Abstract
Junctional neurulation represents the most recent adjunct to the well-known sequential embryological processes of primary and secondary neurulation. While its exact molecular processes, occurring at the end of primary and the beginning of secondary neurulation, are still being actively investigated, its pathological counterpart –junctional neural tube defect (JNTD)– had been described in 2017 based on three patients whose well-formed secondary neural tube, the conus, is widely separated from its corresponding primary neural tube and functionally disconnected from corticospinal control from above. Several other cases conforming to this bizarre neural tube arrangement have since appeared in the literature, reinforcing the validity of this entity. The cardinal clinical, neuroimaging, and electrophysiological features of JNTD, and the hypothesis of its embryogenetic mechanism, form part of this review.
Spinal dysraphic malformations are caused by embryogenetic defects of primary, secondary or junctional neurulation, which are fundamentally different processes to form the central neuraxis.
Primary neurulation involves dorsal folding and midline fusion of a neuroepithelial plate derived from the ectoderm. Due to continuity of the primitive neural plate with the cutaneous ectoderm, fusion and closure of the neural tube also preempts successful closure of the skin and mesodermal tissues. Therefore, complete failure of neural plate closure always results in an “overt” open neural tube defect, and a focal, restricted incomplete closure and spatially confined non-disjunction of the neural and cutaneous ectoderms results in limited dorsal myeloschisis as a diminutive variant within the open defect spectrum [14,31,32]. Primary neurulation ends with closure of the caudal neuropore and secondary neurulation begins sequentially with the process of mesenchymal-epithelial transition (MET), consisting of aggregation and transformation of a loosely cellular, pluripotent mesenchymal blastema into tightly adherent, apicobasally polarized epithelial cells [4,19,21,25,38]. The pluripotent mesenchymal blastema is called caudal cell mass in humans [23,33,34], and tail bud in avians [8,17,22,37,44], rodents [20] and amphibians [2]. Besides the secondary neural tube, the caudal notochord, caudal somites, most of the hind gut and urogenital tract are all derived from the caudal cell mass [23,26,40]. Following MET, secondary neurulation in chick and mouse consists of three phases – condensation of neuroprogenitor cells to form the medullary cord, cavitation of the medullary cord, and degenerative regression of most of the caudal medullary cord to form the conus (S2–5 spinal cord segments) and the filum terminale [18,36,44]. Secondary neurulation failure leads to closed neural tube defects without cutaneous opening, which include absence of condensation in agenesis of the conus in some cases of caudal agenesis [27], or failure of regression as in retained medullary cord, terminal myelocystocele, terminal lipoma, and tickened filum terminale [28,29]. Even though malformation of the conus is the main focus of a secondary neurulation defect, it is neither functionally nor anatomically disconnected from the primary neural tube.
Like most developmental anomalies, there are also mixed forms of spinal cord malformations that seem to straddle the classic types of neurulation defects and possess characteristics of both primary and secondary neurulation failures. Dorsal spinal cord lipomas, for example, have normal skin covering, though thought to arise from premature disjunction during primary neurulation, whilst transitional spinal lipomas consist of a rostral portion identical to a dorsal lipoma but also a conus clearly invaded by adipogenic mesenchyme during secondary neurulation [30].
The latest adjunct and least understood of the neurulation processes is junctional neurulation. During spinal cord development, junctional neurulation is responsible for the fusion and functional connectivity of the rostral primary neural tube with the emerging caudal secondary neural tube. Junctional neurulation therefore takes place chronologically at the end of primary neurulation and the beginning of caudal cell mass transformation.
The neurulation process varies significantly among species [19]. The chick model appears to be closest to the human spinal cord development. Morphological changes during junctional neurulation in chicks occur between Hamburger-Hamilton (HH) [18] stages 8 and 12 in a region of the neural plate known as the Node-Streak Border (NSB), i.e., the area between the rostral Hensen’s node and the caudal primitive streak [3,39]. After HH stage 12, the transient NSB disappears simultaneous with caudal neuropore closure.
At HH stage 8, the NSB contains remnants of the primitive streak medially and Hensen’s node laterally. As dorsal folding of the primary neural plate ends, Hensen’s node regresses. The distribution of neuroprogenitor cells at the NSB can be traced with in-situ hybridization for SOX-2 as an early neuronal marker, found in cells of the neural plate in the primary neural tube. Within the NSB, SOX-2 positive cells are lined up dorsally and laterally in continuity with the primary neural tube rostrally, whereas the medially and ventrally located SOX-2 negative cells represent remnants of the primitive streak. As junctional neurulation proceeds from HH 8 to 12, these ventromedial cells become SOX-2 positive, suggesting neuronal differentiation. DiI microcrystal fluorescence tracking of cells at the NSB shows the dorsolateral cell population undergo dorsal folding followed by midline fusion as expected for primary neural tube closure, whereas the ventromedial cells migrate caudally to give rise to the secondary neural tube [10]. The medial SOX-2 negative cell population meanwhile express Snail-2, Bmp-4 and N-cadherin instead of E-cadherin, evidence of mesodermal progenitor cells undergoing epithelium-to-mesenchyme transition [9,24,42]. Nevertheless, this cell population do not form mesoderm but start to express SOX-2 and ultimately become the part of the secondary neural tube overlapped by the dorsally located tip of the primary neural tube at the junctional zone [10].
Multifactorial causes for neurulation defects are discussed in the literature and specific gene mutations play a crucial role. For example, the Sonic Hedgehog signaling pathway is involved in the bending of the neural plate, and the planar cell polarity (PCP) pathway in the initiation of neural tube closure [6,7]. Genes within the PCP pathway like Prickle-1, Flamingo, Scrib, and Vangl-2 are essential for a process called convergent extension [11,45], which involves morphological cellular changes along the medio-lateral and cranio-caudal axes in cell-to-cell intercalation. The Prickle-1 gene may have a crucial role in junctional neurulation, since it is expressed mainly at the NSB and within the elongating caudal neural tube [5].
Since our initial description of the entity JNTD in 2017 [13] and presentation of the first three cases, three more cases of JNTD appeared in the literature [1,15,35]. Detailed patient data of all six published cases is summarized in Table 1. The common features of all six cases include the absence of an open skin defect, a well formed and neurologically functioning “primary” spinal cord at or above the L5 spinal cord segment, complete urinary incontinence, a hypertonic bladder and anal sphincter, partial sacral agenesis and various other forms of caudal cell mass malformations. A perfectly formed conus is present but anatomically widely separated from the “primary” spinal cord and functionally unconnected to the rest of the central neuraxis derived from the primary neural tube including the cerebral cortex. Nevertheless, the conus shows locally active reflex circuits.
In our own series, none of the patients had any cutaneous stigmata typically seen with primary neurulation defects. The dominant symptom of two patients was club feet, one diagnosed in utero, the other postnatally. All three patients presented with delayed ability to walk at age 2 years due to lower extremity weakness and foot deformity. Two patients also had congenital scoliosis.
Motor functions corresponding to the L5 and S1 spinal cord segments (i.e., within the terminal primary spinal cord) were compromised in all three patients to different degrees. There was complete atrophy and paralysis of intrinsic foot muscles suggesting non-functioning S2 spinal cord segment (within the rostral conus) in all patients.
All patients had sensory deficits with clearly diminished but discriminable L5 and S1 sensation and complete anesthesia including and caudal to S2.
All three patients had a hypertonic external anal sphincter without voluntary control. All patients presented with primary urinary incontinence. Two patients showed hydronephrosis prior to starting clean intermittent catheterization. Two patients had detrusor hyperreflexia with severe detrusor-sphincter-dyssynergia on urodynamic examination. Unfortunately, no urodynamic study was performed on the third patient.
Pathognomonic features on imaging seen in all three patients are a bluntly terminating “upper” spinal cord at the thoracolumbar vertebrae level (T11 to L1), and a “lower” spinal cord 3 to 5 vertebral levels (at L3–S2) below the rostral spinal cord, with the same spinal cord signal characteristics as the upper spinal cord and a tapering appearance resembling the typical shape of a normal conus with exiting sacral nerve roots and terminal filum. A uniform band, which is surrounded by cerebrospinal fluid, connects the upper with the lower spinal cord (Fig. 1).
Associated spinal deformities are various degrees of sacral agenesis, alone or in combination with scoliosis and/or hemivertebrae.
Electrophysiological monitoring was performed intraoperatively with comprehensive data acquisition during exploratory surgery of the first encountered patient initially thought to have a retained medullary cord [29].
The intraoperative findings were astonishing. The termination of the “upper” spinal cord was abrupt and blunt, unlike the gradual slender taper of a normal conus-to-filum transition. The structure connecting the upper to the lower spinal cord was a white, thick band without the glistening fibers of a true filum. Caudally this white band was attached to the “lower” spinal cord which showed a gradual conical taper at its caudal termination resembling the appearance of a normal conus (Fig. 2). It was richly endowed with bilateral nerve roots. In fact, the number of rootlets (7 or 8 pairs) exceeded the usual number expected from the S2–5 cord segments. The most caudal nerve roots were very puny and small, suggesting they were vestigial coccygeal roots.
Stimulating the individual ventral rootlets of the “upper” spinal cord with 0.5 milliampere (mA) current activated the rectus femoris, anterior tibialis, and gastrocnemius muscle, generating compound action potentials of equal amplitudes on each side. Bilateral anal sphincter response was absent. These findings suggested that the upper spinal cord was essentially the primary neural tube terminating at the L5 to S1 cord segments.
Equivalent stimulation of the proximal three to four pairs of nerve roots from the “lower” spinal cord activated only the external anal sphincter on the corresponding side with strong anal contractions. Distally, the effect dwindled and stimulating the last few pairs of nerve rootlets elicited no response. Direct stimulation of the lower spinal cord surface with a current of 5 mA activated both sides of the external anal sphincter, but no contractions of any lower extremity muscles (Fig. 3). Stimulation of the sensory domain of the pudendal nerve (S3–S5) generated bilateral robust bulbocavernosus reflexes with strong anal sphincter contractions having normal latencies.
Stimulating the connecting whitish band between upper and lower spinal cord with currents as high as 6 mA did not elicit any muscle response (Fig. 4).
Transcranial motor evoked potentials stimulation elicited symmetric bilateral responses from the rectus femoris, anterior tibialis, and gastrocnemius, but nothing from the abductor hallucis (S2) or external anal sphincter (S2–S4), strongly suggesting that only the primary spinal cord but not the conus was under cortical control (Fig. 5).
These electrophysiological findings all confirmed that the anatomically and functionally fully formed conus is completely isolated and physiologically unconnected to the upper primary spinal cord and the cerebral motor cortex.
The remarkable feature of JNTD is that the structural and functional connectivity between primary and secondary neural tubes is completely disrupted or unformed, but secondary neurulation has succeeded to the extent of forming a locally functioning conus with proper innervation to the bladder and external anal sphincter, albeit totally disconnected from upper corticospinal tract control. According to the recent understanding of junctional neurulation, at least some ventromedial cells of the NSB are able to express SOX-2 and become neuroprogenitor cells that migrate caudally and initialize complete secondary neurulation. Despite their intended fate, these neuroprogenitor cells fail to maintain functional and physical continuity with the dorsolateral cells of the NSB, which form the terminal primary neural tube. Other ventromedial, primitive streak derived cells, however, presumably remain SOX-2 negative and undergo epithelium-to-mesenchyme transition to form a dense mesodermal, connective tissue stalk, which is without neuronal cell properties and therefore electrophysiologically inert.
Prickle-1, as a member gene of the PCP pathway, also regulates polarised deposition of the cell adhesion molecule fibronectin on the surface of cells undergoing convergent extension during mouse gastrulation [12,16,41]. In the chick embryo, fibronectin deposition in the basement membrane of the junctional neural tube accurately matches Prickle-1 expression in the NSB. In Prickle-1 knockdown chick embryo, fibronectin distribution becomes unpolarized and haphazardly scattered [10]. Thus, impairment of neural progenitor cell adhesions in the NSB can theoretically prevent proper coupling between the dorsal and ventral cell populations, leaving them unconnected. The discordant growth rates between the neural and mesodermal components of the craniocaudal axis of the embryo consequently lead to rising of the primary neural tube rostrally towards the thoracolumbar vertebrae, leaving behind the secondary neural tube within its embryonic location in the sacral region.
Interestingly, in all JNTD patients reported here, the L5 and S1 spinal cord segments corresponding to the caudal tip of the primary neural tube were formed but functionally abnormal, with various degrees of paresis of ankle dorsi- and plantarflexion. Similarly, all patients had severe wasting of the intrinsic foot muscles, suggesting absence of S2 motor neurons and defective formation of the upper secondary neural tube segment. This picture of impaired formation of the caudal tip of the primary spinal cord (L5–S1 segments) and rostral secondary neural tube (S2 segment) suggests defective development of both types of NSB cells located at the opposing tips of the two neural tubes at the junctional neurulation zone.
Contrary to segmental spinal dysgenesis (SSD) where any spinal segment including the cervical ones may be affected, JNTD is a specific disturbance of junctional neurulation and therefore impacts only the NSB zone between the opposing extremities of the primary and secondary neural tubes. Thus, in JNTD, only the neural segments caudal to L5 are clinically affected, as shown throughout all published cases [1,13,15,35], and not the more rostral segments as commonly seen in SSD. As primary neurulation is almost completed when junctional neurulation begins, most of the primary neural tube is already formed when the embryogenic insult happens during the temporo-spatial events of junctional neurulation. As well, local formation of the secondary neural tube is primarily unaffected, though other caudal cell mass derivatives may be defective as seen in the association with sacral agenesis. Also, in JNTD, the whitish connecting band between primary and secondary neural tube is floating within a wide cerebrospinal fluid space without any bony compression, unlike most cases of SSD. It has been posited that in SSD, an initial neurulation defect also results in concurrent bony malformation at the corresponding levels, and that these latter malformations in turn cause bony compression and myelopathy [43], but the levels of myelopathy in reported cases of SSD do not usually correspond to the embryological junction between the primary and secondary neural tubes, as in JNTD. Like most developmental anomalies, there are always mixed forms of phenotypes that seem to implicate more than one neurulation mechanism, as in dorsal and transitional spinal cord lipomas [30]. Though JNTD and SSD may share some common features and malfunctioning pathways, JNTD nevertheless designates a very specific temporo-spatial neurulation defect with pathognomonic clinical and electrophysiological signatures.
JNTD is caused by genetic mutation(s) within the narrow temporospatial interlude between the termination of primary and commencement of secondary neurulation, resulting in successful formation of the secondary neural tube which nevertheless lacks anatomical and functional connection with the rest of the central neuraxis. This rare and unique neurulation defect leads to a locally functional but isolated and unintegrated conus without corticospinal inhibition or control. The handful of cases described in the literature all share common clinical features of neurogenic hypertonic bladder and bowel dysfunction, and variable loss of sensory-motor function below the L5–S1 level. The complex embryology and metabolic pathways determining complete and integrative neurulation will continue to raise controversies, encouraging critical appraisal of new scientific data as well as challenges of old paradigms, which should ultimately improve our current understanding of neurulation.
References
1. Ali M, McNeely PD. Junctional neural tube defect: a supporting case report. Childs Nerv Syst. 34:1447–1448. 2018.


2. Beck CW, Slack JM. A developmental pathway controlling outgrowth of the Xenopus tail bud. Development. 126:1611–1620. 1999.


3. Catala M, Teillet MA, De Robertis EM, Le Douarin NM. A spinal cord fate map in the avian embryo: while regressing, Hensen’s node lays down the notochord and floor plate thus joining the spinal cord lateral walls. Development. 122:2599–2610. 1996.


4. Colas JF, Schoenwolf GC. Towards a cellular and molecular understanding of neurulation. Dev Dyn. 221:117–145. 2001.


5. Cooper O, Sweetman D, Wagstaff L, Munsterberg A. Expression of avian prickle genes during early development and organogenesis. Dev Dyn. 237:1442–1448. 2008.


6. Copp AJ, Greene ND. Genetics and development of neural tube defects. J Pathol. 220:217–230. 2010.


7. Copp AJ, Greene ND, Murdoch JN. The genetic basis of mammalian neurulation. Nat Rev Genet. 4:784–793. 2003.


8. Criley BB. Analysis of the embryonic sources and mechanisms of development of posterior levels of chick neural tubes. J Morphol. 128:465–501. 1969.


9. Dady A, Blavet C, Duband JL. Timing and kinetics of E- to N-cadherin switch during neurulation in the avian embryo. Dev Dyn. 241:1333–1349. 2012.


10. Dady A, Havis E, Escriou V, Catala M, Duband JL. Junctional neurulation: a unique developmental program shaping a discrete region of the spinal cord highly susceptible to neural tube defects. J Neurosci. 34:13208–13221. 2014.


11. Doudney K, Ybot-Gonzalez P, Paternotte C, Stevenson RE, Greene ND, Moore GE, et al. Analysis of the planar cell polarity gene Vangl2 and its co-expressed paralogue Vangl1 in neural tube defect patients. Am J Med Genet A. 136:90–92. 2005.
12. Dzamba BJ, Jakab KR, Marsden M, Schwartz MA, DeSimone DW. Cadherin adhesion, tissue tension, and noncanonical wnt signaling regulate fibronectin matrix organization. Dev Cell. 16:421–432. 2009.


13. Eibach S, Moes G, Hou YJ, Zovickian J, Pang D. Unjoined primary and secondary neural tubes: junctional neural tube defect, a new form of spinal dysraphism caused by disturbance of junctional neurulation. Childs Nerv Syst. 33:1633–1647. 2017.


14. Eibach S, Moes G, Zovickian J, Pang D. Limited dorsal myeloschisis associated with dermoid elements. Childs Nerv Syst. 33:55–67. 2017.


15. Florea SM, Faure A, Brunel H, Girard N, Scavarda D. A case of junctional neural tube defect associated with a lipoma of the filum terminale: a new subtype of junctional neural tube defect? J Neurosurg Pediatr. 21:601–605. 2018.


16. Goto T, Davidson L, Asashima M, Keller R. Planar cell polarity genes regulate polarized extracellular matrix deposition during frog gastrulation. Curr Biol. 15:787–793. 2005.


17. Griffith CM, Wiley MJ, Sanders EJ. The vertebrate tail bud: three germ layers from one tissue. Anat Embryol (Berl). 185:101–113. 1992.


18. Hamburger V, Hamilton HL. A series of normal stages in the development of the chick embryo. J Morphol. 88:49–92. 1951.


19. Hughes AF, Freeman RB. Comparative remarks on the development of the tail cord among higher vertebrates. J Embryol Exp Morphol. 32:355–363. 1974.


20. Kostović-Knezević L, Gajović S, Svajger A. Morphogenetic features in the tail region of the rat embryo. Int J Dev Biol. 35:191–195. 1991.
21. Lowery LA, Sive H. Strategies of vertebrate neurulation and a re-evaluation of teleost neural tube formation. Mech Dev. 121:1189–1197. 2004.


22. Mills CL, Bellairs R. Mitosis and cell death in the tail of the chick embryo. Anat Embryol (Berl). 180:301–308. 1989.


23. Müller F, O’Rahilly R. The primitive streak, the caudal eminence and related structures in staged human embryos. Cells Tissues Organs. 177:2–20. 2004.


24. Nakaya Y, Kuroda S, Katagiri YT, Kaibuchi K, Takahashi Y. Mesenchymal-epithelial transition during somitic segmentation is regulated by differential roles of Cdc42 and Rac1. Dev Cell. 7:425–438. 2004.


25. Nievelstein RA, Hartwig NG, Vermeij-Keers C, Valk J. Embryonic development of the mammalian caudal neural tube. Teratology. 48:21–31. 1993.


26. O’Rahilly R, Müller F. Somites, spinal ganglia, and centra. Enumeration and interrelationships in staged human embryos, and implications for neural tube defects. Cells Tissues Organs. 173:75–92. 2003.
27. Pang D. Sacral agenesis and caudal spinal cord malformations. Neurosurgery. 32:755–779. discussion 778-779. 1993.


28. Pang D, Zovickian J, Lee JY, Moes GS, Wang KC. Terminal myelocystocele: surgical observations and theory of embryogenesis. Neurosurgery. 70:1383–1404. discussion 1404-1405. 2012.
29. Pang D, Zovickian J, Moes GS. Retained medullary cord in humans: late arrest of secondary neurulation. Neurosurgery. 68:1500–1519. discussion 1519. 2011.


30. Pang D, Zovickian J, Oviedo A. Long-term outcome of total and neartotal resection of spinal cord lipomas and radical reconstruction of the neural placode: part I-surgical technique. Neurosurgery. 65:511–528. discussion 528-529. 2009.
31. Pang D, Zovickian J, Oviedo A, Moes GS. Limited dorsal myeloschisis: a distinctive clinicopathological entity. Neurosurgery. 67:1555–1579. discussion 1579-1580. 2010.


32. Pang D, Zovickian J, Wong ST, Hou YJ, Moes GS. Limited dorsal myeloschisis: a not-so-rare form of primary neurulation defect. Childs Nerv Syst. 29:1459–1484. 2013.


33. Saitsu H, Yamada S, Uwabe C, Ishibashi M, Shiota K. Development of the posterior neural tube in human embryos. Anat Embryol (Berl). 209:107–117. 2004.


34. Saraga-Babic M, Krolo M, Sapunar D, Terzic J, Biocic M. Differences in origin and fate between the cranial and caudal spinal cord during normal and disturbed human development. Acta Neuropathol. 91:194–199. 1996.


35. Schmidt C, Voin V, Iwanaga J, Alonso F, Oskouian RJ, Topale N, et al. Junctional neural tube defect in a newborn: report of a fourth case. Childs Nerv Syst. 33:873–875. 2017.


36. Schoenwolf GC. Histological and ultrastructural studies of secondary neurulation in mouse embryos. Am J Anat. 169:361–376. 1984.


37. Schoenwolf GC, Delongo J. Ultrastructure of secondary neurulation in the chick embryo. Am J Anat. 158:43–63. 1980.


39. Shimokita E, Takahashi Y. Secondary neurulation: fate-mapping and gene manipulation of the neural tube in tail bud. Dev Growth Differ. 53:401–410. 2011.


40. Tam PP. The histogenetic capacity of tissues in the caudal end of the embryonic axis of the mouse. J Embryol Exp Morphol. 82:253–266. 1984.


41. Tao H, Suzuki M, Kiyonari H, Abe T, Sasaoka T, Ueno N. Mouse prickle1, the homolog of a PCP gene, is essential for epiblast apical-basal polarity. Proc Natl Acad Sci U S A. 106:14426–14431. 2009.


42. Thiery JP, Sleeman JP. Complex networks orchestrate epithelial-mesenchymal transitions. Nat Rev Mol Cell Biol. 7:131–142. 2006.


43. Wang KC, Lee JS, Kim K, Im YJ, Park K, Kim KH, et al. Do junctional neural tube defect and segmental spinal dysgenesis have the same pathoembryological background? Childs Nerv Syst. 36:241–250. 2020.


Fig. 1.
T2-weighted MRI of JNTD. A : Sagittal view shows the “upper spinal cord” (primary neural tube) ending at T12/L1, with a “cut off” stump instead of the usual taper. B : At S1/2 level the sagittal view shows the “lower spinal cord” (secondary neural tube) tapering into the filum, resembling a true conus. C : Axial view at T12 shows the “upper spinal cord”. D : Axial view at L2 shows the connecting band between the “upper” and “lower spinal cords”. E : Axial view at S1 demonstrates the “lower spinal cord” with bilateral ventral and dorsal roots. MRI : magnetic resonance imaging, JNTD : junctional neural tube defect.
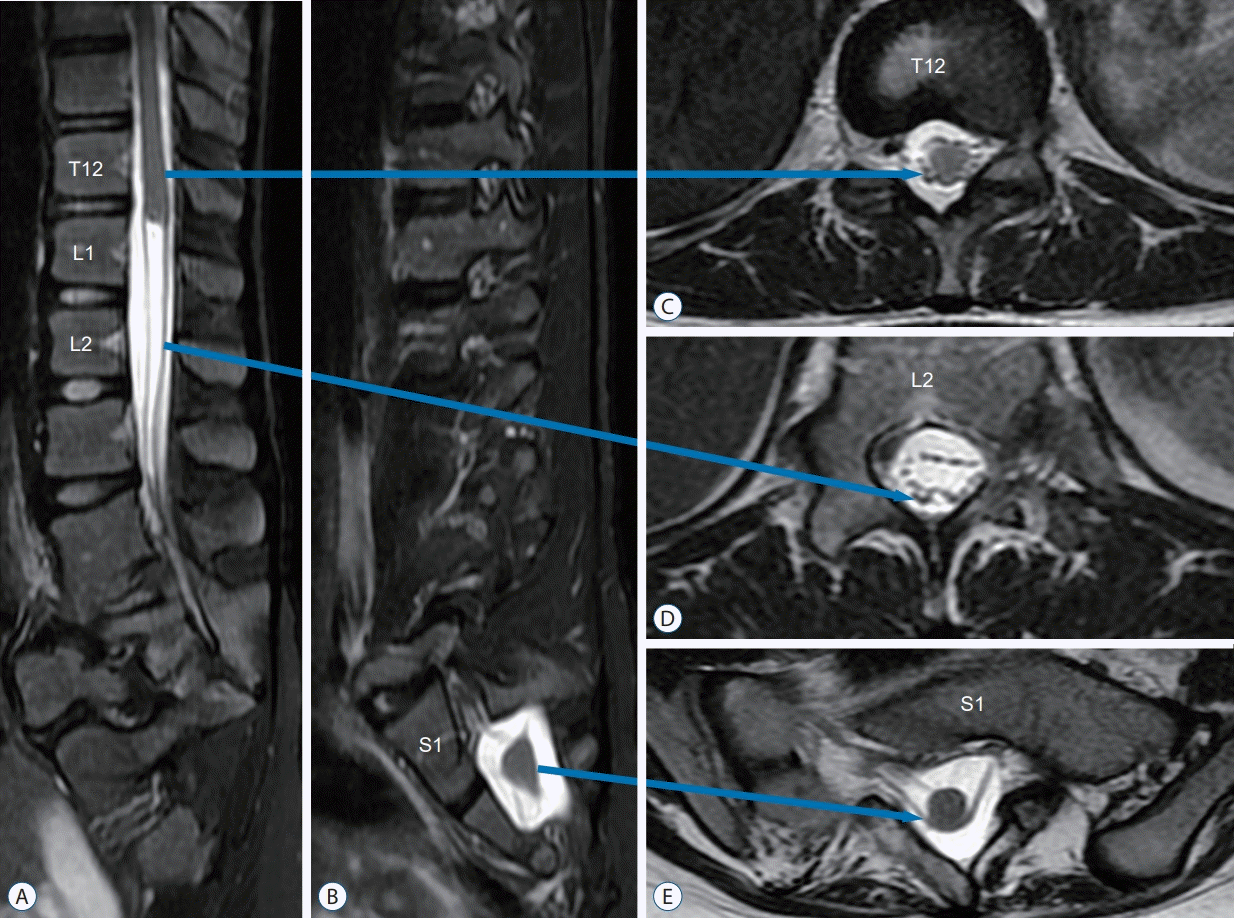
Fig. 2.
Intraoperative pictures during exploratory surgery of JNTD. A : The “upper spinal cord” (primary neural tube) is connected to the “lower spinal cord” (secondary neural tube) by a whitish soft band. B : Close-up view of this bridging band. C : Close-up view of the “upper spinal cord” (primary neural tube) showing a dense leash of nerve roots. JNTD : junctional neural tube defect.
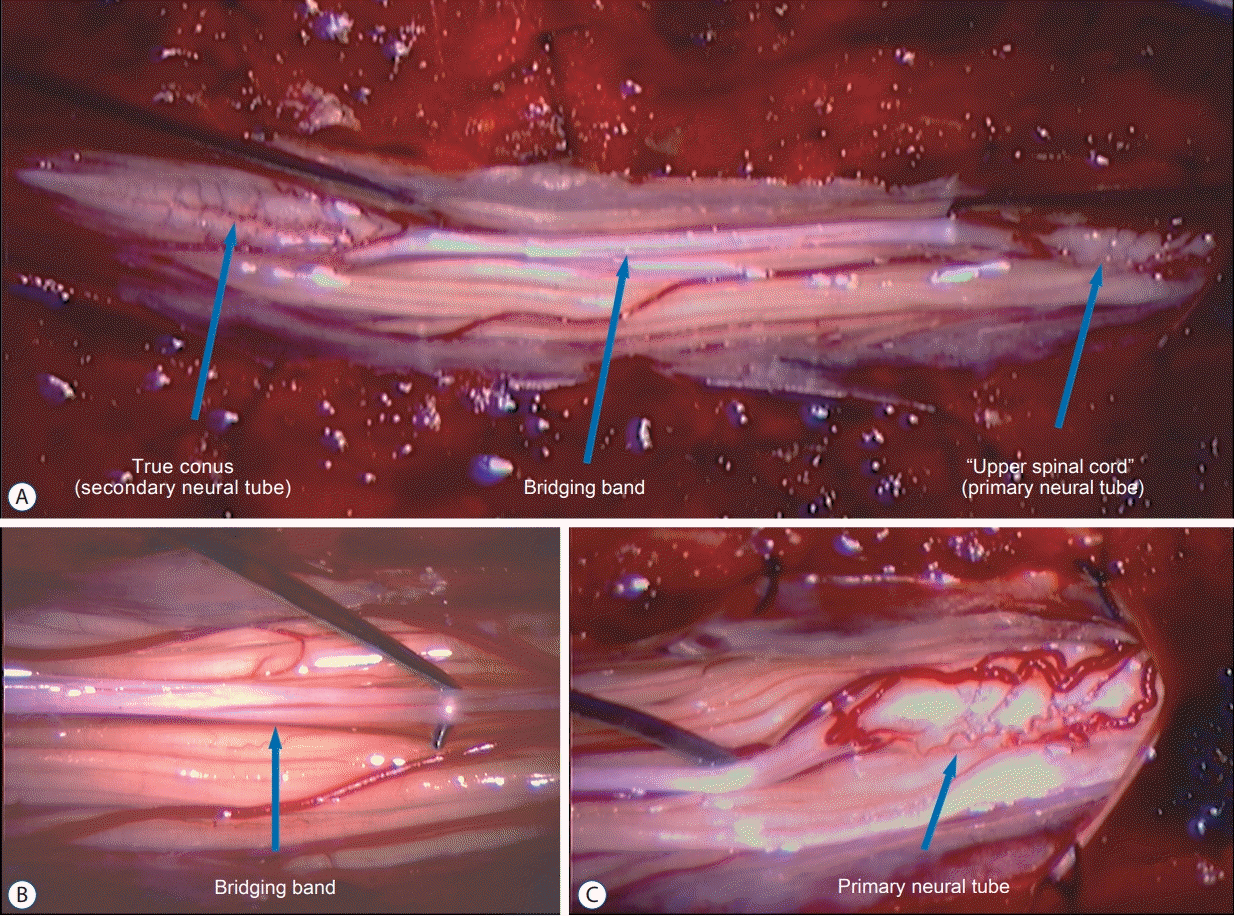
Fig. 3.
Direct stimulation of the “lower spinal cord” in JNTD elicits strong bilateral EMG responses in the external anal sphincter, indicating it is in fact a functioning conus. EMG : electromyography, L : left, Rec Fem : rectus femoris muscle, Ant Tib : anterior tibialis muscle, Gastroc : gastrocnemius muscle, Anus : sphincter ani muscle, R : right, JNTD : junctional neural tube defect.
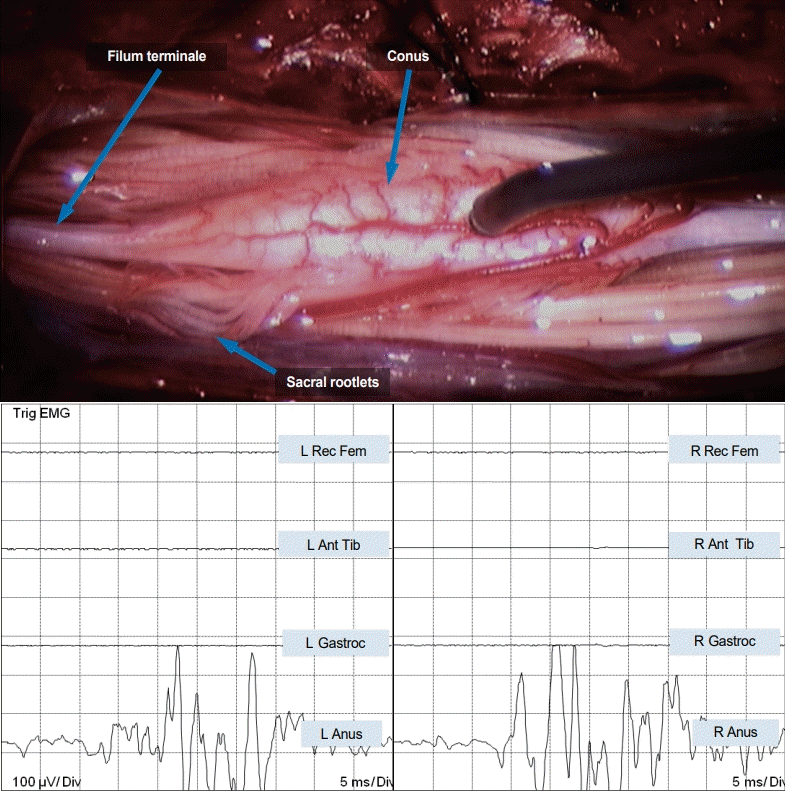
Fig. 4.
Bipolar stimulation of the non-functional, bridging band in JNTD between the “upper” and “lower spinal cord” shows no EMG response. EMG : electromyography, L : left, Rec Fem : rectus femoris muscle, Ant Tib : anterior tibialis muscle, Gastroc : gastrocnemius muscle, Anus : sphincter ani muscle, R : right, JNTD : junctional neural tube defect.
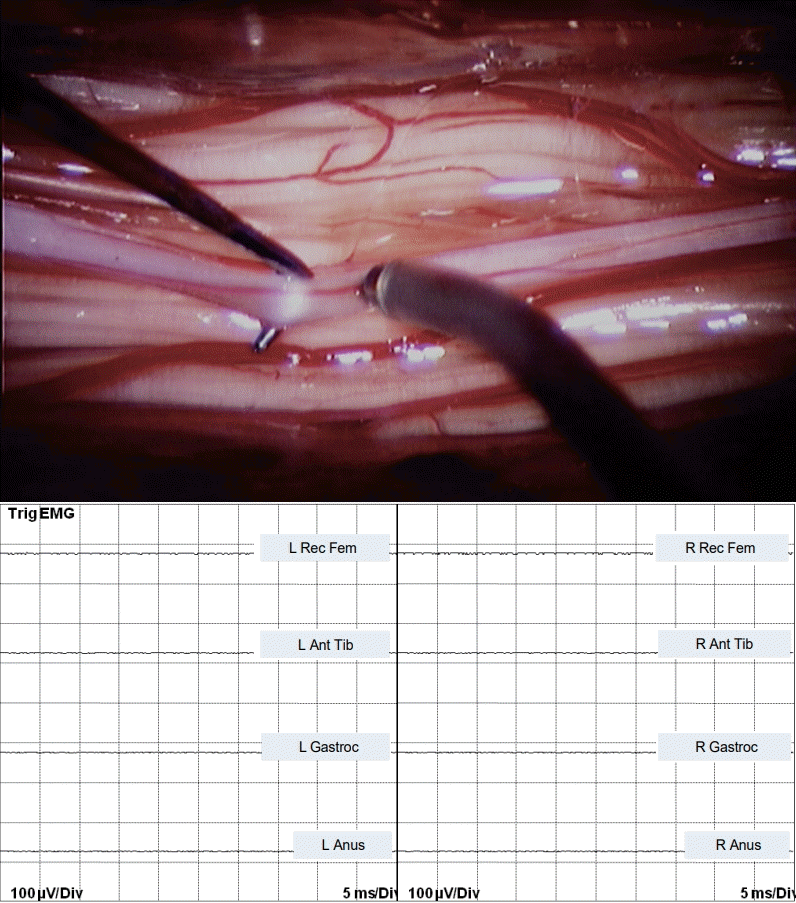
Fig. 5.
TcMEP in JNTD showing motor responses in bilateral rectus femoris, anterior tibialis, and gastrocnemius muscles. There is no motor response of the abductor hallucis or sphincter ani muscles bilaterally, indicating no functional connection to the conus (S2–S5 segments) from the motor cortex. TcMEP : transcranial motor evoked potentials, L : left, Rec Fem : rectus femoris muscle, Ant Tib : anterior tibialis muscle, Gastroc : gastrocnemius muscle, Anus : sphincter ani muscle, R : right, JNTD : junctional neural tube defect.
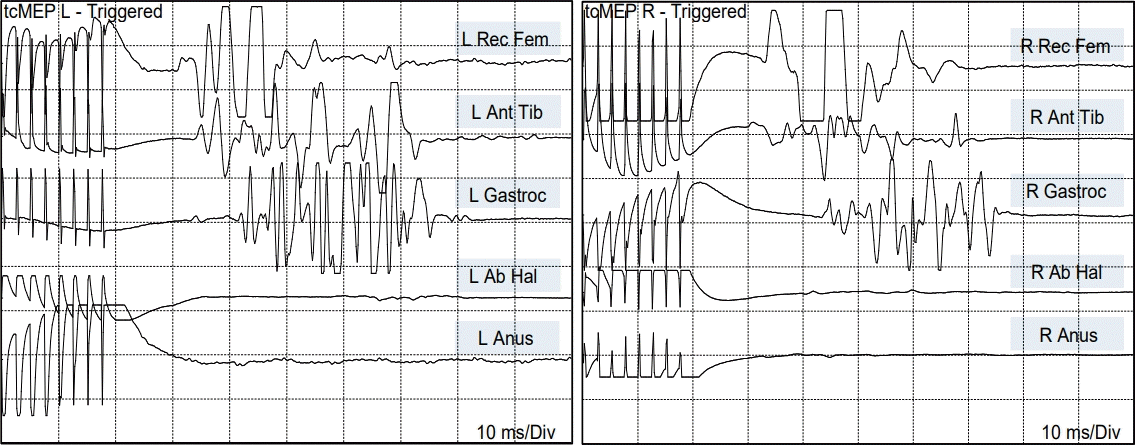
Table 1.
Published cases of junctional neural tube defect
Case | Study | Age (years) | Sex | Cutaneous sign | Presenting symptom | “Primary Spinal cord”/conus Level | Motor function | Sensation | External anal sphincter function | Kidney U/S or urodynamics | Spinal deformity/malformation |
---|---|---|---|---|---|---|---|---|---|---|---|
1 | Eibach et al. [13] | 8 | M | None | Scoliosis, delayed walking, primary urinary incontinence | L1/L3–4 | L5, S1 weakness; absent toe flaring (S2); positive BCR | Hypesthesia L5+S1; anesthesia S2–4 | Hypertonic sphincter, no voluntary contraction | Hydronephrosis | Hemivertebrae T12+L3, partial sacral agenesis scoliosis |
2 | Eibach et al. [13] | 13 | F | None | Club feet in utero, primary urinary incontinence | T12–L1/S1–2 | Weakness caudal to L5, decreased hip abduction & flaccid feet; absent toe flaring (S2) | Hypesthesia L5+S1; anesthesia S2–4 | Hypertonic sphincter, no voluntary contraction, clonic contractions of anal sphincter to perianal stimulation | Resolved hydronephrosis, detrusor hyperreflexia, DSD, spastic, small capacity, heavily trabeculated bladder | Lumbo-sacral vertebral segmentation failure |
3 | Eibach et al. [13] | 30 | F | None | Club feet, scoliosis, primary urinary incontinence | T11/L5–S1 | L5, S1 weakness; absent toe flaring (S2) | Hypesthesia L5+S1; anesthesia S2–4 | Hypertonic sphincter, no voluntary contraction | Detrusor hyperreflexia, DSD, spastic, small capacity, heavily trabeculated bladder | Partial sacral agenesis, scoliosis |
4 | Schmidt et al. [35] | Newborn | M | None | Anorectal atresia | T11/L2 | Normal newborn movements | Normal | Ano-rectal atresia | Normal U/S, no urodynamics | Partial sacral agenesis |
5 | Florea et al. [15] | 5 | M | Not specified | Club feet, delayed walking, ureterorectal fistula, anteriorly displaced anus, primary urinary incontinence | T11/L5–S1 | L5, S1 weakness and muscle atrophy | Not specified | Hypertonic sphincter | Urinary retention | Partial sacral agenesis, Filum lipoma |
6 | Aliand McNeely [1] | 28 | M | Lumbosacral dimple | Planovalgus foot deformity b/l, lower extremity atrophy, urinary incontinence | T12/L4–5 | Weakness caudal to L5, decreased hip abduction, normal dorsiflexion, decreased plantarflexion | Partial L5 hypersensitivity | Not specified | Normal U/S, no urodynamics | Sacral agenesis |