Abstract
Objective
Endovascular mechanical thrombectomy (MT) has been regarded as one of the standard treatments for acute ischemic stroke caused by large vessel occlusion. Despite the wide use of stent retrievers for MT, arterial intimal damage caused when deployed stent is pulled has been a certain disadvantage. We hypothesized that statin could protect and stabilize vessel damage after endovascular MT using a stent retriever. In this animal study, we observed the protective effects of the statins towards MT-induced vessel wall injury.
Methods
Twenty-eight carotid arteries of fourteen rabbits were used in the experiments with MT using stent retriever. We divided the rabbits into four groups as follows : group 1, negative control; group 2, positive control; group 3, statin before MT; and group 4, statin after MT. After MT procedures, we harvested the carotid arteries and performed histomorphological and immunohistochemical analyses.
Results
In histomorphological analysis with hematoxylin and eosin and Masson’s trichrome stain, significant intimal thickening (p<0.05) was observed in the positive control (group 2), compared to in the negative control (group 1). Intimal thickening was improved in the statin-administered groups (groups 3 and 4 vs. group 2, p<0.05). We also observed that statin administration after MT (group 4) resulted in a more effective decrease in intimal thickness than statin administration before MT (group 3) (p<0.05). We performed immunohistochemical analysis with the antibodies for tumor necrosis factor-alpha (TNF-α), cluster of differentiation (CD)11b, and CD163. In contrast to the negative control (group 1), the stained percentage areas of all immunological markers were markedly increased in the positive control (group 2) (p<0.05). Based on statin administration, the percentage area of TNF-α staining was significantly reduced (p<0.05) in group 3, compared to the positive control group (group 2). However, significant differences were not observed for CD11b and CD163 staining. In group 4, no significant differences were observed for TNF-α, CD11b, and CD163 staining (p≥0.05). The differences in the percentage areas of the different markers between the statin-administered groups (groups 3 and 4) were also not revealed.
Conclusion
We presented that statin administration before and after MT exerted protective effects towards vessel wall injury. The efficacy of statins was greater post-administration than pre-administration. Thus, statin administration in routine prescriptions in the peri-procedural period is strongly advised.
Intravenous (IV) infusion of recombinant tissue plasminogen activator (rt-PA) is a well-established medical treatment for acute ischemic stroke (AIS) caused by large vessel occlusion. Despite its therapeutic benefits, its recanalization rate remains under 40%, as reported in the previous literature [1,5,10,33]. Since recanalization is an influential decisive factor of good clinical outcome [5], clinicians have continued their efforts in improving its recanalization rate. Since the advent of the Merci device in 2001 [36], endovascular mechanical thrombectomy (MT) has been regarded as one of the standard AIS treatments. In 2015, five randomized controlled trials (RCTs) reported the efficacy and safety of stent retrievers [4,8,15,18,34]. These reports showed that stent retrievers, compared to IV rt-PA infusion, showed an enhanced clinical outcome and a markedly improved recanalization rate of over 80%. In this context, stent retrievers were suggested as first-line AIS treatments in the American Heart Association/American Stroke Association guidelines for early AIS management [32].
Stent retrievers have since been used widely for MT; however, certain disadvantages due to the characteristics of a self-expandable stent have been brought to light. One of them is the arterial intimal damage caused when the deployed stent is pulled in an expanded state. It is a type of direct mechanical injury, which results from friction between the arterial wall and the tightly contacted stent, which was repeatedly pulled to capture the clot. Some clinical analyses and animal studies confirmed the intimal and medial damage that occurs due to direct mechanical irritation by the stent retriever, resulting in vasospasm, intimal denudation, intimal hyperplasia, medial thickening, and inflammatory reaction [2,7,13,27,28]. These morphological changes that occurred after MT using stent retrievers were reported to cause clinical symptoms due to vessel reocclusion and stenosis in the acute and chronic phases, respectively. Regarding this concern about vessel wall injury, clinicians are attempting to reduce the number of times MT is being performed and protect the arterial wall during the procedure or promote recovery of the injured intima by using vascular protective agents.
Besides the powerful low-density lipoprotein (LDL)-lowering effect, the pleiotropic effect of statins is known to improve endothelial function, enhance atherosclerotic plaque stability, decrease oxidative stress and inflammation, and inhibit the thrombogenic response [25]. We hypothesized that statins could protect and stabilize vessel damage and reduce the inflammatory reaction that occurred after endovascular MT using a stent retriever. Thus, in this study, we observed the protective effects of statins towards MT-induced vessel wall injury using experimental animal models.
Animal experimental procedures were conducted according to the International Guide for the Care and Use of Laboratory Animals, and were approved by the Institutional Animal Care and Use Committee of Kbio Health (Osong Advanced Medical Industry Promotion Foundation, Osong, Korea; Accession number KBIO-IACUC-2019-127). The experimental study was approved by the Animal Welfare Act, and all animals received humane care. The Principles of Laboratory Animal Care formulated by the Institute of Laboratory Animal Resources (National Research Council, NIH Publication No. 85–23, revised 1996) were followed.
We chose rabbits as the experimental animal models for the following reasons. First, the diameter (about 3 mm) of the rabbit carotid artery is similar to that of the human middle cerebral artery (MCA) of the M1 segment. The commonly occluded cerebral artery in AIS in humans is the MCA, and the stent retriever used in this study was suitable for the human MCA. Although, unlike rabbit carotid artery, human MCA does not have external elastic lamina, we considered the similarity of vessel diameter between rabbit carotid artery and human MCA. Second, the action mechanisms of statins in rabbits resemble those in humans [30]. Third, the features of histopathologically and immunohistochemically stained tissues of rabbits are similar to those of humans. Fourth, rabbits possess excellent regenerative abilities and the capacity to withstand stress. Fifth, breeding and anesthetic methods for rabbits are easy to perform and well-established. Atorvastatin is a 3-hydorxy-3-methylglutaryl-coenzyme-A (HMG-CoA) reductase inhibitor that is widely used for clinical and animal model applications. In a previous study, the therapeutic and safe dosage of atorvastatin in rabbits was reported to be 3–10 mg/kg [3]. We administered 20 mg atorvastatin to rabbits orally.
Fourteen male New Zealand white rabbits (12–14 weeks, 2.6–3.0 kg; SamtacoBio, Ansan, Korea) were housed individually in metal cages for 15 days. We fed the rabbits with a regular chow diet and maintained the following cage conditions: a 12-hour light-dark cycle (150–300 Lux [light cycle from 08:00 to 20:00]), temperature at 23±3℃, and air ventilation at 10–20 times/h. There was no dropout of animals during the study period.
The experimenters, wearing lab gowns that were autoclaved (121℃, 20 minutes), handled the rabbits by following the Association for Assessment and Accreditation of Laboratory Animal Care International system guidelines.
We performed statistical power analysis using G*Power ver. 3.1 (Heinrich Heine Universität Düsseldorf, Düsseldorf, Germany) to determine the sample size [11,12]. The total sample size calculated by power analysis was 24, and we included 28 carotid arteries of 14 rabbits. We divided the rabbits into four groups as follows (Table 1) : group 1 (negative control, n=4 carotid arteries of 2 rabbits), no MT or statin administration; group 2 (positive control, n=8 carotid arteries of 4 rabbits), MT without statin administration; group 3 (statin before MT, n=8 carotid arteries of 4 rabbits), MT with statin administration for seven days before MT; group 4 (statin after MT, n=8 carotid arteries of 4 rabbits), MT with statin administration for seven days after MT.
The experimental and breeding period was 14 days. Group 1 was the negative control group, and MT was not performed for the group 1 rabbits. MT was performed on experimental day 8 for the group 2, group 3, and group 4 rabbits. Atorvastatin was not administered to the negative (group 1) and positive (group 2) control group rabbits. We administered atorvastatin (20 mg) (LipiTOR; Jeil Pharmaceutical Co., Ltd., Seoul, Korea) daily via a 5 F oral gavage tube to the group 3 and group 4 rabbits. We maintained atorvastatin administration for seven days before (day 1–7) and after (day 8–14) MT for the group 3 and group 4 rabbits, respectively. On day 15, the rabbits of all groups were euthanized using deep isoflurane (>5%) (Forane; JW Pharmaceutical, Seoul, Korea) anesthesia, followed by IV potassium chloride injection.
Rabbits were inducted to general anesthesia by administering an intramuscular tiletamine/zolazepam (15 mg/kg, Zoletil; Virbac Korea, Seoul, Korea) and xylazine (5 mg/kg, Rompun; Bayer Korea, Ansan, Korea) injection. We maintained isoflurane (<3%) anesthesia and oxygen with a respiratory anesthesia device (Fabius GS premium; Dräger Medical GmbH, Lübeck, Germany). After shaving the groin area of the rabbit, we made a skin incision (length, 2 cm) and exposed the right femoral artery by carefully dissecting the muscle and fascia layers (Fig. 1).
MT was performed using the angio machine (Artis Zee Multi-Purpose; Siemens Healthineers AG, Erlangen, Germany). The endovascular approach was achieved via the right femoral artery using a 4 F sheath. We navigated the microcatheter (Trevo Pro 18; Stryker Neurovascular, Fremont, CA, USA) through the right femoral artery to the distal carotid artery. The stent retriever (Trevo XP Provue 4×20 mm; Stryker Neurovascular, Fremont, CA, USA) was introduced into the microcatheter and deployed in the carotid artery. For tight apposition of the stent to the vessel wall, we used the ‘push and fluff technique’ while deploying the stent [16]. After 2–3 minutes, the deployed stent was pulled back to the proximal segment of the carotid artery (Fig. 2). We repeated this procedure for both carotid arteries thrice. Three consecutive MT procedures are considered acceptable in a real clinical situation, and are presumed to cause adequate vessel wall injury.
The microcatheter and femoral artery sheath were subsequently removed after MT, and the right femoral artery was ligated. Muscle and fascia were sutured with absorbable sutures, and the skin was closed with 4–0 nylon suture.
After euthanizing the rabbits, the carotid arteries were harvested. We removed both carotid arteries from the proximal to the distal segments. The excised tissues were fixed in 10% normal buffered formalin for 24 hours and subsequently embedded in paraffin blocks. The tissue cross-section was cut into 4-µm-thick slices using a microtome and mounted on microscope slides. We stained the slides with hematoxylin and eosin (H&E) and Masson’s trichrome. Additionally, the slides were stained immunohistochemically with antibodies to cluster of differentiation (CD)163 (ABCAM, Cambridge, MA, USA), CD11b (NovusBio, Littleton, CO, USA), and tumor necrosis factor-alpha (TNF-α; NovusBio, Littleton, CO, USA).
We obtained digital images of the slides using a Carl Zeiss Axio Observer 5 microscope and the Zen microscope software (Carl Zeiss AG, Jena, Germany). Histomorphometry was performed using the Image J program (National Institutes of Health, Bethesda, MD, USA). We observed the inner vessel wall mechanical injury by measuring the thickness of the tunica intima (defined as the vessel wall layer from the internal elastic lamina to the lumen) stained with H&E and Masson’s trichrome. We selected the slide with the middle segment of the carotid artery and measured the intima at the thickest position. We also evaluated the percentage areas of the tissue immunohistochemically stained with antibodies to rabbit macrophages, CD163, CD11b, and TNF-α, to measure the inflammatory reaction.
Data analysis was conducted using SPSS ver. 23.0 (SPSS Inc., Chicago, IL, USA). The variables were expressed as the mean±standard deviation, and were compared using one-way analysis of variance (ANOVA) for three or more groups with post-hoc (Duncan’s method). p-value <0.05 was considered statistically significant.
We measured the thickness of the tunica intima stained with H&E and Masson’s trichrome microscopically (Fig. 3). Significant intimal thickening was observed in group 2, while decreased intimal thickness was observed in the statin-administered groups (groups 3 and 4). We then measured the intimal thickness of the rabbits in each group by histomorphological analysis (Table 2 and Fig. 4). Significant intimal thickening (p<0.05) was observed in the positive control (group 2, 48.04±12.28 µm of H&E and 42.72±8.82 µm of Masson’s trichrome), compared to in the negative control (group 1, 3.74±0.62 µm of H&E stain and 5.84±0.63 µm of Masson’s trichrome). Intimal thickening was improved in the statin-administered groups (groups 3 and 4 vs. group 2, p<0.05). The measured thickness was 30.88±10.41 µm (H&E) and 28.36±9.86 µm (Masson’s trichrome) in group 3 and 15.86±3.19 µm (H&E) and 14.93±5.75 µm (Masson’s trichrome) in group 4. We also observed that statin administration after MT (group 4) resulted in a more effective decrease in the intimal thickness than statin administration before MT (group 3) (p<0.05).
Immunohistochemical staining of macrophage markers was performed using TNF-α, CD11b, and CD163 to evaluate the anti-inflammatory effect of statins (Fig. 5). The stained percentage areas of all immunological antibodies were markedly increased in group 2 (Table 2 and Fig. 6). In contrast to group 1 (TNF-α, 10.55±3.63 area%; CD11b, 11.15±4.95 area%; and CD163, 14.25±1.28 area%), group 2 showed significantly increased percentage areas of TNF-α (21.75±6.78 area%, p<0.05) and CD11b, (19.64±5.19 area%, p<0.05). However, percentage area of CD163 (24.54±8.59 area%) did not significantly increased (p>0.05). Although the percentage areas of the markers based on statin administration were decreased, significant differences were distinguished among the markers. In group 3, the percentage area of TNF-α staining was significantly reduced (p<0.05), but significant differences were not observed for CD11b and CD163 staining compared to group 2. In group 4, no significant differences were observed for TNF-α, CD11b, and CD163 staining (p≥0.05) compared to group 2. The differences in the percentage areas of the different markers between the statin-administered groups (groups 3 and 4) were also not revealed.
We observed the protective effects of statins towards MTinduced vessel wall injury. Intimal thickening after endothelial injury before or after statin administration, compared to that without statin administration, was found to be significantly reduced. To date, this animal experiment performed to elucidate the role of statins in vascular protection is the first report on the reduction of the after-effects of MT-induced endothelial injury. This study demonstrated that group 2 (MT without statin administration) showed increased intimal thickening, validating the irritating effects of MT on the vessel wall, and these injury-mediated vessel wall changes (intimal thickening) could be reduced by statin administration (groups 3 and 4).
Despite the remarkable improvement in the complete revascularization rate of stent retrievers used for AIS treatment, a difference between the post-procedural radiological result and clinical outcome was observed, causing great concern. One of the possible factors for this was delayed arterial re-occlusion after MT. Given that capturing and retrieving the clots by deploying the stent in the vessel and extracting it in the expanded state might damage the inner vessel wall, subsequent changes in the vessel wall were expected to occur, affecting the patency of the re-perfused vessel. One autopsy report showed vascular and parenchymal abnormalities in five patients who expired after MT [39]. Although a first generation retrieving stent was used for MT, one of the causes of death was vessel occlusion due to a subintimal dissection. Accordingly, clinical analysis of the vessel wall injury was reported. Post-procedural vasospasm and de novo stenosis and occlusion after MT using a second generation retrieving stent have been confirmed to occur in 26.0% and 3.4% of the patients, respectively [20]. In the meantime, several animal studies also demonstrated vessel wall injury associated with the use of stent retrievers [2,7,27,28]. These studies proved that pulling a fully expanded stent denudated the endothelium due to the abrasive force of the expansile stent on the vessel wall. To study the pathomechanism of re-stenosis after vessel wall injury, Perren et al. [31] gathered previous reports on animal model-based pathophysiology of this phenomenon and summarized them. They showed that once moderate shear or stress forces were exerted on the vessel wall, the tunica intima was denuded within 48 hours, resulting in platelet and monocyte accumulation at the implicated site. Between days 7 and 14, myointimal cells covered the intima, forming several thick layers at some areas. This is called myointimal hyperplasia. Thereafter, histological evolution depended on the amount of shear force applied to the vessel wall. For stress forces around 120 Pa, complete intimal recovery was expected after 4 weeks. In contrast, shear forces over 200 Pa fractured the internal elastic lamellae situated in the outermost part of the intima, leading to persistent myointimal hyperplasia. Likewise, Arai et al. [2] performed a study using an animal model in which stent retrievers of two different sizes (Solitare FR 4 mm vs. Solitare FR 6 mm) were used to compare the extent of vessel wall injury. The tendency to develop intimal thickening was more evident when Solitare FR 6 mm was used. Based on this finding, they postulated that the extent of intimal thickening was strongly correlated with the radial force of the stent. To date, it has been elucidated that stress forces applied to a vessel wall can induce a myointimal reaction, leading to transient or permanent hyperplasia. Subsequently, myointimal hyperplasia may regress or progress into severe stenosis depending on the degree of radial force exerted on the vessel wall [29,37].
In line with previous literature, our study showed a significant increase in the intimal thickening in the positive control group (48.04±12.28 µm of H&E and 42.72±8.82 µm of Masson’s trichrome), compared to in the negative control group (3.74±0.62 µm of H&E stain and 5.84±0.63 µm of Masson’s trichrome). Additionally, intimal thickening was confirmed to be associated with the inflammatory reactions elicited by direct mechanical injury of the vessel wall. TNF-α, CD11b, and CD163, the immunohistochemical stains of macrophage markers used for assessing the inflammatory reactions, were markedly increased in the positive control group (group 2). TNF-α, which was initially described as a circulating factor associated with tumor necrosis, is a key regulator of inflammatory response. It is mainly secreted by activated macrophages, and it sends signals to vascular endothelial cells, exerting pro-inflammatory changes, such as leukocyte adhesion, transendothelial migration, and vascular leak, and ultimately promoting thrombosis and plaque formation [6]. The significant increase in TNF-α in the positive control showed that MT-induced inner vessel wall injury initiated the inflammatory responses, sequentially leading to intimal thickening. Likewise, CD11b and CD163, which are markers for macrophages observed in advanced plaques, were consistently increased in the positive control group, thus supporting the evidence on the presence of an immune response against the inner vessel wall injury. Although it failed to reduce all immunohistochemical variables in the statin-administered groups (groups 3 and 4) significantly, TNF-α significantly reduced these variables in group 3. Other variables in groups 3 and 4, compared to those in the positive control group, were also substantially reduced, but failed to show statistically significant differences. Since there have been reports that statins exert prohibitory effects on atherosclerotic plaque formation by reducing macrophage content [19,24], the difference in the window period during which the rabbit carotid arteries were harvested between this study (1 week) and previous literature (2 or more weeks) was possibly the reason why our immunohistochemical results failed to show significance.
Statins, identified as fungal metabolites, are HMG-CoA reductase inhibitors, and have been on the market since 1987 [26,35,38]. Since HMG-CoA reductase is the rate-limiting enzyme for cholesterol biosynthesis in the liver, its inhibition results in decreased cholesterol production and LDL receptor up-regulation [17]. Large RCTs have proven that statins effectively prevent coronary heart disease by exerting either LDL-cholesterol-dependant or LDL-cholesterol-independent effects [25]. The LDL-cholesterol-independent effect, also known as the ‘pleiotropic’ effect, showed that the overall benefits observed due to statin treatment were more significant than those expected due to lipid level decrease. The pleiotropic effect of statins is known to improve endothelial function, enhance atherosclerotic plaque stability, decrease oxidative stress and inflammation, and inhibit the thrombogenic response [25]. Specifically, the pleiotropic effect on enhancing endothelial function is profoundly associated with the bioavailability of endothelial-derived nitric oxide (NO). Endothelial-derived NO is essential for vasodilation, platelet aggregation, vascular smooth muscle proliferation, and endothelial-leukocyte interactions [14]. By exerting this pleiotropic effect, statins can increase endothelial NO production by up-regulating endothelial NO synthase [21,22]. Besides, statins can decrease platelet-derived growth factor (PDGF)-induced DNA synthesis in vascular smooth muscle cells, leading to reduced cellular proliferation, leukocyte accumulation, and PDGF receptor phosphorylation and thus decreasing intimal thickening [9,23].
Accordingly, the pleiotropic effect of statins on the endothelium and vascular smooth muscle cell played an important role in this study. On comparing the changes in the intimal thickness after MT, we observed that the statin-administered group showed a lesser increase in intimal thickness than the control groups, showing statistically significant differences between group 2 and groups 3 and 4 (both were p=0.001).
Interestingly, the increase in intimal thickness was far lesser in group 4 than in group 3. The measured intimal thickness in group 4 was almost half of that in group 3 and one-third of that in group 2. We believe that this phenomenon was associated with the pharmacokinetic nature of statins. Since the plasma half-life of atorvastatin is 14 hours, the protective effects on the vessel after statin discontinuation will decrease abruptly. Therefore, the efficacy of statins was probably lesser in group 3 (statin pre-administered) than in group 4 (statin post-administered). Substantially, the observed findings of the changes in the intimal thickness and processed statins in group 4 were far different from those in group 3 (see the boxplots of Fig. 4). Meanwhile, this finding could be another evidence for the direct effect of statins on vessel wall protection (pleiotropic effect), and not the adjunct plasma lipid-lowering effect of statins. Nevertheless, the exact reason should be investigated in future studies.
In the present study, we used rabbit carotid arteries to investigate the vascular damage caused due to endovascular MT using stent retrievers and identify the protective effects of statins. The MT-induced endothelial injury was confirmed to occur as not only an increase in intimal thickening but also an inflammatory reaction. The increase in intimal thickening was reduced in the statin-administered groups, among which the statin post-administered group showed increased intimal thickness that was one-third of that in the non-statin-administered group and half of that in the statin pre-administered group. The efficacy of the vascular protective effects of statins was higher when the statin was given after MT than when it was given before MT.
Although this study provided insights into the protective effects of statins towards MT-induced vessel wall injury, it also showed several limitations. Because our research focused on the early protective effect of statin towards injured vessel after MT, we were unable to show the long-term changes in the intimal layer after MT-induced vessel wall injury with or without statin administration. As the first step to confirm the protective effects of statins on the vessel wall, this study could be a cornerstone for resolving the questions on the diverse clinical outcomes despite successful recanalization with MT. The serum lipid and inflammatory factor levels were not included in this study. Since statins exert both LDL-cholesterol-dependant and LDL-cholesterol-independent effects for vascular protection, measuring serum lipid levels may provide convincing evidence of the pleiotropic effect of statins.
Nevertheless, taking into account the different results of groups 3 and 4, we could observe the pleiotropic effect of statins indirectly. Lastly, a relatively small animal population was used in this study to establish the whole hypothesis of MT-induced vascular injury and the protective effects of statins towards it. A well-planned additional study with a larger sample size will add weight to the conclusion of our hypothesis. And, another experimental design using transgenic animal model of lipid metabolic disorder and another immunohistochemical stain will be considered to prove the relationship between vessel injury after MT and healing mechanism of statin administration.
Endovascular MT plays an important role in AIS, which is more common in the elderly. As the aging population increases, the use of stent retrieving devices will rise steeply. Although the stent retrieving device has proven to be effective in recanalization of large occluded vessels, vascular wall injury during blood clot extraction is a cause of great concern. In this study, we showed that MT induced endothelial changes by measuring the changes in the intimal thickness. Furthermore, we presented that statin administration before and after MT exerted protective effects towards vessel wall injury. The efficacy of statins was greater post-administration than pre-administration. Thus, including statin administration in routine prescriptions in the peri-procedural period is worth considering.
ACKNOWLEDGMENTS
This work was supported by a grant from Kyung Hee University in 2018 (KHU-20180940).
We would like to thank Jong Un Moon for the technical support in animal experiments.
References
1. Alexandrov AV, Molina CA, Grotta JC, Garami Z, Ford SR, Alvarez-Sabin J, et al. Ultrasound-enhanced systemic thrombolysis for acute ischemic stroke. N Engl J Med. 351:2170–2178. 2004.


2. Arai D, Ishii A, Chihara H, Ikeda H, Miyamoto S. Histological examination of vascular damage caused by stent retriever thrombectomy devices. J Neurointerv Surg. 8:992–995. 2016.


3. Auerbach BJ, Krause BR, Bisgaier CL, Newton RS. Comparative effects of HMG-CoA reductase inhibitors on apo B production in the casein-fed rabbit: atorvastatin versus lovastatin. Atherosclerosis. 115:173–180. 1995.


4. Berkhemer OA, Fransen PS, Beumer D, van den Berg LA, Lingsma HF, Yoo AJ, et al. A randomized trial of intraarterial treatment for acute ischemic stroke. N Engl J Med. 372:11–20. 2015.
5. Bhatia R, Hill MD, Shobha N, Menon B, Bal S, Kochar P, et al. Low rates of acute recanalization with intravenous recombinant tissue plasminogen activator in ischemic stroke: real-world experience and a call for action. Stroke. 41:2254–2258. 2010.


7. Brekenfeld C, Schroth G, El-Koussy M, Nedeltchev K, Reinert M, Slotboom J, et al. Mechanical thromboembolectomy for acute ischemic stroke: comparison of the catch thrombectomy device and the Merci Retriever in vivo. Stroke. 39:1213–1219. 2008.


8. Campbell BC, Mitchell PJ, Kleinig TJ, Dewey HM, Churilov L, Yassi N, et al. Endovascular therapy for ischemic stroke with perfusion-imaging selection. N Engl J Med. 372:1009–1018. 2015.


9. Chen Z, Fukutomi T, Zago AC, Ehlers R, Detmers PA, Wright SD, et al. Simvastatin reduces neointimal thickening in low-density lipoprotein receptor-deficient mice after experimental angioplasty without changing plasma lipids. Circulation. 106:20–23. 2002.


10. del Zoppo GJ, Poeck K, Pessin MS, Wolpert SM, Furlan AJ, Ferbert A, et al. Recombinant tissue plasminogen activator in acute thrombotic and embolic stroke. Ann Neurol. 32:78–86. 1992.


11. Faul F, Erdfelder E, Buchner A, Lang AG. Statistical power analyses using G*Power 3.1: tests for correlation and regression analyses. Behav Res Methods. 41:1149–1160. 2009.


12. Faul F, Erdfelder E, Lang AG, Buchner A. G*Power 3: a flexible statistical power analysis program for the social, behavioral, and biomedical sciences. Behav Res Methods. 39:175–191. 2007.


13. Gascou G, Lobotesis K, Machi P, Maldonado I, Vendrell JF, Riquelme C, et al. Stent retrievers in acute ischemic stroke: complications and failures during the perioperative period. AJNR Am J Neuroradiol. 35:734–740. 2014.


14. Gauthier TW, Scalia R, Murohara T, Guo JP, Lefer AM. Nitric oxide protects against leukocyte-endothelium interactions in the early stages of hypercholesterolemia. Arterioscler Thromb Vasc Biol. 15:1652–1659. 1995.


15. Goyal M, Demchuk AM, Menon BK, Eesa M, Rempel JL, Thornton J, et al. Randomized assessment of rapid endovascular treatment of ischemic stroke. N Engl J Med. 372:1019–1030. 2015.


16. Haussen DC, Rebello LC, Nogueira RG. Optimizating clot retrieval in acute stroke: the push and fluff technique for closed-cell stentrievers. Stroke. 46:2838–2842. 2015.
17. Istvan ES, Deisenhofer J. Structural mechanism for statin inhibition of HMG-CoA reductase. Science. 292:1160–1164. 2001.


18. Jovin TG, Chamorro A, Cobo E, de Miquel MA, Molina CA, Rovira A, et al. Thrombectomy within 8 hours after symptom onset in ischemic stroke. N Engl J Med. 372:2296–2306. 2015.


19. Kato M, Sada T, Mizuno M, Kitayama K, Inaba T, Koike H. Effect of combined treatment with an angiotensin II receptor antagonist and an HMG-CoA reductase inhibitor on atherosclerosis in genetically hyperlipidemic rabbits. J Cardiovasc Pharmacol. 46:556–562. 2005.


20. Kurre W, Pérez MA, Horvath D, Schmid E, Bäzner H, Henkes H. Does mechanical thrombectomy in acute embolic stroke have long-term side effects on intracranial vessels? An angiographic follow-up study. Cardiovasc Intervent Radiol. 36:629–636. 2013.


21. Laufs U, La Fata V, Plutzky J, Liao JK. Upregulation of endothelial nitric oxide synthase by HMG CoA reductase inhibitors. Circulation. 97:1129–1135. 1998.


22. Laufs U, Liao JK. Post-transcriptional regulation of endothelial nitric oxide synthase mRNA stability by Rho GTPase. J Biol Chem. 273:24266–24271. 1998.


23. Laufs U, Marra D, Node K, Liao JK. 3-Hydroxy-3-methylglutaryl-CoA reductase inhibitors attenuate vascular smooth muscle proliferation by preventing rho GTPase-induced down-regulation of p27(Kip1). J Biol Chem. 274:21926–21931. 1999.


24. Lee SG, Lee SJ, Thuy NVP, Kim JS, Lee JJ, Lee OH, et al. Synergistic protective effects of a statin and an angiotensin receptor blocker for initiation and progression of atherosclerosis. PLoS One. 14:e0215604. 2019.


27. Mordasini P, Hiller M, Brekenfeld C, Schroth G, Fischer U, Slotboom J, et al. In vivo evaluation of the Phenox CRC mechanical thrombectomy device in a swine model of acute vessel occlusion. AJNR Am J Neuroradiol. 31:972–978. 2010.


28. Mordasini P, Frabetti N, Gralla J, Schroth G, Fischer U, Arnold M, et al. In vivo evaluation of the first dedicated combined flow-restoration and mechanical thrombectomy device in a swine model of acute vessel occlusion. AJNR Am J Neuroradiol. 32:294–300. 2011.


29. Neville RF, Sidawy AN. Myointimal hyperplasia: basic science and clinical considerations. Semin Vasc Surg. 11:142–148. 1998.
30. Pecoraro V, Moja L, Dall’Olmo L, Cappellini G, Garattini S. Most appropriate animal models to study the efficacy of statins: a systematic review. Eur J Clin Invest. 44:848–871. 2014.


31. Perren F, Kargiotis O, Pignat JM, Pereira VM. Hemodynamic changes may indicate vessel wall injury after stent retrieval thrombectomy for acute stroke. J Neuroimaging. 28:412–415. 2018.


32. Powers WJ, Rabinstein AA, Ackerson T, Adeoye OM, Bambakidis NC, Becker K, et al. Guidelines for the early management of patients with acute ischemic stroke: 2019 update to the 2018 guidelines for the early management of acute ischemic stroke: a guideline for healthcare professionals from the American Heart Association/American Stroke Association. Stroke. 50:e344–e418. 2019.


33. Ribo M, Alvarez-Sabín J, Montaner J, Romero F, Delgado P, Rubiera M, et al. Temporal profile of recanalization after intravenous tissue plasminogen activator: selecting patients for rescue reperfusion techniques. Stroke. 37:1000–1004. 2006.


34. Saver JL, Goyal M, Bonafe A, Diener HC, Levy EI, Pereira VM, et al. Stent-retriever thrombectomy after intravenous t-PA vs. t-PA alone in stroke. N Engl J Med. 372:2285–2295. 2015.


36. Smith WS. Safety of mechanical thrombectomy and intravenous tissue plasminogen activator in acute ischemic stroke. Results of the multi Mechanical Embolus Removal in Cerebral Ischemia (MERCI) trial, part I. AJNR Am J Neuroradiol. 27:1177–1182. 2006.
37. Sterpetti AV, Cucina A, Lepidi S, Randone B, Stipa F, Aromatario C, et al. Progression and regression of myointimal hyperplasia in experimental vein grafts depends on platelet-derived growth factor and basic fibroblastic growth factor production. J Vasc Surg. 23:568–575. 1996.


38. Stone NJ, Robinson JG, Lichtenstein AH, Bairey Merz CN, Blum CB, Eckel RH, et al. 2013 ACC/AHA guideline on the treatment of blood cholesterol to reduce atherosclerotic cardiovascular risk in adults: a report of the American College of Cardiology/American Heart Association task force on practice guidelines. Circulation. 129:S1–S45. 2014.
Fig. 1.
Exposure of the rabbit right femoral artery. After shaving the groin area of the rabbit (A), we made a skin incision (length, 2 cm) (b). The right femoral artery was exposed by carefully dissecting the muscle and fascia layers (c).
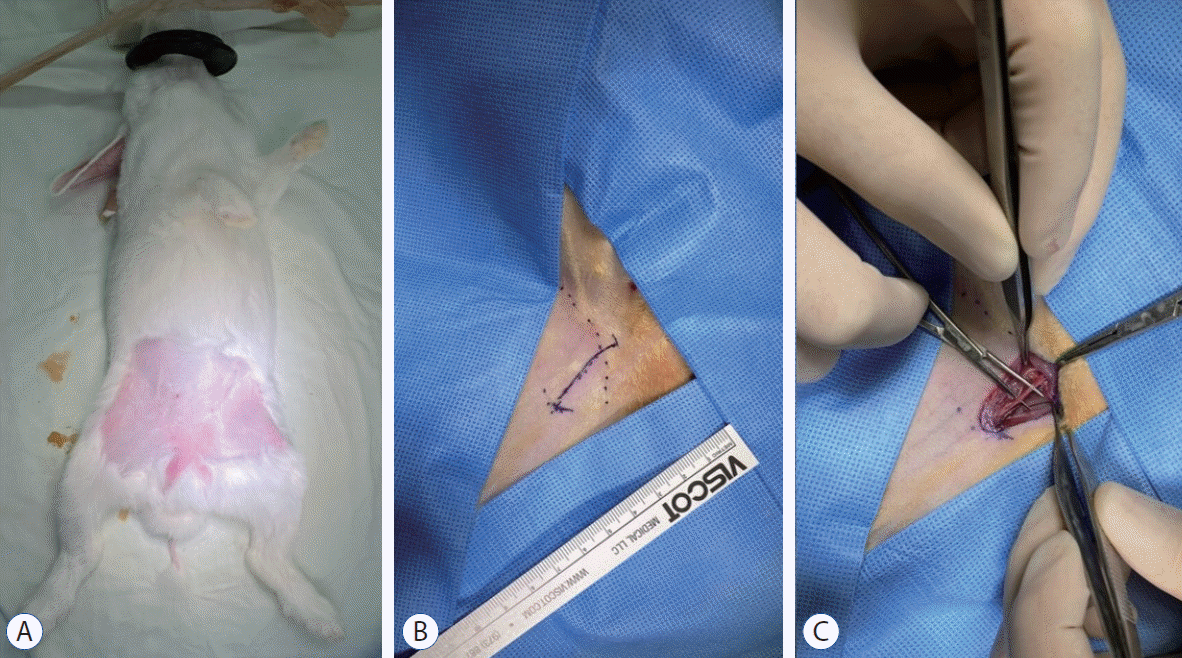
Fig. 2.
Procedure for endovascular MT using stent retrievers in the rabbit. The microcatheter was navigated via the right femoral artery to the distal carotid artery through a 4 F sheath (A). A stent retriever was introduced into the microcatheter and deployed in the carotid artery (b). For tight apposition of the stent to the vessel wall, we used the ‘push and fluff technique’ while deploying the stent (c). After 2–3 minutes, the deployed stent was pulled back to the proximal segment of the carotid artery (d).
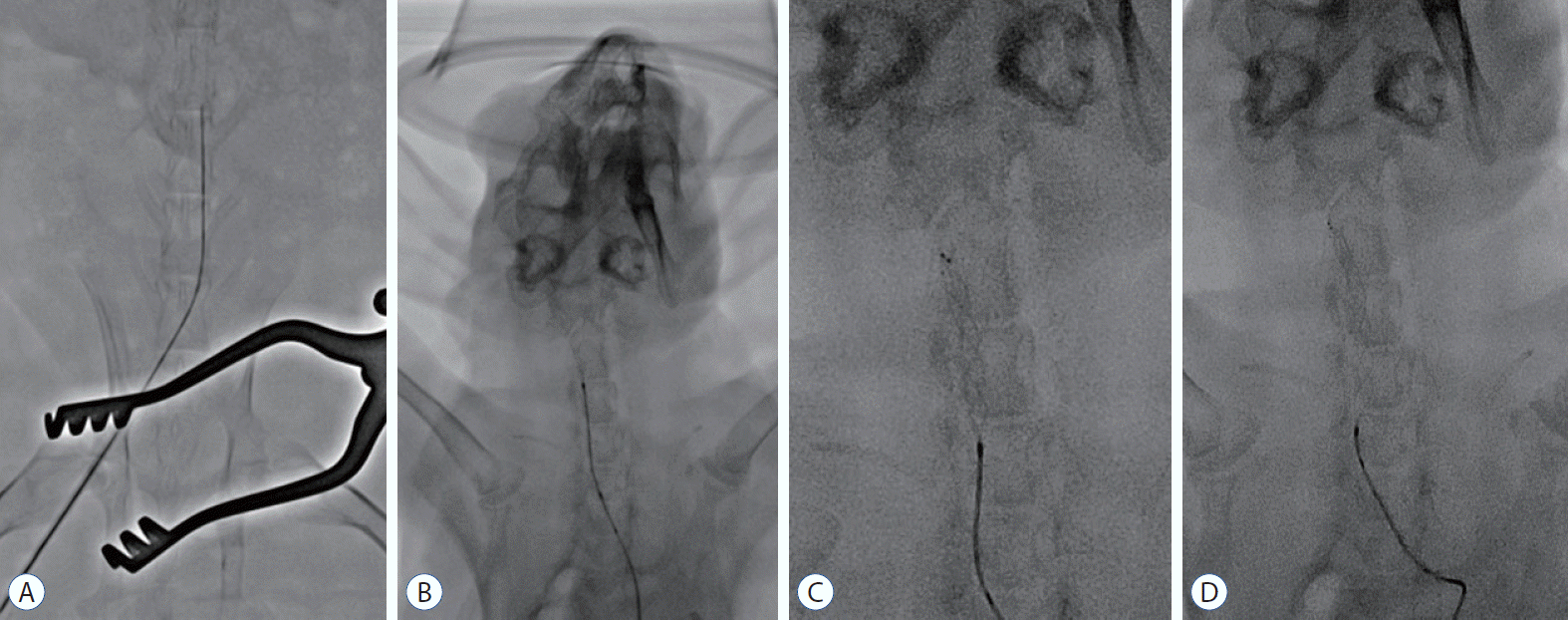
Fig. 3.
Microscopic view of the H&E and Masson’s trichrome stained tissues. The tunica intima of the negative control group (group 1) shows a single thin layer of endothelial cells. Marked thickening of tunica intima can be observed in the positive control group (group 2). In the statin-administered groups 3 and 4, a significant decrease in intimal thickening can be observed (magnification ×20 on 1st column, magnification ×200 on 2nd and 3rd columns). H&E : hematoxylin and eosin, G1 : group 1, G2 : group 2, G3 : group 3, G4 : group 4.
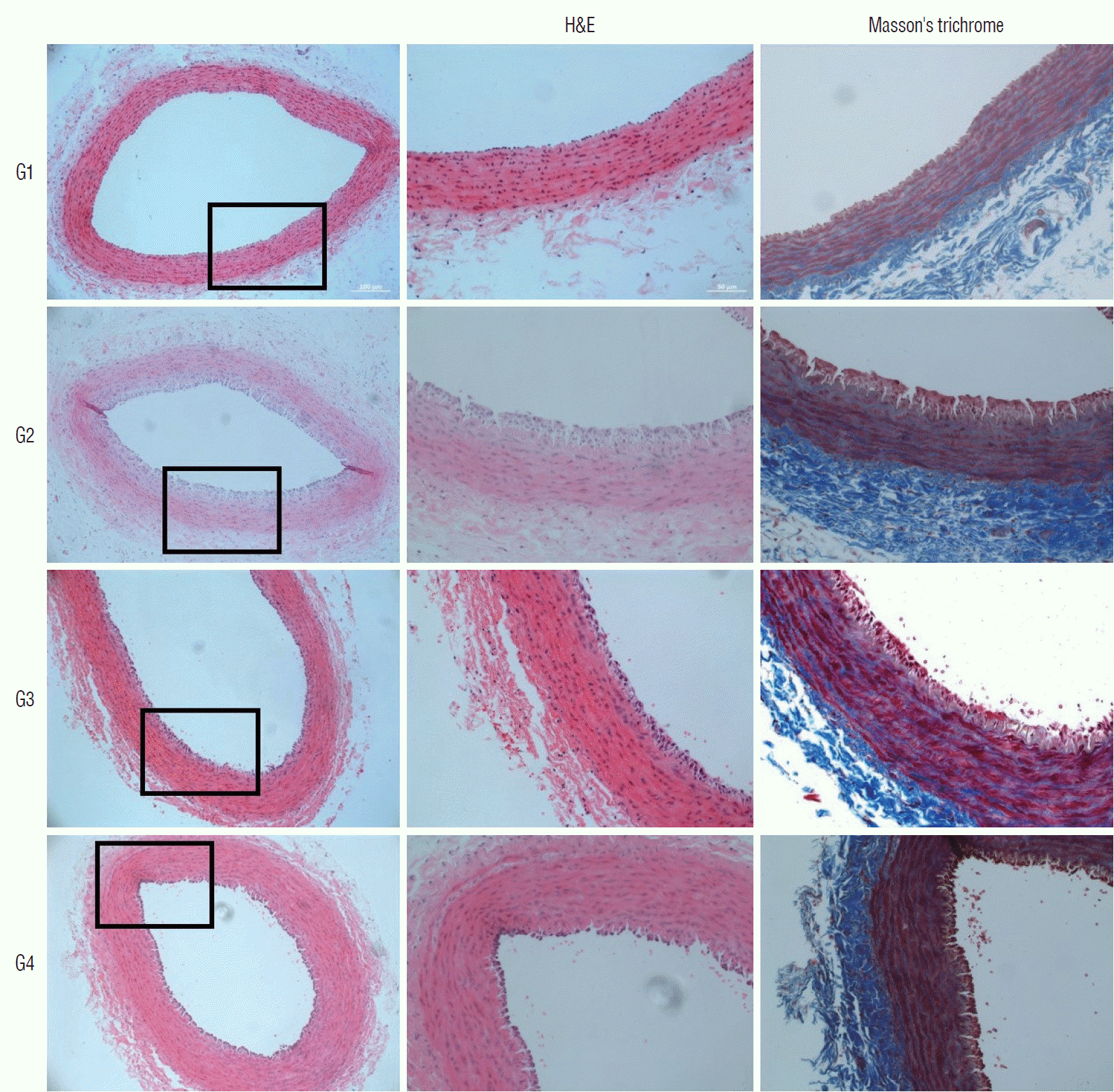
Fig. 4.
Histomorphological analysis of the intimal thickness in the hematoxylin and eosin (H&E) and Masson’s trichrome stained tissues. Marked intimal thickening was observed in the groups for which endovascular mechanical thrombectomy was performed. compared to the positive control group (group 2), the statin-administered groups (groups 3 and 4) showed decreased intimal thickness. The statistical analysis was performed using one-way analysis of variance (ANOVA) for three or more groups. Post-hoc was performed using duncan’s method. *p<0.05 vs. the positive control group. †p<0.05 between the statin-administered groups (before and after endovascular mechanical thrombectomy).
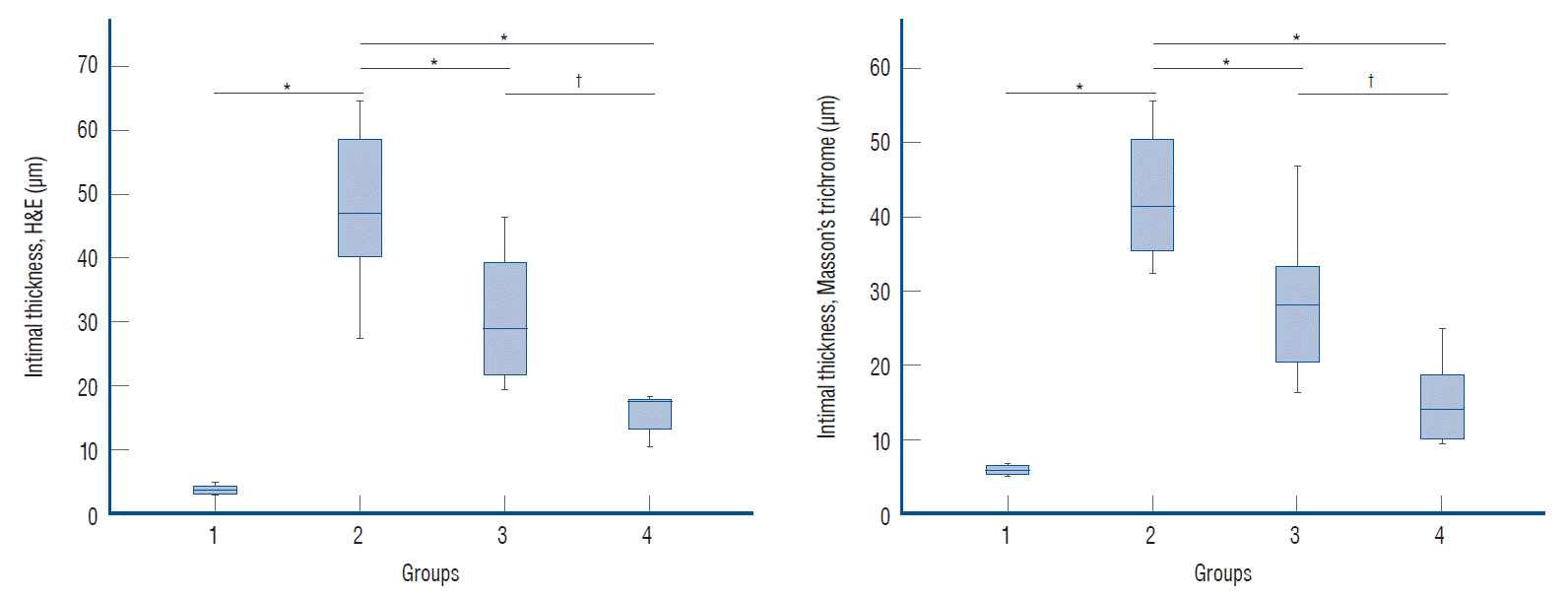
Fig. 5.
Microscopic view of the immunohistochemically stained tissues. The stained percentage areas were markedly increased in the positive control group (group 2), compared to in the negative control group (group 1). Statin administration reduced the observed percentage areas of all immunological antibodies (magnification ×200 for all samples). TNF-α : tumor necrosis factor-α, cd : cluster of differentiation, G1 : group 1, G2 : group 2, G3 : group 3, G4 : group 4.
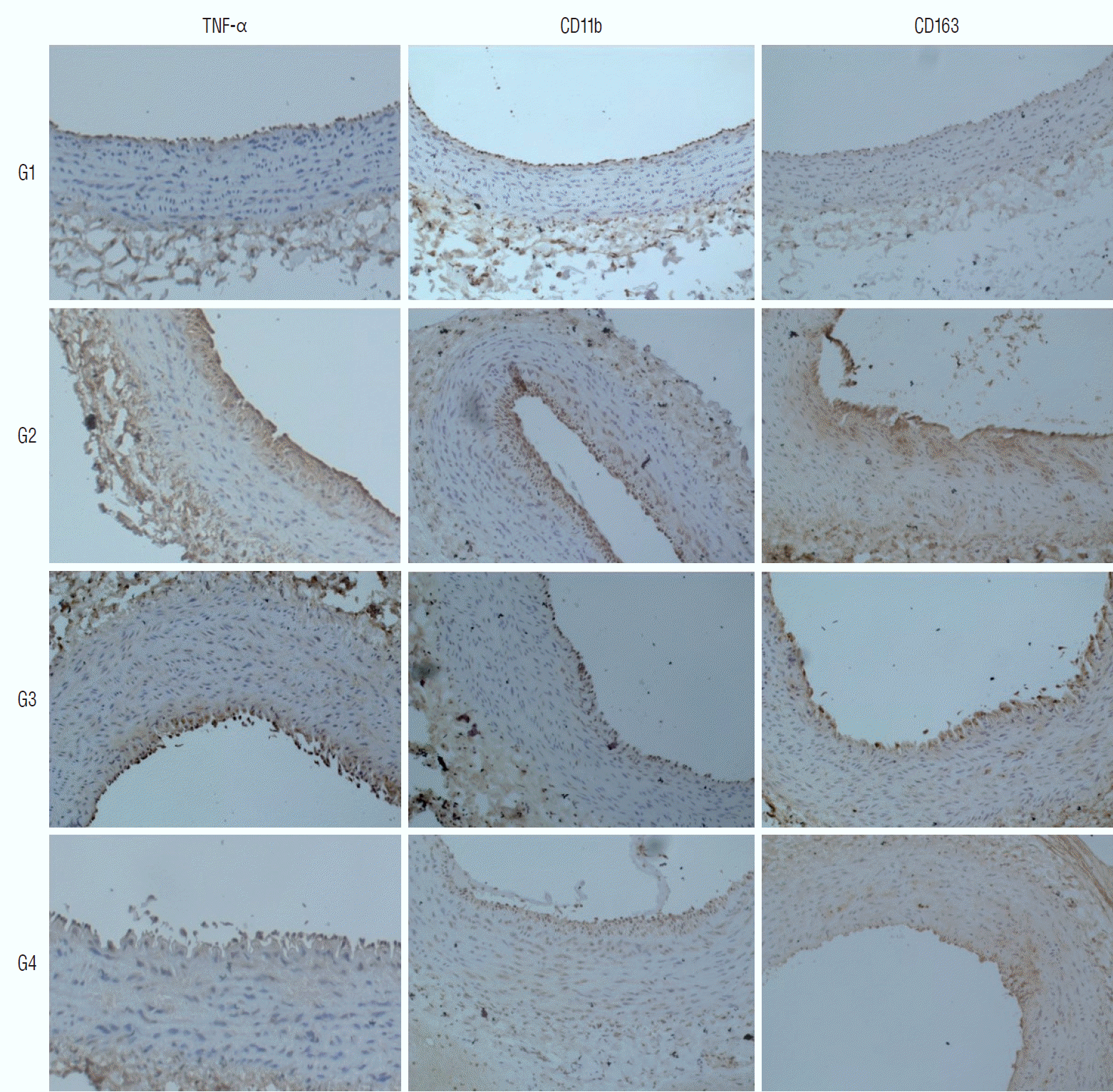
Fig. 6.
Immunohistochemical analysis of the inflammatory markers. The percentage area of all immunological antibodies significantly increased in positive control group (group 2), compared to in the negative control group (group 1). In group 3, the percentage area of TNF-α staining was significantly reduced (p<0.05), compared to the positive control group (group 2), but significant differences were not observed for cd11b and cd163 staining. In group 4, no significant differences were observed for TNF-α, cd11b, and cd163 staining (p≥0.05). The statistical analysis was performed using one-way analysis of variance (ANOVA) for three or more groups. *p<0.05 vs. positive control group. TNF-α : tumor necrosis factor-α, cd : cluster of differentiation.
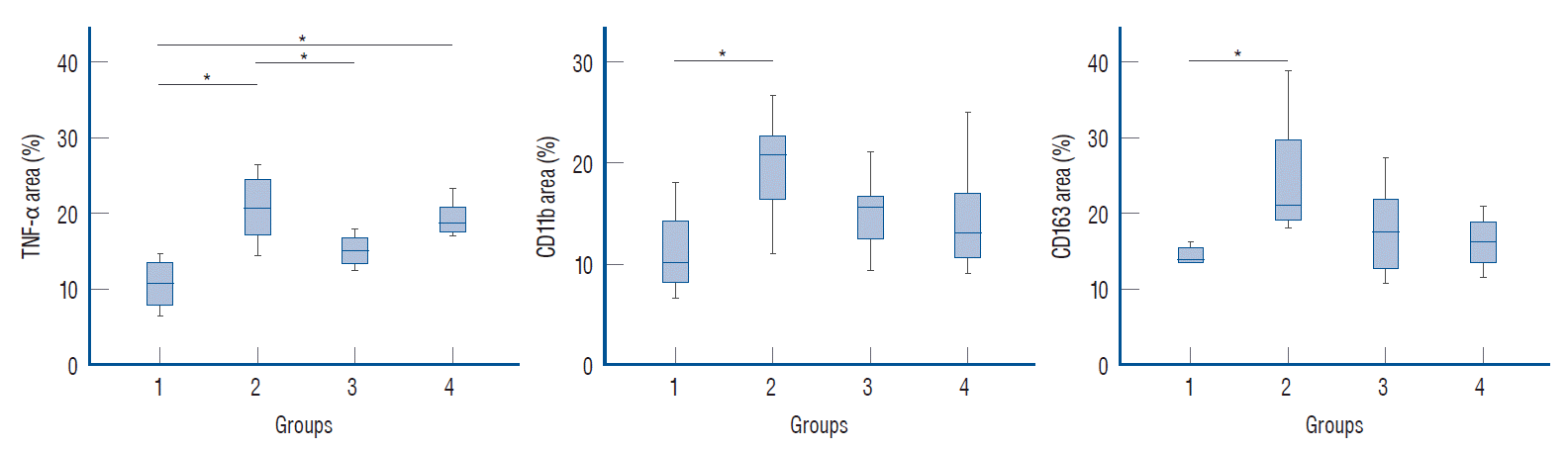
Table 1.
Study schedule and animal groups
Group 1 is the negative control group without mechanical thrombectomy (MT). MT was performed on experimental day 8 for the group 2, group 3, and group 4 rabbits. Group 2 is the positive control group without statin administration. We administered 20 mg atorvastatin daily to the group 3 (7 days before MT, day 1–7) and group 4 (7 days after MT, day 8–14) rabbits. On day 15, the rabbits of all groups were euthanized
Table 2.
Histomorphological analysis of the intimal thickness in each group
Group 1 (n=4) | Group 2 (n=8) | Group 3 (n=8) | Group 4 (n=8) | F* | Sig.(p)* | |
---|---|---|---|---|---|---|
Intimal thickness (µm) | ||||||
H&E | 3.74±0.62a | 48.04±12.28d | 30.88±10.41c | 15.86±3.19b | 28.885 | 0.001 |
Masson’s trichrome | 5.84±0.63a | 42.72±8.82d | 28.36±9.86c | 14.93±5.75b | 26.754 | 0.001 |
Immunohistochemistry (area%) | ||||||
TNF-α | 10.55±3.63a | 21.75±6.78c | 15.55±3.44a,b | 18.46±3.44b,c | 5.703 | 0.004 |
CD11b | 11.15±4.95a | 19.64±5.19b | 14.84±3.63a,b | 14.35±5.27a,b | 3.303 | 0.037 |
CD163 | 14.25±1.28 | 24.54±8.59 | 17.65±5.86 | 17.47±6.62 | 2.758 | 0.064 |