Abstract
Objective
Recently, microscope-integrated near infrared indocyanine green videoangiography (ICG-VA) has been widely used in cerebrovascular surgery because it provides real-time high resolution images. In our study, we evaluate the efficacy of intraoperative ICG-VA during cerebrovascular surgery.
Methods
Between August 2011 and April 2012, 188 patients with cerebrovascular disease were surgically treated in our institution. We used ICG-VA in that operations with half of recommended dose (0.2 to 0.3 mg/kg). Postoperative digital subtraction angiography and computed tomography angiography was used to confirm anatomical results.
Results
Intraoperative ICG-VA demonstrated fully occluded aneurysm sack, no neck remnant, and without vessel compromise in 119 cases (93.7%) of 127 aneurysms. Eight clipping (6.3%) of 127 operations were identified as an incomplete aneurysm occlusion or compromising vessel after ICG-VA. In 41 (97.6%) of 42 patients after carotid endarterectomy, the results were the same as that of postoperative angiography with good patency. One case (5.9%) of 17 bypass surgeries was identified as a nonfunctioning anastomosis after ICG-VA, which could be revised successfully. In the two patients of arteriovenous malformation, ICG-VA was useful for find the superficial nature of the feeding arteries and draining veins.
Conclusion
ICG-VA is simple and provides real-time information of the patency of vessels including very small perforators within the field of the microscope and has a lower rate of adverse reactions. However, ICG-VA is not a perfect method, and so a combination of monitoring tools assures the quality of cerebrovascular surgery.
Several methods have been used in cerebrovascular surgery to identify aneurysm obliteration or graft patency or residual nidus. The standard technique to assess anatomic results and confirm success or failure of cerebrovascular surgery is postoperative angiography. However, in case of abnormal finding in postoperative angiography, irreversible neurological deficit due to ischemic injury may have been developed already, or another surgical procedure could be required.
Numerous authors have recommended the application of intraoperative digital subtraction angiography (DSA) to readjust an imperfect procedure immediately5,13). However, the routine use of intraoperative DSA is not available at most institutions. Therefore, there is a need for a simple and reliable method with high resolution that allows intraoperative observation and documentation of blood flow in large and small vessels.
Indocyanine green (ICG) fluorescence angiography is widely used in ophthalmology for the assessment of the retinal microcirculation in a variety of disorders. Recently, many surgeons have advocated the use of microscope-integrated near infrared ICG videoangiogrphy for intraoperative assessment3,4,16). We report our early experience with intraoperative ICG videoangiography during the surgical treatment of cerebrovascular disease.
Between August 2011 and April 2012, 188 patients with cerebrovascular disease were surgically treated by the authors in our institution. The patient population consisted of 84 males and 104 females. The patients' age ranged from 28 to 82 years (mean 59.3 years). Fifty-eight surgeries followed an acute subarachnoid hemorrhage (SAH), 69 operations were performed on patients with unruptured aneurysms, 42 cases of carotid endarterectomy (CEA) were performed, 17 extracranial to intracranial (EC-IC) bypass surgeries were performed on patients with Moyamoya or atherosclerotic occlusion or complex aneurysm, and two surgeries involved arteriovenous malformation (AVM). According to the aneurysm location, there were 55 anterior cerebral artery (ACA) aneurysms, 41 middle cerebral artery (MCA) aneurysms, 18 aneurysms of the internal cerebral artery (ICA), one aneurysm at the vertebrobasilar (VB) artery, and 12 multiple aneurysms. For aneurysm clipping, a pterional transsylvian, bifrontal interhemispheric, suboccipital approach was performed depending on site and size of the aneurysm. CEA was performed for symptomatic patients with more than 50% carotid stenosis and for asymptomatic patients with more than 60% carotid stenosis by angiography. Indications for EC-IC bypass surgery were hemodynamic compromise due to atherosclerotic stenooclusive cerebrovascular disease in seven patients, Moyamoya syndrome or disease in six patients, and the presence of complex intracranial aneurysms in four patients; the four patients were treated with aneurysm trapping (n=3) and aneurysmorrhaphy (n=1). Patients underwent direct revascularization either through a standard superficial temporal artery (STA)-MCA bypass (n=14), an occipital artery (OA)-posterior inferior cerebellar artery (PICA) bypass (n=2), or radial artery (RA) high-flow bypass (n=1). Both of the patients with AVM were Spetzler-Martin grade 1 and were scheduled for elective surgery.
Intraoperative ICG videoangiography (ICG-VA) was done using an OPMI® Pentero™ surgical microscope (Carl Zeiss, Jena, Germany). The recommended dose of ICG dye for VA is 0.2 to 0.5 mg/kg, and the maximum daily dose should not exceed 5 mg/kg. For measurements, the unit was positioned 30 to 40 cm from the area of interest. Care was taken to uniformly illuminate the area of interest with the laser light source. At the time of angiography, ICG was then administered intravenously by the anesthesiologist upon the request of the surgeon. Half dose (0.2 to 0.3 mg/kg) was used as a bolus (standard dose of 25 mg dissolved in 5 mL water).
Anatomical results assessed by postoperative angiography included the remnant of aneurysm after clipping, patency of graft or recanalized internal carotid artery, and remnant of nidus. Postoperative DSA and computed tomography (CT) angiography was used to confirm anatomical results on aneurysm patient at 14 days postoperatively. An almost unruptured aneurysm and SAH patients whose condition was not satisfactory to perform DSA were examined by CT angiography. Also, we performed CT angiography on the patients 7 days after CEA. All patients received bypass surgery or surgical removal of AVM were confirmed by DSA 7 days postoperatively. The results of intraoperative ICG-VA were compared with postoperative angiography.
During the 188 cerebrovascular surgeries, 198 ICG videoangiographic examinations were performed. Table 1 shows the classification of patients after ICG-VA by diagnosis and performed procedure. All patients tolerated the intravenous injection of ICG well. Side effects or allergic reactions were not observed. If necessary, the videoangiographic study could be repeated within 15 minutes following clearance of the ICG. In these cases, repeated injections did not pose any problems with respect to patient safety and image quality. The image quality and resolution allowed assessment of arterial branches, including small arteries (<0.5 mm in diameter). The ICG-VA proved to be an easy-to-use and practical procedure, adding no more than 3 minutes to the regular operation time.
Intraoperative ICG-VA demonstrated fully occluded aneurysm sac without vessel compromise in 119 cases (93.7%) of 127 aneurysms. However, a small portion of the clipped aneurysm was filled with bright fluorescent material in 6 patients (4.7% : five unruptured cases and one SAH case), and small perforators or branched arteries were not showed in two cases (1.6%). Correction of the clip was done immediately by adding another clip on the previously clipped aneurysm in 4 patients and repositioning the clip in 4 patients. Right after clip correction, we performed ICG-VA and the repeated VA demonstrated good reduction (Table 3).
Postoperative DSA was performed on 33 of 58 patients of SAH and 4 of 69 unruptured aneurysms. CT angiography was performed postoperatively on 90 patients instead of DSA. Postoperative angiographies showed 126 aneurysms (99.2%) without residual aneurysm sac and vessel compromise, which are the same results of intraoperative ICG-VA. Intraoperative findings of ICG-VA in relation to postoperative DSA are listed in Table 2.
However, in one case of a ruptured left MCA bifurcation aneurysm, postoperative CT angiography showed a 6 mm size remnant sac, although intraoperative ICG-VA did not show any residual site of the aneurysm. One month later, the patient had been scheduled for a cranioplasty due to a skull defect caused by severe brain swelling. During the operative procedure, we became aware that we could not identify the residual site mimicking the branched artery because of severe atherosclerosis. The remnant was clipped completely.
Forty-two intraoperative ICG-VA were performed after endarterectomy. In all cases, the ICA was demonstrated to be patent on ICG-VA by homogenous enhancement following excision of the plaque. At the distal end, ICA patency was seen with no stenosis in all cases. Postoperative CT angiography performed in 41 patients (97.6%) at 7 days postoperatively showed good patency of ICA without any stenosis. In one patient, postoperative CT angiography showed a segmental stenosis (NASCET, <50%) at the distal part of the endarterectomy site. We planned to follow the patient and to consider stent insertion if symptoms were appeared.
Intraoperative ICG-VA revealed adequate bypass patency in 14 (100%) of 14 STA-MCA bypasses, in one (50%) of two OA-PICA bypasses, and in one (100%) high-flow bypass. In contrast, one patient undergoing OA-PICA bypass surgery was found intraoperatively to have an occlusion of the bypass graft. In this case, the cause of failure was intimal injury of the donor vessel at the anastomotic site. Following revision, revised bypass grafts finally exhibited good filling according to repeated ICG-VA. Postoperative DSA was performed in all 17 patients who received bypass surgery. A comparison of the intraoperative findings of ICG-VA with the findings of postoperative DSA confirmed adequate bypass graft patency in 16 patients, which resulted in an early bypass patency rate of 94.1%. However, in one patient with complex supraclinoid ICA aneurysm who were treated with RA-high flow bypass and aneurysm trapping, postoperative DSA showed an occlusion of RA graft though intraoperative ICG-VA have shown good patency. Fortunately, the DSA demonstrated good collateral flow from posterior communicating artery, and the patient presented no neurological deficit although mild dysarthria was seen temporarily. Table 4 presents the characteristics of patients who received EC-IC bypass.
Four intraoperative ICG videoangiogrphy were performed in 2 patients. Both patients had an injection before and after resection of their AVM. In both, ICG-VA before resection revealed arteriovenous shunting and helped the surgeon to discern the AVM arteries, draining veins, and cortical veins. After resection of AVM, ICG-VA showed no remnant of nidus. Like intraoperative ICG-VA, postoperative DSA was negative for residual lesion.
Here, we describe several cases that showed aneurysmal remnant through ICG-VA after clipping. First case was a 62-year-old female presented with dizziness. CT angiography indicated a distal anterior cerebral artery aneurysm, the diameter was 8 mm. We performed bifrontal craniotomy and clipping of the aneurysm through interhemispheric approach (Fig. 1A, B). Second was a 42-year-old woman showed convulsive seizure, the 7 mm sized aneurysm at MCA bifurcation was detected incidentally on CT angiography. Patient underwent aneurysmal clipping through traditional pterional approach (Fig. 1C, D). The third case was a 61-year-old woman who presented with headache. We performed clipping of the 5 mm sized aneurysm at anterior communicating artery disclosed by cerebral angiogram (Fig. 1E, F).
In six cases including above three cases, after the clipping of the aneurysm, ICG-VA showed small neck remnant. All aneurysmal filling sites with fluorescent material were at the end of the clip blade. The filling pattern was typical. The brightness of fluorescence presented with two patterns; it has become brighter gradually, or it has showed "blinking sign" caused by arterial pulsation.
For a long time, ICG dye has been used in ophthalmology to measure retinal blood flow with a clinically relevant complication rate of less than 0.1% (hypotension, tachycardia, or an allergic reaction to iodine)7). Feindel et al.8,9) were the first to apply the concept of fluorescence angiography to the intraoperative visualization of cerebral microcirculation in patients undergoing neurosurgical procedure.
ICG-VA has proven to be a practical and low-cost technique that can be easily applied in the neurosurgical operating room on a routine basis. One of the advantages of ICG dye is that it can be administered intravenously as a bolus injection. Therefore, the procedure can be performed any time during surgery, and repeated administration is possible if a minimum time interval of 15 minutes between repeated injections to allow clearance is maintained. ICG-VA is very simple and rapid, and there is no need to move the microscope from the surgical field or to interrupt the operation. In our study, the results of ICG-VA were available within 3 minutes for all patients. This simplicity and speed are very important advantages of ICG-VA for the neurosurgeon to act immediately to correct the mistake before critical cerebral injury can occur.
In our experiences, ICG-VA is the most useful option in aneurysm surgery. Its high resolution allows the neurosurgeon to identify the patency of all vessels within the surgical field. Especially, ICG-VA is the most accurate tool to confirm complete aneurysmal occlusion and patency of very small perforating arteries. We need not puncture the clipped aneurysm sac to ensure no bleeding or to relieve concern about whether the very small perforator is patent or not. The rate of postoperative aneurysm remnants ranges from 4% to 19%1,6,14) and unexpected vessel occlusion ranges from 0.3% to 12% in aneurysm surgery. Although postoperative detection of unexpected findings might necessitate reoperation, the results of such revision are not very good14). Similar to previous reports, in our study, eight clipping (6.3%) of 127 operations were identified as an incomplete aneurysm occlusion or compromising vessel after ICG-VA. However, we could correct the clipping immediately and we did not need to reoperate except for one remnant. In a review of the literature, after ICG-VA in aneurysm surgery, the incidence of residual filling of aneurysm is 2% to 8%, and that of parent or branching artery occlusion is 4% to 12%10,17,22). Raabe et al.17) reported that clip adjustment was performed in 10 of 111 cases after clip application. Wang et al.22) reported that clip reperforming was carried out in three of 101 aneurysm cases. The rates of concordance between intraoperative ICG-VA and postoperative DSA in previous reports as well as our study are 90% to 100%10,17,22). However, the significant limitation of the ICG angiographic view is the restriction to the angle of surgical approach for vessels covered by blood clots, aneurysm, or brain tissue that are not visible to the surgeon. In other words, ICG-VA captures only what is visible within the field of the microscope. In our case, the remnant aneurysm occurred because we ignored this fact and assumed the success of the ICG-VA, although marginal dissection was insufficient. Moreover, repeated injections of ICG for more information within a short interval may cause false positive findings. Proximal-to-distal filling of arteries should be undertaken carefully to avoid misdiagnosing the retrograde filling of the branches distal to the aneurysm.
ICG-VA was effective in identifying ICA patency after CEA according to our study. In 41 (97.6%) of 42 patients, the results of intraoperative ICG-VA were the same as that of postoperative DSA with good patency except for one patient. However, ICG-VA was not sufficient to find the exact bounds of atherosclerosis before arteriotomy, although the fact that there was only one case in our study makes this conclusion tentative. Haga et al.11) reported that ICG-VA was performed in all of 60 CEA procedures and good patency could be identified. Also, they reported that ICG-VA showed the exact location of stenosis before arteriotomy in some cases, although it was impossible in many more cases because of spontaneous fluorescence of calcified tissue. Although there are a few reports on the application of ICG-VA during CEA2), in our opinion, ICG-VA is useful for intraoperative evaluation of the patency after CEA because it is easy to handle and rapidly provides high-quality information on the blood stream of the cervical ICA. In our one case, which ICG-VA was performed before and after arteriotomy, we could not find any advantage for finding the exact location of stenosis compared to inspection or palpation. Because visualization of ICG fluorescence may also be affected by the thickness and calcification, ICG-VA can be used selectively to predict the range of arteriotomy in CEA.
In bypass surgeries, ICG-VA is thought to be very useful. Even if there was one case of early graft failure, we thought that there had been spasm at the RA graft and the graft developed occlusion gradually after ICG-VA. In our study, excellent visualization of cererbral arteries, the bypass graft, and brain perfusion was noted in all cases. One case of 17 bypass surgeries was identified as a nonfunctioning OA-PICA bypass, which could be revised successfully. The postoperative bypass patency rate has been reported to be only between 90% and 96%7,15,18). There have been no possible methods that provide direct intraoperative inspection about the blood stream except intraoperative DSA. However, Woitzik et al.23) reported the value of ICG-VA for the revision of four of 35 STA-MCA bypasses. In addition, all four cases exhibited good filling of the bypass according to repeated ICG-VA. Several subsequent studies19,21) have reported that ICG-VA has the potential to improve the outcomes of bypass surgery. However, it is hard to determine the direction of blood flow with ICG-VA, so we recommend additional Doppler sonography to confirm the direction.
Previous reports about ICG-VA using AVM are infrequent. In our two cases, ICG-VA before resection showed arteriovenous shunting and helped the surgeon to discern the AVM arteries, draining veins, and cortical veins. However, both were only Spetzler-Martin (S-M) grade I AVMs. The finding that ICG-VA was useful for AVM removal could reflect the superficial nature of the feeding arteries and draining veins in our cases. Killory et al.12) reported that, although application of ICG-VA in 10 AVMs was ideal for early identification of AVM arteries and veins, ICG-VA only complemented rather than replaced intraoperative DSA. This because, in their experience, ICG-VA is less useful with deep-seated lesions or when AVM vessels are not on the surface, such as S-M grade III or IV. Killory et al. reported that ICG is frequently of limited use for deep-seated AVMs that must be approached through a long, narrow corridor. However, our study had same result as study by Takagi et al.,20) that ICG-VA is useful utility to determine the remnant of nidus postoperatively.
It is well-known that ICG-VA can show feeder, draining vein, cortical vein, and nidus in AVM surgery. ICG-VA clearly revealed arteriovenous shunting and helped the surgeon to discern the AVM arteries, draining veins, and cortical veins in sequence from arterial phase to venous phase, distinctions that were not obvious with conventional intraoperative microscopic magnification.
After review of the previous literature, we found that there was no consideration about the filling pattern of ICG dye in incomplete clipped aneurysms. In our six incomplete clipped aneurysm cases, the ICG filling showed typical patterns. All aneurysmal filling sites with fluorescent material were the tip site of the clip blade. There was no case that ICG filling was showed at the heel site of clip blade and we believe that is result of clip blade pressure force. Furthermore, the brightness of fluorescence presented with two patterns : it has become brighter gradually, or it has showed "blinking sign". This can be shown at incompletely clipped aneurysm with extremely minimal gap for contrast to flow, but can be seen or blink on arterial pulsation. This pattern is important to discern the residual ICG filling of aneurysm from diffuse weak fluorescence of thrombus or atherosclerosis or calcification.
It is very important to identify small perforator which is main factor of postoperative neurological deficit in cerebrovascular surgery. ICG-VA is the best way to identify small perforator compare to other methods such as intraoperative Doppler. Most ideal method is comparison of patency and brightness of small perforators before and after procedure via ICG-VA. However, most neurovascular surgeons can raise questions if unconfirmed perforators are less bright compare to adjacent arteries after procedure. In one of our cases with giant aneurysm at MCA, after STA-M3 bypass surgery with aneurysmorrhaphy, ICG-VA showed a trace of fluorescence at a small perforator. We repeated open and closure of the aneurysm three or four times for identification of backflow of the perforator. The backflow was very weak and bleed slowly from the orifice. However, the patency was retained according to postoperative DSA, and the patient had no neurological deficit, despite our concern (Fig. 2). Although it is difficult to establish the clear standard degree of the brightness of fluorescence, if the perforator is not complete dark with flow-free during ICG-VA and the procedure did not affect the perforator, it means that the flow have been weak even before procedure in our experience. Also, brightness of contrast in perforator does not imply the patency, so the monitoring of motor or sensory evoked potentials can be helpful to determine the patency of perforator.
ICG-VA is simple and provides real-time information of the patency of vessels including very small perforators within the field of the microscope and has a lower rate of adverse reactions. ICG-VA has the potential to achieve the goal of routine intraoperative vascular imaging during cerebrovascular surgery. However, ICG-VA is not a perfect method, and so a combination of monitoring tools assures the quality of cerebrovascular surgery.
Acknowledgements
This study was supported by a grant (CRI12009-1) Chonnam National University Hospital research institute of clinical medicine.
References
1. Alexander TD, Macdonald RL, Weir B, Kowalczuk A. Intraoperative angiography in cerebral aneurysm surgery : a prospective study of 100 craniotomies. Neurosurgery. 1996; 39:10–17. discussion 17-18. PMID: 8805135.
2. Chiang VL, Gailloud P, Murphy KJ, Rigamonti D, Tamargo RJ. Routine intraoperative angiography during aneurysm surgery. J Neurosurg. 2002; 96:988–992. PMID: 12066932.


3. Dashti R, Laakso A, Niemelä M, Porras M, Hernesniemi J. Microscope-integrated near-infrared indocyanine green videoangiography during surgery of intracranial aneurysms : the Helsinki experience. Surg Neurol. 2009; 71:543–550. discussion 550. PMID: 19328531.


4. de Oliveira JG, Beck J, Seifert V, Teixeira MJ, Raabe A. Assessment of flow in perforating arteries during intracranial aneurysm surgery using intraoperative near-infrared indocyanine green videoangiography. Neurosurgery. 2007; 61(3 Suppl):63–72. discussion 72-73. PMID: 17876226.


5. Dehdashti AR, Thines L, Da Costa LB, terBrugge KG, Willinsky RA, Wallace MC, et al. Intraoperative biplanar rotational angiography during neurovascular surgery. Technical note. J Neurosurg. 2009; 111:188–192. PMID: 19301971.


6. Drake CG, Allcock JM. Postoperative angiography and the "slipped" clip. J Neurosurg. 1973; 39:683–689. PMID: 4759655.


7. The EC/IC Bypass Study Group. Failure of extracranial-intracranial arterial bypass to reduce the risk of ischemic stroke. Results of an international randomized trial. N Engl J Med. 1985; 313:1191–1200. PMID: 2865674.
8. Feindel W, Yamamoto YL, Hodge CP. Intracarotid fluorescein angiography : a new method for examination of the epicerebral circulation in man. Can Med Assoc J. 1967; 96:1–7. PMID: 4959610.
9. Feindel W, Yamamoto YL, Hodge P. The human cerebral microcirculation studied by intra-arterial radio-active tracers, Coomassie Blue and fluorescein dyes. Bibl Anat. 1967; 9:220–224. PMID: 4961774.
10. Fischer G, Stadie A, Oertel JM. Near-infrared indocyanine green videoangiography versus microvascular Doppler sonography in aneurysm surgery. Acta Neurochir (Wien). 2010; 152:1519–1525. PMID: 20623360.


11. Haga S, Nagata S, Uka A, Akagi Y, Hamada Y, Shono T. Near-infrared indocyanine green videoangiography for assessment of carotid endarterectomy. Acta Neurochir (Wien). 2011; 153:1641–1644. discussion 1644. PMID: 21499960.


12. Killory BD, Nakaji P, Gonzales LF, Ponce FA, Wait SD, Spetzler RF. Prospective evaluation of surgical microscope-integrated intraoperative near-infrared indocyanine green angiography during cerebral arteriovenous malformation surgery. Neurosurgery. 2009; 65:456–462. discussion 462. PMID: 19687689.


13. Klopfenstein JD, Spetzler RF, Kim LJ, Feiz-Erfan I, Han PP, Zabramski JM, et al. Comparison of routine and selective use of intraoperative angiography during aneurysm surgery : a prospective assessment. J Neurosurg. 2004; 100:230–235. PMID: 15086229.


14. Macdonald RL, Wallace MC, Kestle JR. Role of angiography following aneurysm surgery. J Neurosurg. 1993; 79:826–832. PMID: 8246049.


15. Mendelowitsch A, Taussky P, Rem JA, Gratzl O. Clinical outcome of standard extracranial-intracranial bypass surgery in patients with symptomatic atherosclerotic occlusion of the internal carotid artery. Acta Neurochir (Wien). 2004; 146:95–101. PMID: 14963741.


16. Raabe A, Beck J, Seifert V. Technique and image quality of intraoperative indocyanine green angiography during aneurysm surgery using surgical microscope integrated near-infrared video technology. Zentralbl Neurochir. 2005; 66:1–6. discussion 7-8. PMID: 15744621.


17. Raabe A, Nakaji P, Beck J, Kim LJ, Hsu FP, Kamerman JD, et al. Prospective evaluation of surgical microscope-integrated intraoperative near-infrared indocyanine green videoangiography during aneurysm surgery. J Neurosurg. 2005; 103:982–989. PMID: 16381184.


18. Schmiedek P, Piepgras A, Leinsinger G, Kirsch CM, Einhüpl K. Improvement of cerebrovascular reserve capacity by EC-IC arterial bypass surgery in patients with ICA occlusion and hemodynamic cerebral ischemia. J Neurosurg. 1994; 81:236–244. PMID: 8027807.


19. Schuette AJ, Dannenbaum MJ, Cawley CM, Barrow DL. Indocyanine green videoangiography for confirmation of bypass graft patency. J Korean Neurosurg Soc. 2011; 50:23–29. PMID: 21892400.


20. Takagi Y, Sawamura K, Hashimoto N, Miyamoto S. Evaluation of serial intraoperative surgical microscope-integrated intraoperative near-infrared indocyanine green videoangiography in patients with cerebral arteriovenous malformations. Neurosurgery. 2012; 70(1 Suppl Operative):34–42. discussion 42-43. PMID: 21768916.


21. Wang JT, Zhang D, Zuo F, Wang S. [Application of indocyanine green angiography in bypass surgery for moyamoya disease]. Zhonghua Yi Xue Za Zhi. 2010; 90:1628–1630. PMID: 20979753.
22. Wang S, Liu L, Zhao YL, Zhang D, Yang MQ, Zhao JZ. [Application of indocyanine green angiography in bypass surgery for moyamoya disease]. Zhonghua Yi Xue Za Zhi. 2009; 89:146–150. PMID: 19537026.
23. Woitzik J, Horn P, Vajkoczy P, Schmiedek P. Intraoperative control of extracranial-intracranial bypass patency by near-infrared indocyanine green videoangiography. J Neurosurg. 2005; 102:692–698. PMID: 15871512.


Fig. 1
Surgical view (A, C and E) and ICG videoangiography (B, D and F) at the time of operation. All aneurysmal filling sites with fluorescent material were at the end of the clip blade. The filling pattern was typical. The brightness of fluorescence presented with two patterns; it has become brighter gradually, or it has showed "blinking sign" caused by arterial pulsation. ICG : indocyanine green.
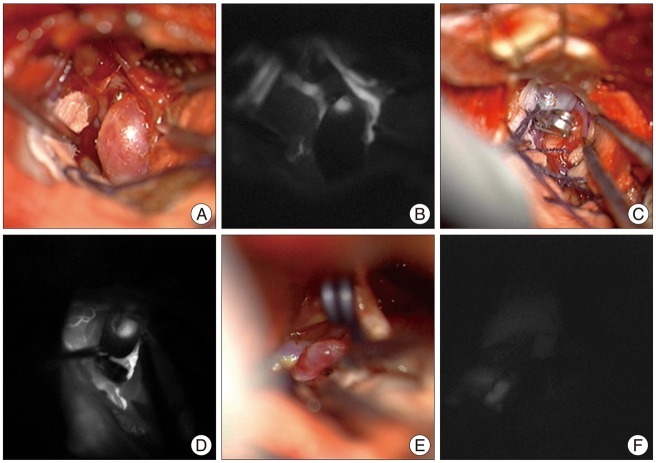
Fig. 2
Giant aneurysm at MCA (A). After STA-M3 bypass surgery with aneurysmorrhaphy, we concerned about the weak brightness at a small perforator (black and white arrow) on ICG-VA (B and C). We repeated open and closure of the aneurysm three or four times for identification of backflow of the perforator. After operation, the patency of the perforator was retained according to postoperative DSA (D, black arrow head), and the patient had no neurological deficit, despite our concern. MCA : middle cerebral artery, STA : superficial temporal artery, ICG-VA : indocyanine green videoangiography, DSA : digital subtraction angiography.
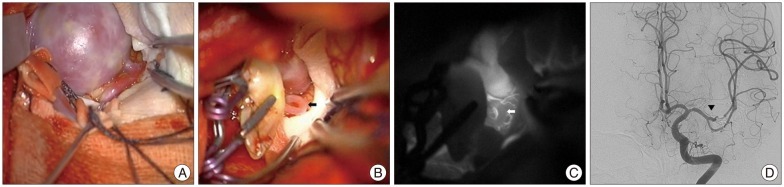
Table 1
Classification of patients after indocyanine green videoangiography by performed procedure and diagnosis

Table 2
The results of aneurysm clipping after indocyanine green videoangiography and relation to postoperative angiography

Table 3
Characteristics of cerebral aneurysm patients who underwent additional procedure after indocyanine green videoangiography
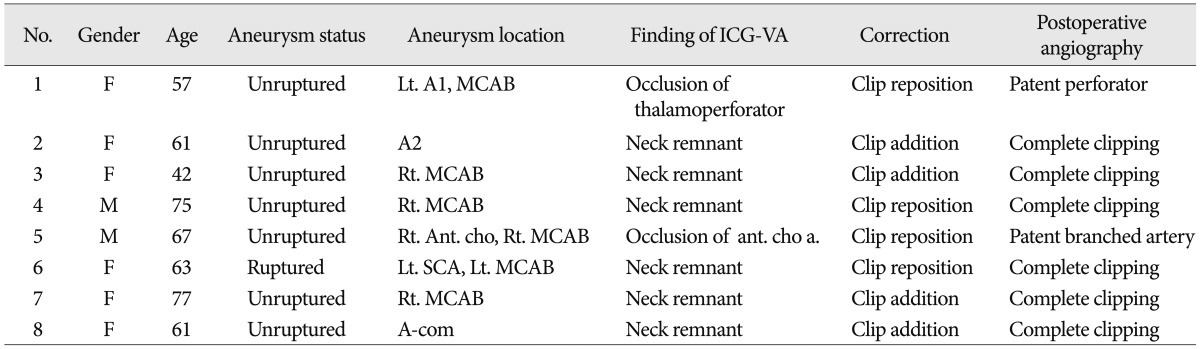
Table 4
Summary of demographic and treatment-related data in 17 patients who underwent extracranial to intracranial bypass operations

*Following revision after 1st ICG-VA, good patency was showed on 2nd ICG-VA. MCA : middle cerebral artery, ICA : internal carotid artery, ICG-VA : indocyanine green videoangiography, OA : occipital artery, PICA : posterior inferior cereballar artery, RA : radial artery, STA : superficial temporal artery