Abstract
Objective
The aim of this study was to devise an objective clustering method for magnetoencephalography (MEG) interictal spike sources, and to identify the prognostic value of the new clustering method in adult epilepsy patients with cortical dysplasia (CD).
Methods
We retrospectively analyzed 25 adult patients with histologically proven CD, who underwent MEG examination and surgical resection for intractable epilepsy. The mean postoperative follow-up period was 3.1 years. A hierarchical clustering method was adopted for MEG interictal spike source clustering. Clustered sources were then tested for their prognostic value toward surgical outcome.
Results
Postoperative seizure outcome was Engel class I in 6 (24%), class II in 3 (12%), class III in 12 (48%), and class IV in 4 (16%) patients. With respect to MEG spike clustering, 12 of 25 (48%) patients showed 1 cluster, 2 (8%) showed 2 or more clusters within the same lobe, 10 (40%) showed 2 or more clusters in a different lobe, and 1 (4%) patient had only scattered spikes with no clustering. Patients who showed focal clustering achieved better surgical outcome than distributed cases (p=0.017).
Cortical dysplasia (CD) is the second most common pathologic entity in surgically treated epilepsy4,8,10,11,13,17). However, the postoperative seizure outcome is relatively limited when compared outcome with epilepsy associated with hippocampal sclerosis or tumor4,7,18). Recently, magnetoencephalography (MEG) has emerged as a useful tool for localization of the epileptogenic zone. MEG studies, which have attempted to determine the relationship between interictal spike source patterns and postoperative seizure outcome, have mostly dealt with children and their patient groups were mixed with several different pathologies9,15,25). Moreover, those studies used a subjective clustering method to determine clusters; none of the studies used a statistically based clustering method. One MEG study recruited children who had a single pathology of CD25). However, because they were interested in influences of complete or partial resection of areas containing MEG spike sources on surgical outcome, they did not report an association of clustering pattern with surgical outcome. There is one MEG study with adult epilepsy patients associated with CD, but that study included only four patients14). Therefore, based on the above studies alone, it is difficult to determine which features of the MEG spike source have prognostic value in CD-associated epilepsy surgery.
In this study, we developed an objective clustering method to classify MEG spike source patterns, and we determined the prognostic value of that new clustering method in adult epilepsy patients with CD. To that end, we undertook a retrospective analysis of MEG data with respect to postsurgical outcome.
Initially included were 58 patients with intractable epilepsy with histologically proven CD who underwent MEG examination and surgical operation between 2005 and 2010 at our hospital. Of that total, 15 patients with less than a 1 year follow-up, one patient younger than age of 15 years at surgery and 8 patients who had fewer than 5 MEG interictal spikes were excluded. Also excluded were 8 patients with medial temporal lobe epilepsy and one patient who had atrophy. As a result, 25 patients (mean age at surgery=29.3 years; SD=8.3; 11 females) were included in our retrospective study. The mean postoperative follow-up period was 3.1 years (SD=1.2 years). All patients provided informed consent. This study was approved by the Institutional Review Board of Seoul National University Hospital.
We classified focal CD (FCD) according to Blumcke's criteria2). FCD type I is characterized by radial (FCD type Ia) or tangential (FCD type Ib) dyslamination of the neocortex. FCD type II is characterized by cortical dyslamination and dysmorphic neurons without (type IIa) or with (type IIb) balloon cells. FCD type III was not included because, by definition, this type is associated with other pathologies.
All patients were examined using either a GE 1.5 or 3 T MRI unit (GE Horizon Echospeed) or a Siemens 1.5 T scanner (Siemens Avento system, Erlangen, Germany) both before and after surgery. The images were reconstructed offline and transferred to a UNIX workstation (HP C3750).
Cortical magnetic activity and electroencephalographic (EEG) activities were recorded simultaneously using a whole-head MEG system (VectorView™, Elekta Neuromag Oy, Helsinki, Finland) consisting of 306 channels arranged in triplets of two planar gradiometers and one magnetometer. Spontaneous MEG/EEG signals were acquired about 60 min (four 15 min epochs of data) while the patients were lying in a supine position with eyes closed in a magnetically shielded room. The sampling rate was 600 Hz with a bandpass filter of 0.1-200 Hz. A bipolar electrooculogram and an electrocardiogram were simultaneously recorded in order to monitor eye movement and cardiac artifacts. The location of the subject's head in the MEG sensor space was determined by measuring the magnetic signals from four head position indicator coils placed on the scalp. The coil locations with respect to the three anatomical landmarks, the nasion and two preauricular points, were identified by a three dimensional digitizer (FASTRAK™, Polhemus, Colchester, VT, USA). The obtained information was used to co-register the MRI, MEG, and head coordinates so that the sources of the MEG signals could be superim-posed on the subjects' MR image. After the recording, a signal-space-separation method was applied in order to reduce environmental and biological noises21,22). Each MEG interictal spike was visually inspected and localized using the single equivalent current dipole (ECD) method in Neuromag software (Elekta Neuromag, Helsinki, Finland). The ECDs were identified by a least-squares search in a spherical volume conductor model by using subsets of 20-50 channels surrounding the maximum signals. Only ECDs with a goodness-of-fit value >85% and confidence volume <3 mm3 were accepted. Accepted ECDs then were superimposed on the patients' individual MR image.
Hierarchical clustering analysis was adopted for MEG spike source clustering using SPSS 19.0 software (SPSS, Chicago, IL, USA). Euclidean distances between ECDs in each patient were calculated as input values. The hierarchical grouping starts by defining a number of clusters equal to the total number of ECDs, N. In the first grouping step the number of clusters is reduced to N-1 by combining the two closest ECDs using a complete linkage method. In successive stages the newly formed groups are linked to other ECDs until the entire ECD population is finally linked together into one cluster. To make an appropriate number of cluster groups, a dendrogram tree, which shows the result of the hierarchical clustering analysis, was cut by using a two-step criteria. First, if the ECDs projected onto the MR image were shown as clearly different clustered locations, we divided the dendrogram where the same number of clusters are grouped (Fig. 1). Second, we calculated the standard deviation (SD) of the Euclidian distances of ECDs within the same cluster. The dendrogram tree was then cut where any pair of ECDs distances within the same cluster did not exceed the 2.5 SD. The 1 SD should not exceed 10 mm. More than 3 spikes in one group was considered a cluster.
Extraoperative intracranial recording was done for all 25 patients to delineate the ictal-onset zone and to obtain functional mapping. The extent of invasive intracranial electrode implant was determined as follows4). If MRI showed a visible lesion, invasive intracranial electrodes were placed around the lesion. If MRI showed no visible lesion, invasive intracranial electrodes were placed to encompass the ictal-onset zone delineated by scalp video-EEG monitoring. When noninvasive results were discordant, invasive intracranial electrodes were implanted bilaterally as needed. After the invasive study, video-EEG monitoring was done until at least three typical seizures recorded. Resection included the whole extent of the visible lesion, if present, or the whole ictal-onset zone bound by sulci or functional cortex. In addition, underlying white matter was resected, if feasible. The surgical outcome was classified according to modified Engel criteria5).
Postoperative seizure outcome was Engel class I in 6 (24%) patients, class II in 3 (12%), class III in 12 (48%), and class IV in 4 (16%) patients. Table 1 presents a summary of the patients' profiles.
Twelve of 25 (48%) patients showed 1 cluster, 8 (32%) showed 2 clusters, 3 (12%) showed 3 clusters, 1 (4%) showed 5 clusters, and 1 (4%) had scattered spikes with no clustering. Of the patients who showed 2 or more clusters, 2 patients' clusters were located within the same lobe and 10 patients' clusters were in different lobes. We defined the presence of 1 cluster and ≥2 clusters within the same lobe as focal clustering and ≥2 cluster in different lobes and scattered spikes as distributed clustering. Patients with focal clustering had a better surgical outcome than those with distributed clustering (p=0.017, Engel I vs. II-IV) (Table 2).
We visually checked whether the MEG clustered area was resected regardless of its extent (complete or partial) by projecting the ECDs on postoperative MR images. The area that contained a MEG cluster was removed in 5 of 6 (83%) cases in the Engel class I group, 2 of 3 (67%) in Engel II, 5 of 12 (42%) in Engel III, and 1 of 4 (25%) in the Engel IV group. Patients who had resection of a MEG cluster tended to show a better surgical outcome (p=0.097) (Table 1).
Of the 25 patients, 19 (76%) had type I and 6 (24%) had type II CD (Table 2). With respect to postoperative seizure outcome, there were no statistically significant differences were found between patients having type I CD and type II CD (p=0.074). Of the 25 patients, 14 (56%) underwent frontal lobe resection, 8 (32%) temporal lobe resection, and 3 (12%) occipital lobe resection (Table 2). There was no postoperative seizure outcome difference among patients with different lobe resection areas (p=0.278).
Nine (36%) of 25 patients showed a visible lesion of focal CD on their MR images (Table 2). No statistical differences were found between normal and abnormal MRI findings with respect to surgical outcome (p=0.363).
In this study, we developed an objective method of MEG interictal spike source classification, and identified the prognostic values of MEG dipole clusters in adult epilepsy patients with CD. A hierarchical clustering method was adopted to classify MEG spike sources in 25 adult patients with histologically proven CD, who underwent surgical resection for intractable epilepsy and had at least 1 year of follow-up. The results of the MEG spike source clustering method were then tested for their prognostic value toward surgical outcome. The 14 (56%) patients in the focal clustering group achieved better outcome than the 11 (44%) in the distributed clustering group. No statistical differences were found according to the CD type and MRI findings with respect to surgical outcome.
Some researchers have aimed at determining the relationship between MEG interictal spike source patterns and postoperative seizure outcome in their studies9,15,25). However, the fact that each researcher used different criteria in classification of spike sources to describe their results makes it difficult to evaluate the prognostic values of MEG in epilepsy. Moreover, all of these clustering methods are somewhat subjective in that none used a statistically based clustering method. The hierarchical clustering method which we adopted in this study is a widely used data analysis tool in many fields, including machine learning, data mining, bioinformatics, and image analysis. It is a simple and objective method compared to other clustering methods used by previous researchers. To adopt this method in MEG spike source clustering, we simply entered the Euclidean distance matrix between ECDs as an input value. Subsequently, the obtained dendrogram depicts the clustering results by linking the closest ECDs together. By using this clustering method, we could clearly separate sub-groups of either focal or distributed cluster according to the dendrogram's clustering patterns. If other researchers who are interested in MEG spike source clustering use this hierarchical clustering method, we can expect an increase in the comparability of results among studies in the future.
Studies focused on MEG clustering with respect to surgical outcome have suggested that focal clustering of MEG spike sources and resection of that area may predict a favorable postoperative outcome. However, most of these studies have dealt with children or with mixed groups with various pathologies6,9,15,16,25). Although there is one study dealing with adult epilepsy patients associated with CD, only 4 patients were included in their study14). We studied an adult group of 25 patients with histologically proven CD. This is the largest MEG epilepsy study with the single pathology of CD in an adult group with more than a 1 year follow-up period.
As a result of the MEG spike source clustering method, 14 (56%) of our patients showed focal clustering and 11 (44%) showed distributed clustering. There are statistically significant differences in postoperative seizure outcome between these two groups : the focal clustering group achieved a better outcome than the distributed clustering group. In addition to clustering type, we also compared the clustered area with the surgically resected area as seen on postoperative MR images. The area that contained the MEG cluster was removed in 5 of 6 (83%) patients in the Engel I group, 2 of 3 (67%) in Engel II, 5 of 12 (42%) in Engel III, and 1 of 4 (25%) in the Engel IV group. Four of the 5 (80%) patients in Engel III had another cluster (other than the resected cluster) in a location remote from the actual resection area compared with only 1 of 7 (14%) patients with another cluster in the Engel I and II groups. One Engel IV patient (#25) is the only patient in our group who underwent multiple area resection. Additionally, he had an awake-lesionectomy with intra-operative sensory, motor, and language mapping to avoid injury to the eloquent cortex. Multiple area resection and hindrance of complete resection caused by the eloquent area were considered as a bad prognostic factor12). Failure to achieve a seizure free outcome despite resection of the focal clustered area in Patient #25 may result from these reasons.
On the basis of our results, we propose that the presence of focal clustered spike sources and resection of that area predict a favorable surgical outcome, whereas the presence of distributed clustering predicts a poor surgical outcome, in adult epilepsy patients with CD.
To draw firm conclusions about the relationship between MEG clustering and surgical outcome, explanation of the patients who had poor outcome despite the presence of focal clustering is necessary. This is especially important for the patients who showed no abnormality on their MR images. Many studies agree on the prognostic value of MRI-visible lesions1,19,23,26), but not many studies have elucidated the prognostic factors of the patients with normal MRI findings, especially in relation with associated electrophysiological characteristics16). Of our patients who showed no abnormal features on their MR images, 3 patients showed a poor surgical outcome (Engel class IV) despite the presence of focal MEG spike clustering. One patient (#25) had an awake-lesionectomy with multiple resection of frontal lobe as stated previously, while two other patients (#22, #23) had 1 cluster located in the posterior part of the resection area. We suggest that if the area containing MEG spike sources were resected, our non-lesional, single clustered patients might achieve favorable seizure outcome. Further intensive study with non-lesional patients is needed to confirm this suggestion.
With regard to postoperative seizure outcome and CD type, no statistically significant differences were found. Even though there is a debate about surgical outcome depending on CD type, recent studies have concluded that type II CD patients have better outcome than type I patients3,4,20,24). The discrepancy between previous studies and our results may due to the limited number of patients. Tendency to show better outcome in type II CD in our results support this idea (p=0.074). Even though many studies report better outcome in lesional cases, no surgical outcome differences were observed between patients with normal and abnormal MRI findings in our patient cohort. This could be explained by the fact that the small number of patients having abnormality on MR images in our study makes it difficult to reveal statistically significant results. Only 9 of 25 patients showed lesions on preoperative MR images. Furthermore, 5 of 6 who achieved Engel class II-IV had divergent presurgical evaluation results, including PET, ictal SPECT, video-EEG monitoring, invasive EEG, MEG, and MRI. Previous studies have reported that divergent presurgical evaluation results predict poor outcome13,16). Thus, the limited number of patients and the presence of divergent presurgical evaluation results may play roles in the lack of improved outcome in the lesional cases in our study.
Its retrospective design in a single institute is a major caveat which should be taken into account in exploiting the results of the present study. Nevertheless, in terms of the prognostic values of the MEG interictal spike cluster, this is the largest MEG study that has dealt with adult epilepsy group who had the single pathology of cortical dysplasia. In addition, this is the first study which introduces an objective method to classify the distribution of MEG interictal spike sources.
In this study, a hierarchical clustering method was adopted for MEG interictal spike source clustering in adult epilepsy patients with CD. By using this method of hierarchical clustering, we were able to delineate the value of using clustering to predict surgical outcome more clearly. The presence of focal clustered MEG interictal spike sources and subsequent resection of the clustered area predicted a favorable postoperative outcome. In contrast, the presence of a distributed cluster predicted a poor surgical outcome.
Acknowledgements
This Work was supported by the National Research Foundation of Korea (NRF) grant funded by Korea Government (MEST) (No. 2012-0000996).
References
1. Berkovic SF, McIntosh AM, Kalnins RM, Jackson GD, Fabinyi GC, Brazenor GA, et al. Preoperative MRI predicts outcome of temporal lobectomy : an actuarial analysis. Neurology. 1995; 45:1358–1363. PMID: 7617198.


2. Blümcke I, Thom M, Aronica E, Armstrong DD, Vinters HV, Palmini A, et al. The clinicopathologic spectrum of focal cortical dysplasias : a consensus classification proposed by an ad hoc Task Force of the ILAE Diagnostic Methods Commission. Epilepsia. 2011; 52:158–174. PMID: 21219302.


3. Chassoux F, Devaux B, Landré E, Turak B, Nataf F, Varlet P, et al. Stereoelectroencephalography in focal cortical dysplasia : a 3D approach to delineating the dysplastic cortex. Brain. 2000; 123:1733–1751. PMID: 10908202.


4. Chung CK, Lee SK, Kim KJ. Surgical outcome of epilepsy caused by cortical dysplasia. Epilepsia. 2005; 46(Suppl 1):25–29. PMID: 15816975.


5. Engel JJ. Engel JJ, editor. Outcome with respect to epileptic seizures. Surgical treatment of the epilepsies. 1993. New York: Raven Press;p. 609–621.
6. Fischer MJ, Scheler G, Stefan H. Utilization of magnetoencephalography results to obtain favourable outcomes in epilepsy surgery. Brain. 2005; 128:153–157. PMID: 15563514.


7. Hirabayashi S, Binnie CD, Janota I, Polkey CE. Surgical treatment of epilepsy due to cortical dysplasia : clinical and EEG findings. J Neurol Neurosurg Psychiatry. 1993; 56:765–770. PMID: 8331351.


8. Hudgins RJ, Flamini JR, Palasis S, Cheng R, Burns TG, Gilreath CL. Surgical treatment of epilepsy in children caused by focal cortical dysplasia. Pediatr Neurosurg. 2005; 41:70–76. PMID: 15942276.


9. Iida K, Otsubo H, Matsumoto Y, Ochi A, Oishi M, Holowka S, et al. Characterizing magnetic spike sources by using magnetoencephalography-guided neuronavigation in epilepsy surgery in pediatric patients. J Neurosurg. 2005; 102:187–196. PMID: 16156229.


10. Kim IM, Son EI. Surgery of intractable epilepsy associated with cortical dysplasia. J Korean Neurosurg Soc. 1999; 28:942–948.
11. Kloss S, Pieper T, Pannek H, Holthausen H, Tuxhorn I. Epilepsy surgery in children with focal cortical dysplasia (FCD) : results of long-term seizure outcome. Neuropediatrics. 2002; 33:21–26. PMID: 11930272.


12. Kral T, Clusmann H, Blümcke I, Fimmers R, Ostertun B, Kurthen M, et al. Outcome of epilepsy surgery in focal cortical dysplasia. J Neurol Neurosurg Psychiatry. 2003; 74:183–188. PMID: 12531945.


13. Lee SK, Lee SY, Kim KK, Hong KS, Lee DS, Chung CK. Surgical outcome and prognostic factors of cryptogenic neocortical epilepsy. Ann Neurol. 2005; 58:525–532. PMID: 16037972.


14. Morioka T, Nishio S, Ishibashi H, Muraishi M, Hisada K, Shigeto H, et al. Intrinsic epileptogenicity of focal cortical dysplasia as revealed by magnetoencephalography and electrocorticography. Epilepsy Res. 1999; 33:177–187. PMID: 10094429.


15. Oishi M, Kameyama S, Masuda H, Tohyama J, Kanazawa O, Sasagawa M, et al. Single and multiple clusters of magnetoencephalographic dipoles in neocortical epilepsy : significance in characterizing the epileptogenic zone. Epilepsia. 2006; 47:355–364. PMID: 16499760.


16. RamachandranNair R, Otsubo H, Shroff MM, Ochi A, Weiss SK, Rutka JT, et al. MEG predicts outcome following surgery for intractable epilepsy in children with normal or nonfocal MRI findings. Epilepsia. 2007; 48:149–157. PMID: 17241222.


17. Rosenow F, Lüders H. Presurgical evaluation of epilepsy. Brain. 2001; 124:1683–1700. PMID: 11522572.


18. Sisodiya SM. Surgery for malformations of cortical development causing epilepsy. Brain. 2000; 123:1075–1091. PMID: 10825348.


19. Spencer SS. Long-term outcome after epilepsy surgery. Epilepsia. 1996; 37:807–813. PMID: 8814092.


20. Tassi L, Colombo N, Garbelli R, Francione S, Lo Russo G, Mai R, et al. Focal cortical dysplasia : neuropathological subtypes, EEG, neuroimaging and surgical outcome. Brain. 2002; 125:1719–1732. PMID: 12135964.


21. Taulu S, Simola J. Spatiotemporal signal space separation method for rejecting nearby interference in MEG measurements. Phys Med Biol. 2006; 51:1759–1768. PMID: 16552102.


22. Taulu S, Simola J, Kajola M. Applications of the signal space separation method. IEEE Trans Signal Process. 2005; 53:3359–3372.


23. Tonini C, Beghi E, Berg AT, Bogliun G, Giordano L, Newton RW, et al. Predictors of epilepsy surgery outcome : a meta-analysis. Epilepsy Res. 2004; 62:75–87. PMID: 15519134.
24. Widdess-Walsh P, Kellinghaus C, Jeha L, Kotagal P, Prayson R, Bingaman W, et al. Electro-clinical and imaging characteristics of focal cortical dysplasia : correlation with pathological subtypes. Epilepsy Res. 2005; 67:25–33. PMID: 16181772.


25. Widjaja E, Otsubo H, Raybaud C, Ochi A, Chan D, Rutka JT, et al. Characteristics of MEG and MRI between Taylor's focal cortical dysplasia (type II) and other cortical dysplasia : surgical outcome after complete resection of MEG spike source and MR lesion in pediatric cortical dysplasia. Epilepsy Res. 2008; 82:147–155. PMID: 18774695.


26. Yun CH, Lee SK, Lee SY, Kim KK, Jeong SW, Chung CK. Prognostic factors in neocortical epilepsy surgery : multivariate analysis. Epilepsia. 2006; 47:574–579. PMID: 16529624.


Fig. 1
Hierarchical dendrogram (A) and equivalent current dipoles on MR images (B) for Patient #10. The individual spike sources (n=20) are represented along with the vertical axis. The ⊃-shaped lines connect the spike objects that are combined at each aggregation stage.
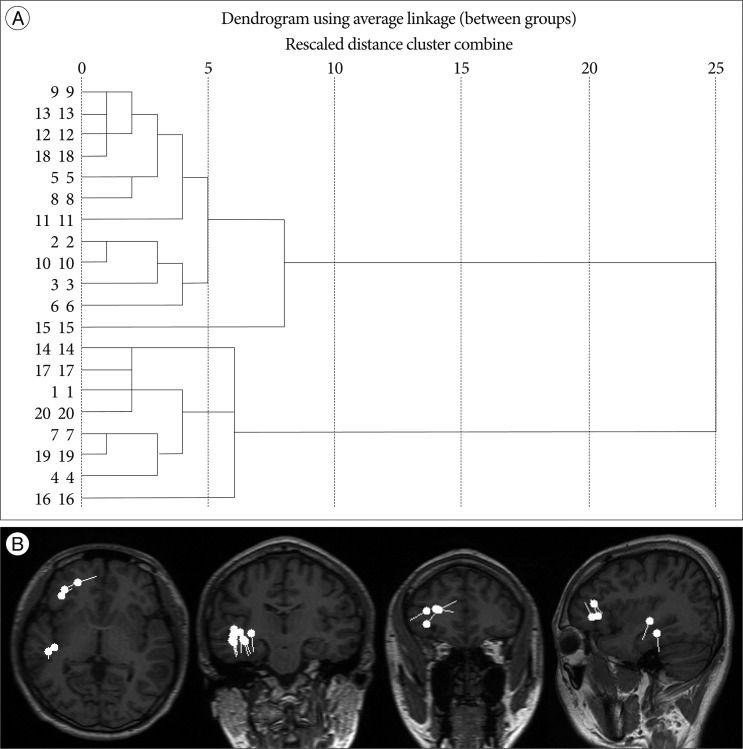