight chamber of the IVIS100 imaging system equipped with a cooled charge-coupled device (CCD) camera. The dose of luciferin (Caliper, Hopkinton, MA, USA) that was intraperitoneally (i.p.) injected was 3 mg per mouse. Photons emitted from luciferase-expressing bacteria were collected and integrated over 1-minute periods. Pseudo-color images indicating photon counts were overlaid on photographs of the mice using the Living Image software v. 2.25 (Caliper, Hopkinton, MA, USA). A ROI was selected manually based on the signal intensity measured at the same site. An integration time of 1 minute was used for luminescent image acquisition. The data of the ROI was normalized to peak signal intensity of each time course.
Serial bioluminescence images were collected from nude mouse for 30 min after i.p. injection of D-luciferin, and the mean photon flux relative to peak signal was determined. Fig. 1A shows the bioluminescence images of a nude mouse with orthotopic glioma xenograft from this experiment. Bioluminescence images of nude mouse, displayed as a pseudocolor image in the brain, revealed intense signals arising from the tumor. The peak activity was selected among the time-course images.
Bioluminescence images of U87-Fluc tumors developed in mice (n=8) from day 10 to day 24 after implantation were obtained (Fig. 1B). Modification of cells by Fluc tranfection allowed stable, long-term expression of the luciferase gene by the transduced cells. This optical bioluminescence of brain tumor model was non-invasive, reproducible, repeated detection using the cooled-CCD camera. We repeated this experiment using the mouse implanted with Lewis lung cancer-Fluc (LLC-Fluc) cell line (data not shown).
Expression of the Lux gene was monitored using a cooled CCD camera. We determined the spatial distribution of S. typhimurium-ΔppGpp carrying Fluc, using nude mice in which the U87-Fluc cell line had been implanted in the brain 15 days (Fig. 2A-a) before S. typhimurium-ΔppGpp (3×107 CFU) were intravenously injected into each mouse via the tail vein. Bioluminescence images were then collected. During the first few hours, bioluminescence signals were detected mainly in the spleen and liver of these mice. However, 5 days post-inoculum, signals were detected exclusively in the tumor region, and persisted more than 6 days. Strong bioluminescence signals from the chromosomal lux operon of salmonellae were detected specificially in the tumor area in 6 mice (Fig. 2A-b-d). No signal was detected superficially in two mice. However, these mice displayed signal after removing out brain for direct detecting under the a cooled CCD camera. Mouse was sacrificed to perform Hematoxylin & Eosin and immunofluorescence staining at day 9 after bacteria injection. The histological analysis showed a human glioma without necrosis (Fig. 2B). Immuofluorescence staining revealed that the genetically engineered bacteria maintained their ability to target glioma xenograft injected with the U87-Fluc cell line (Fig. 2C-a-d). No bacteria were detected in the opposite normal brain (Fig. 2C-e-h). We repeated this experiment in LLC-Fluc bearing-tumor model after tail vein injection with salmonella, the bacteria also targeted the brain tumor (data not shown).
GBM is the most devastating and difficult-to-treat cancer. The overall prognosis of brain cancer patients has remained virtually unchanged for the last 20 years11), with minimal improvement despite advances in surgery, chemotherapy, and radiation treatment. One of those reasons is the lack of effective methods of drug delivery. Therefore, it is necessary to develop novel drug delivery system. For this purpose, we considered the targeted use of bacteria as drug delivery vehicles.
Orthotopic brain tumors are inaccessible for direct measurements of tumor growth, therefore in vivo bioluminescence imaging is particularly attractive in evaluating brain tumor status. One study concluded that bioluminescence imaging is a highly sensitive technique for assessment of tumor burden and response to therapy, making it a superior option for longitudinal monitoring in intracranial rodent models of primary brain tumors1). Two studies compared bioluminescence signal of intracranial supratentorial tumors with magnetic resonance imaging-based measurements using complementary models-the 9L gliosarcoma model in rat9) and the U87 model in nude mice10)- and found an excellent correlation. So, bioluminescence imaging is a useful tool to evaluate the brain tumor generation and growth. But, the bioluminescence signal of the tumor is suddenly lost during penetration through tissue and skull, making comparisons between mice is difficult because of the variable depth of targeting in each tumor. Using each mouse as its own control normalizes these variations that make data more believable. Therefore, we established a glioma xenograft model that could be traced using a cooled CCD camera. Attenuated S. typhimurium reportedly has a propensity to naturally accumulate and replicate in a wide variety of solid tumors7). We previously reported that genetically engineered S. typhimurium target subcutaneous tumors5). Although there are some doubt in safety of bacterial injection, previous investigations demonstrated that salmonella injection with the amount used in this study (3×107 CFU) did not decline body weight and survival time13,14).
There has been a investigation about therapeutic potential of salmonella for intracranial tumor5). However, this paper did not demonstrate direct evidence of bacterial targeting in brain. They checked GFP-labeled bacteria under the CCD camera without immunofluorescence or IHC staining. Furthermore, without treatment effect after tail vein injection, inhibition of tumor growth was only observed after directly intracranial injection of bacteria. The main reason of these results could be explained by lower sensitivity of GFP to be detected under CCD camera.
Our paper focuses on bacteria targeting in glioma tumor model using more sensitive reporter gene Lux. In this report, we have demonstrated that S. typhimurium-ΔppGpp-Lux targeted glioma xenograft in a mouse model after tail vein injection. In sharp contrast, normal tissue rapidly cleared these bacteria even in immunodeficient athymic mice. Three days later, S. typhimurium was undetectable in the liver, lung, spleen, and kidney, but it continued to proliferate in the brain tumor for over 6 days. Therefore, genetically engineered S. typhimurium may be a useful probe for targeting therapy glioma in the future.
Acknowledgements
This research was supported by Leading Foreign Research Institute Recruitment Program through the National Research Foundation of Korea (NRF) funded by the Ministry of Education, Science and Technology (MEST) (2011-0030034).
References
1. Dinca EB, Voicu RV, Ciurea AV. Bioluminescence imaging of invasive intracranial xenografts : implications for translational research and targeted therapeutics of brain tumors. Neurosurg Rev. 2010; 33:385–394. PMID: 20652720.


2. Hoffman S, Propp JM, McCarthy BJ. Temporal trends in incidence of primary brain tumors in the United States, 1985-1999. Neuro Oncol. 2006; 8:27–37. PMID: 16443945.


3. Min JJ, Kim HJ, Park JH, Moon S, Jeong JH, Hong YJ, et al. Noninvasive real-time imaging of tumors and metastases using tumor-targeting light-emitting Escherichia coli. Mol Imaging Biol. 2008; 10:54–61. PMID: 17994265.


4. Min JJ, Nguyen VH, Kim HJ, Hong Y, Choy HE. Quantitative bioluminescence imaging of tumor-targeting bacteria in living animals. Nat Protoc. 2008; 3:629–636. PMID: 18388945.


5. Momiyama M, Zhao M, Kimura H, Tran B, Chishima T, Bouvet M, et al. Inhibition and eradication of human glioma with tumor-targeting Salmonella typhimurium in an orthotopic nude-mouse model. Cell Cycle. 2012; 11:628–632. PMID: 22274398.


6. Nguyen VH, Kim HS, Ha JM, Hong Y, Choy HE, Min JJ. Genetically engineered Salmonella typhimurium as an imageable therapeutic probe for cancer. Cancer Res. 2010; 70:18–23. PMID: 20028866.


7. Pawelek JM, Low KB, Bermudes D. Bacteria as tumour-targeting vectors. Lancet Oncol. 2003; 4:548–556. PMID: 12965276.


8. Pawelek JM, Low KB, Bermudes D. Tumor-targeted Salmonella as a novel anticancer vector. Cancer Res. 1997; 57:4537–4544. PMID: 9377566.
9. Rehemtulla A, Stegman LD, Cardozo SJ, Gupta S, Hall DE, Contag CH, et al. Rapid and quantitative assessment of cancer treatment response using in vivo bioluminescence imaging. Neoplasia. 2000; 2:491–495. PMID: 11228541.


10. Szentirmai O, Baker CH, Lin N, Szucs S, Takahashi M, Kiryu S, et al. Noninvasive bioluminescence imaging of luciferase expressing intracranial U87 xenografts : correlation with magnetic resonance imaging determined tumor volume and longitudinal use in assessing tumor growth and antiangiogenic treatment effect. Neurosurgery. 2006; 58:365–372. discussion 365-372. PMID: 16462491.


11. Tang Y, Shah K, Messerli SM, Snyder E, Breakefield X, Weissleder R. In vivo tracking of neural progenitor cell migration to glioblastomas. Hum Gene Ther. 2003; 14:1247–1254. PMID: 12952596.
12. Yu YA, Shabahang S, Timiryasova TM, Zhang Q, Beltz R, Gentschev I, et al. Visualization of tumors and metastases in live animals with bacteria and vaccinia virus encoding light-emitting proteins. Nat Biotechnol. 2004; 22:313–320. PMID: 14990953.


13. Zhao M, Geller J, Ma H, Yang M, Penman S, Hoffman RM. Monotherapy with a tumor-targeting mutant of Salmonella typhimurium cures orthotopic metastatic mouse models of human prostate cancer. Proc Natl Acad Sci U S A. 2007; 104:10170–10174. PMID: 17548809.


14. Zhao M, Yang M, Li XM, Jiang P, Baranov E, Li S, et al. Tumor-targeting bacterial therapy with amino acid auxotrophs of GFP-expressing Salmonella typhimurium. Proc Natl Acad Sci U S A. 2005; 102:755–760. PMID: 15644448.


Fig. 1
Visualization of tumor development on optical bioluminescence imaging. To evaluate tumor growth in U87-Fluc-bearing nude mouse, a cooled CCD camera was used to analyze the mouse brain tumor model after intraperitoneal injection of 3 mg of D-luciferin per animal. A : The signal gradually increased in brain up to a peak at approximately 30 min, after which time, the signal slowly decayed. B : Bioluminescence images of U87-Fluc tumor developed in mouse over POD 10 and gradually increased over the time. POD : post-operative day, CCD : charge-coupled device.
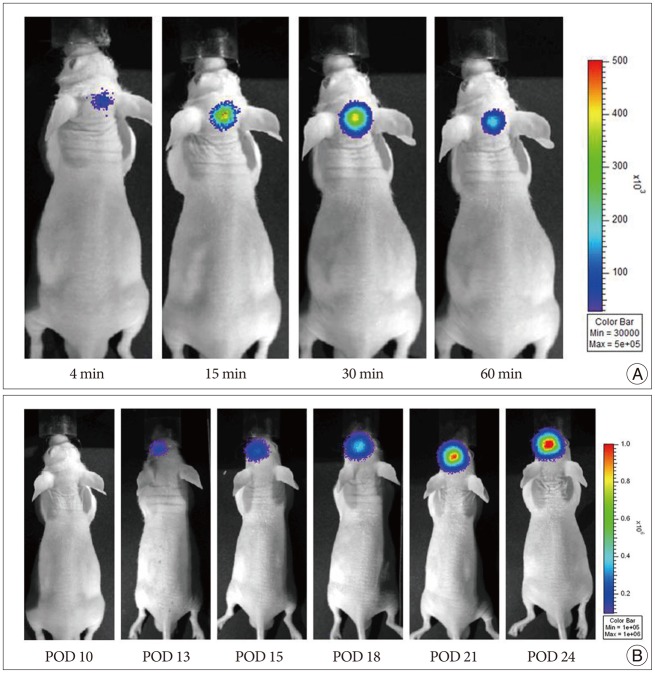
Fig. 2
Bioluminescence imaging of S. typhimurium-ΔppGpp-Lux targeting in the U87-Fluc mouse glioma model. A : In vivo bioluminescence imaging was performed on a nude mouse with U87-Fluc stably expressing firefly luciferase in the left stiatum. (a) After 15 days of celluluar inoculation, tumor growth was demonstrated by intraperitonealy injection of D-lucferin. (b, c, and d) And then the S. typhimurium-ΔppGpp-Lux (3×107 CFU) was administered intravenously via the tail vein in POD 15, and exclusively proliferated in the tumor region at 5 dpi (POD 20). Animals were killed 9 days post-beacteral injection and brain tissue was examined histologically using Hematoxylin & Eosin (B) and immunofluorescence (C) staining. Hematoxylin & Eosin staining shows brain tumor and opposite normal brain. Immunofluorescence staining demonstated S. typhimurium in the corresponding area (small black box in B) of tumor growth, not in normal brain (small white box in B) (a and e : H&E, b and f : DAPI, c and g : anti-salmonella, d and h : merged ×200). POD : post-operative day, dpi : days after post-inoculation.
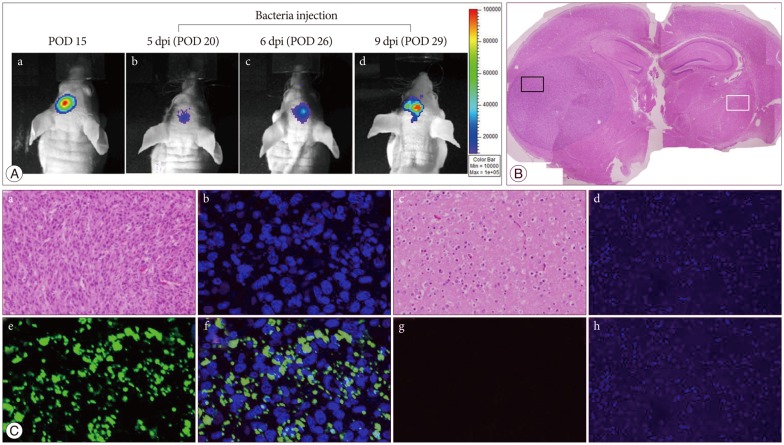