Abstract
Objective
Nerve growth factor (NGF) is a member of the neurotrophic factor family and plays a vital role in the physiological processes of organisms, especially in the nervous system. Many recent studies have reported that NGF is also involved in the regulation of tumourigenesis by either promoting or suppressing tumor growth, which depends on the location and type of tumor. However, little is known regarding the effect of NGF on interspinal schwannoma (IS). In the present study, we aimed to explored whether mouse nerve growth factor (mNGF), which is widely used in the clinic, can influence the growth of interspinal schwannoma cells (ISCs) isolated from IS in vitro.
Methods
ISCs were isolated, cultured and identified by S-100 with immunofluorescence analysis. S-100-positive cells were divided into five groups, and separately cultured with various concentrations of mNGF (0 [phosphate buffered saline, PBS], 40, 80, 160, and 320 ng/mL) for 24 hours. Western blot and quantantive real time polymerase chain reaction (PCR) were applied to detect tyrosine kinase A (TrkA) receptor and p75 neurotrophin receptor (p75NTR) in each group. Crystal violet staining was selected to assess the effect of mNGF (160 ng/mL) on ISCs growth.
Results
ISCs growth was enhanced by mNGF in a dose-dependent manner. The result of crystal violet staining revealed that it was significantly strengthened the cells growth kinetics when cultured with 160 ng/mL mNGF compared to PBS group. Western blot and quantantive real time PCR discovered that TrkA receptor and mRNA expression were both up-regualated under the condition of mNGF, expecially in 160 ng/mL, while the exoression of p75NTR demonstrated no difference among groups.
Nerve growth factor (NGF) is one of the most important growth factors in the nervous system and plays a crucial role in the regulation of neuronal development, the growth of axons, the synthesis of neurotransmitters, neuronal cell apoptosis, and inflammatory hyperalgesia [22]. NGF is composed of 2α subunits, a 1β subunit, 2γ subunits and one or two zinc ions, and the β subunit is the biologically active site [28,31]. As a member of the neurotrophin family, NGF commonly exerts biological effects by binding to specific receptors on cell surfaces, including tyrosine kinase A (TrkA) and p75 neurotrophin receptors (p75NTR) [21]. TrkA has a high affinity for NGF, and activation of the NGF-TrkA signaling pathway can promote some cell proliferation, survival or metastasis [8,9,12]. However, the p75NTR receptor often exhibits a low affinity for NGF and is highly involved in cell apoptosis [5,26]. Due to its homology with human NGF, mouse nerve growth factor (mNGF) is applied as a drug for the treatment of various central nervous system and peripheral system diseases in humans, especially for the repair of peripheral nerve injury and craniocerebral trauma [30].
Although many studies have indicated the important role of neurotrophin in the developing and mature nervous system, NGF was only recently revealed to play a role in the onset and progression of neurogenic and non-neurogenic tumors. For example, NGF can induce medulloblastoma cell apoptosis by modulating the activation of TrkA [3]. On the contrary, the overexpression of TrkA results in the promotion of breast cancer cell metastasis in immunodeficient mice [15]. Furthermore, according to the reports of Khwaja et al. [13] and Krygier and Djakiew [14], the binding of NGF to p75NTR can induce prostate tumor cell apoptosis. These observations suggest that there are large differences in the effects exerted by NGF among tumor cells, which may be dependent on the type of tumor cell line and may correspond to different transduction mechanisms of the NGF signaling pathway. Consequently, it is necessary to identify the roles of NGF in all types of tumors before considering applying mNGF to patients.
While some scholars have elucidated the effect of NGF on partial tumors in vivo and in vitro, its effect on interspinal schwannoma (IS) remains unclear. As the most common benign tumor of the spinal cord, IS generally originates from the sheath of dorsal nerve roots, an overwhelming majority of IS tumors are located in the subdural space and outside of the spinal cord [11]. IS tumors are generally categorized into two pathological types : schwannoma and neurofibromas, which are composed of Schwann cells and fibroblasts respectively. According to information statistics, the incidence of IS is approximately 0.3–0.4/100000 [7], and there are no epidemiological differences between males and females. The initial symptom of IS is spinal root pain, which is caused by the compression of tumors. Clinically, surgical resection under a microscope is the most common treatment for IS [34], although the procedure is frequently accompanied by complications [37], such as severe nerve root injury. Moreover, not all patients are suitable candidates for the surgical procedure. Although the pathological mechanisms of IS remain unclear, some researchers have implied an association of IS with NF1/NF2 gene mutations [18].
In the present study, we cultured primary interspinal schwannoma cells (ISCs) with various concentrations of mNGF to evaluate its effect in vitro. The data suggest that mNGF promotes ISC growth through the activation of TrkA receptors and p75NTR.
The study protocol was approved by the Medical Ethical Committee of Fuzhou Second Affiliated Hospital of Xiamen University, and consent was obtained from each patient involved in this study. Tumor tissue was acquired from patients who were definitively diagnosed to have IS based on preoperative magnetic resonance imaging (Fig. 1A) and postoperative pathological examinations (Fig. 1B-D). All patients underwent a microsurgical resection, and postoperative tumor spices were cautiously collected for the primary ISCs culture. ISCs were isolated according to a modified procedure by Zhang et al. [35]. Briefly, specimens were transferred to a clean bench as quickly as possible, cut into pieces, plated in a petri dish, digested by 0.2% (wt/vol) collagenase I (Sigma) in DMEM (Invitrogen/GIBCO, Grand Island, NY, USA) for 12 hours, and incubated at 37°C and 100% humidity/5% CO2. After digestion, tissue fragments were transferred to another petri dish and pre-processed with a poly-lysine solution (Beyotime) and a laminin solution (Sigma), and the culture media was changed every 2-3 days.
ISCs were identified based on the protocol reported by Zhang et al. [35]. Briefly, ISC slides were prepared by fixing with 4% paraformaldehyde for an hour and washing the slides three times for 5 minutes each with phosphate buffered saline (PBS). Then, the cell membranes were permeabilized with 0.1% Triton X-100, and after 20 minutes, the cell slides were incubated with 7.5% BSA at room temperature for 60 minutes and then washed with 1% bovine serum albumin (BSA). Subsequently, the slides were immersed in a monoclonal antiS-100 (Sigma, b subunit) solution (1 : 200) and placed in a humidified box and incubated overnight under continuous shaking at 4°C. The slides were then incubated with fluorescein isothiocyanate anti-rabbit IgG (Beyotime, 1 : 100) at room temperature for 60 minutes. Finally, 4',6-diamidino-2-phenylindole (DAPI) was used to counterstain the nuclei, and the cells were observed images under an inverted microscope. To determine the cell purity, five non-overlapping horizons were randomly selected, and the DAPI-positive (total number of cells in each horizon) and S-100-positive cells were counted in each horizon under the inverted microscope. Therefore, the cell purity was indicated as the number of anti-S-100-positive cells divided by the number of DAPI-positive cells and multiplied by 100%.
Crystal violet staining was selected to assess the effect of mNGF (Nobex; Sinobioway Medicine, Xiamen, China) on ISCs growth with the passage of time according to Chattopadhyay and Shubayev [2]. Briefly, the cells were plated in triplicate at 100 mL with 5000 cells/well in a 96-well plate and allowed to grow in complete medium with or without 160 ng/mL mNGF. then fixed with 1% glutaraldehyde for 20 minutes and stained by 0.1% crystal violet for 45 minutes at room temperature. Unbound dye was washed away with water, while bound dye was eluted with 10% acetic acid and the absorbance measured at 590 nm. The value of relative increase of absorbance versus time±standard deviation (SD) was plotted for each time-point relative to absorbance values of cells attached overnight (representing a 0 hour time-point).
Twenty-four hours in advance, ISCs were plated in a 6-well plate at a density of 1×106 cells/mL and were treated with the different concentrations of mNGF described above. Proteins were extracted using a lysis buffer (radio immunoprecipitation assay : 1% triton X-100, 1% deoxycholate, 0.1% SDS; 1 mM PMSF). The total protein was measured using a BCA Protein Assay Kit (Beyotime). Samples containing equal amounts of protein (10 µg) were run on a 10% SDS-PAGE gel and then transferred to a PVDF membrane (Millipore, Billerica, MA, USA) in Tris-glycine transfer buffer (Beyotime) at 300 mA for 90 minutes. The membranes were blocked with 5% nonfat milk (Bio-Rad), incubated with a primary antibody (rabbit monoclonal anti-TrkA antibody (Abcam, cat. no. ab76291; Cambridge, MA, USA) and rabbit monoclonal antip75NTR antibody (Abcam, cat. no. ab52987) in 5% BSA in tris buffered saline (TBS) overnight at 4°C, washed in TBS containing 0.05% (v/v) Tween 20, and incubated for 1 hour at room temperature with an HRP-conjugated anti-rabbit (Abcam) or anti-mouse secondary antibody (Abcam). The blots were developed using enhanced chemiluminescence (Beyotime) and ChemiDoc™ XRS+ System with Image Lab™ Software (Bio-Rad Laboratories, Inc., Hercules, CA, USA). All blots represent at least three independent in vitro experiments.
Primers for human p75NTR and TrkA were designed using Oligo 7.0 software (Table 1). ISCs were plated in 6-well plates at a density of 1×106 cells/mL. After 24 hours, the wells were treated with different concentrations of mNGF, as listed above, and continuously incubated for an additional 24 hours. The total RNA was extracted from each group of ISCs using an RNeasy® Mini Kit (Qiagen, Redwood, CA, USA). The RNA purity was verified by the OD260/280 absorption ratio of 2.0. cDNA was synthesized using a RevertaidTM First Strand cDNA Synthesis Kit (Thermo Scientific, Waltham, MA, USA). Gene expression was measured by quantitative real-time PCR using 50 ng cDNA and SYBR® Premix Ex TaqTM with the program (Takara Biomedical Technology [Beijing] Co., Ltd., Beijing, China) : 50°C for 2 minutes followed by 95°C for 10 minutes, 55°C for 15 seconds, and 40 cycles at 60°C for 30 seconds. Duplicate samples without cDNA (no-template control) verified no contaminating DNA, and every group corresponded to three repeated samples. Glyceraldehyde-3-phosphate dehydrogenase was used as a normalizing gene. The relative mRNA levels were quantified using the comparative cycle threshold method15). Fold changes were determined using ABI 7500 Fast software (Applied Biosystems, Inc., Waltham, MA, USA) and the described methods.
The data are expressed as the means±SD based on at least three independent experiments. Differences among groups were determined via one-way analysis of variance, and comparisons between two groups were performed with Student’s t-tests using SPSS 19.0 software (SPSS Inc., Chicago, IL, USA). p<0.05 was considered statistically significant.
Isolated ISCs from the primary cultures displayed typical bipolar or tripolar morphologies based on phase-contrast microscopy (Fig. 2A). The Schwann cells that exhibited this unique morphology were positive for S-100, whereas the negative cells were fibroblasts (Fig. 2B); all of the cell nuclei stained positive for DAPI (Fig. 2C). The purity of the isolated Schwann cells was determined based on the combination of S-100 and DAPI immunofluorescence staining (Fig. 2D). Approximately 95.77±1.18% of the Schwann cells (DAPI-positive cells) were S-100-positive in the five randomly selected horizons.
To identify the biological effect of mNGF on ISC proliferation, we generated a series of mNGF concentration gradients that included 40, 80, 160, and 320 ng/mL, and PBS was used as a control. The same numbers of cells were incubated under the mNGF and PBS conditions, and then the cell counts were estimated using a CCK-8 kit after at 24 hours. The optical density values gradually increased and ultimately reached a peak at the concentration of 160 ng/mL (Fig. 3A). Based on these data, the 160 ng/mL condition was selected for determining the ISC growth curve. Significantly enhanced ISC growth was observed after daily mNGF treatment over the course of 48 hours compared with the control group (Fig. 3B), suggesting that mNGF was correlated with ISC growth kinetics and that it induced a dose-dependent effect.
Because NGF not only mediated cell proliferation but also enhanced apoptosis via its binding with TrkA and/or p75NTR receptors, the overall effect of NGF was generally based on the quantitative distributions of TrkA and/or p75NTR receptors on the membranes of the cells. Therefore, we detected the expression of TrkA and p75NTR when the ISCs were stimulated for 24 hours by the concentrations of mNGF listed above. The western blot results demonstrated that TrkA expression increased in parallel with the increasing concentrations of mNGF, especially at 160 and 320 ng/mL, whereas p75NTR expression was stable among the groups (Fig. 4). Consistent with this finding, the relative level of TrkA mRNA was prominently modulated by the different concentrations of mNGF compared with the control group, but the relative p75NTR mRNA expression exhibited no significant changes among the groups (Fig. 5). All these data are consistent with the observed phenomenon of ISC growth under the conditions of different concentrations of mNGF.
These data are the first to imply a role of mNGF in regulating ISC proliferation, as its activation in the NGF-TrkA and NGF-p75NTR signaling pathway. Furthermore, we found that the acceleration of ISC growth was modulated by mNGF a dose-dependent manner. Unfortunately, the downstream molecular mechanisms of NGF-TrkA and NGF-p75NTR signaling have yet to be fully elucidated. Recent studies have explored the role of NGF in tumor induction and progression. In human pancreatic cancer, NGF can mediate cell growth in relation to the expression levels and ratio of TrkA and p75NTR [36]. George et al. [6] concluded that genetic mutations of TrkA do not seem to play a significant role in the activation of this pathway in prostate cancer but found an absence of mutations in otherwise genetically unstable prostate tumor DNA, which suggests that intact NGF-TrkA pathways may be important in prostate cancer development. Lagadec et al. [15] provided the first direct evidence that TrkA overexpression enhances the tumorigenic properties of breast cancer cells. Moreover, Wadhwa et al. [32] indicated that TrkA receptors are involved in tumor pathogenesis and in medullary thyroid carcinoma [19] and that they contribute to the progression of tumors toward malignancy. Accumulating data have demonstrated that the NGF-TrkA signaling pathway also participates in the growth and invasion of lung cancer cells [25] and ovarian carcinomas [4]. All these studies highlight the hypothesis that NGF and TrkA play vital roles in tumor cell development and progression. However, some researchers have also reported contrasting findings regarding the roles of NGF and TrkA in other types of tumors. For example, in neuroblastomas, high levels of TrkA expression were a sign of favorable prognosis and tended to spontaneously decrease [24]. Therefore, NGF and TrkA may play a variety of roles in different types of tumors.
p75NTR belongs to the tumor necrosis factor superfamily and is commonly associated with the induction of cell apoptosis [27], although recent studies have revealed greater effects of the activation of the NGF-p75NTR pathway. On the one hand, NGF binding to p75NTR can activate NF-κB signaling involving BEX2, which has been definitively shown to promote tumor cell survival [1]; on the other hand, in cells that co-express p75NTR and TrkA, p75NTR not only prolongs cell-surface TrkA-dependent signaling events by negatively regulates receptor ubiquitination but is also capable of regulating TrkA receptor binding properties via the neurotrophin receptor homolog-2, a transmembrane protein related to p75NTR [17,23]. Thus, the effect of NGF on tumors also depends on the expression levels of TrkA and p75NTR within the tumor cells.
Although significant efforts had been made to explore the roles of NGF in tumors, its effects on IS are not fully resolved. Furthermore, due to its benign nature, it is often ignored in studies. In our study, ISCs were isolated from the tumor tissue and treated with a series of mNGF concentrations. Interestingly, the cells proliferated well and exhibited TrkA expression and relative mRNA levels that gradually increased compared to the control group. In particular, these increases were highly significant for the 160 ng/mL treatment condition. Subsequently, we stimulated ISCs for 12, 24, 36, and 48 hours with 160 ng/mL mNGF or PBS to generate the ISC growth curve. We found that cell growth was strongly enhanced at every time point compared with the control group. However, p75NTR expression and its relative mRNA levels remained constant among all groups. These data suggest that mNGF can markedly promote ISC growth in vitro by activating the NGF-TrkA and NGF-p75NTR signaling pathways. Moreover, the activation of p75NTR in ISCs causes the exposure of high-affinity binding sites on NGF, thus improving the binding rate of NGF with TrkA but not inducing cell apoptosis.
mNGF (Enjingfu) is manufactured as a neurotrophic drug by Sinobioway Biomedicine Co., Ltd. (Xiamen, China) and is widely used in therapies for both central and peripheral nerve system diseases, such as spinal injury, traumatic brain injury, diabetic peripheral neuropathy, and traumatic peripheral injury. A recent study demonstrated that radiation-induced temporal lobe necrosis was successfully treated with mNGF33). However, our results showed that mNGF promoted the growth of ISC in vitro, which suggests that it is not appropriate for use in situations where 1) IS is so complex that it cannot be completely resected, such as in dumbbell-type tumors; 2) the size of the tumor is generally small, and the patient has no clinical symptoms; 3) the patient has another severe diseases and cannot tolerate operation; 4) the tumor is located in the C1-2 segment, wrapping the vertebral artery and rendering it unsuitable for operation; or 5) the IS is malignant. Therefore, it is recommended to avoid treating patients who are diagnosed with IS with mNGF or similar drugs. Moreover, base expression levels of TrkA and p75NTR were detected in the control ISCs, which may highlight that a limited amount of NGF, primarily secreted by adjacent nerve fibers or other healthy tissue, exists in the microenvironment of IS to maintain cell survival. In breast cancer and oesophageal squamous cell carcinoma, NGF and TrkA frequently shows overexpression and a autocrine loop of NGF is also observed, which results in a low survival rate of patients [10,29]. Therefore, abolishing the NGF-TrkA signaling pathway with a Trk tyrosine kinase inhibitor, such as CEP-701 [20], could be a potential approach for tumor therapy in the future.
NGF is indispensable for normal organisms, but its effects in some diseases are unpredictable, especially in tumors. In our study, we find that exogenous mNGF markedly stimulated the growth of ISCs and increased the expression of TrkA. Although there was no difference among groups, p75NTR still had a little expression and may play a subsidiary role of TrkA. According to these phenomenon, it may be prudent to administer mNGF for clinicians. However, the conclusion is based on in vitro experiments, therefore, in vivo research will be lauched to reveal the effect of mNGF on IS patients and indicate its cinical application.
Notes
References
1. Ahmad I, Yue WY, Fernando A, Clark JJ, Woodson EA, Hansen MR. p75NTR is highly expressed in vestibular schwannomas and promotes cell survival by activating nuclear transcription factor kappa B. Glia. 62:1699–1712. 2014.


2. Chattopadhyay S, Shubayev VI. MMP-9 controls Schwann cell proliferation and phenotypic remodeling via IGF-1 and ErbB receptor-mediated activation of MEK/ERK pathway. Glia. 57:1316–1325. 2009.


3. Chou TT, Trojanowski JQ, Lee VM. A novel apoptotic pathway induced by nerve growth factor-mediated TrkA activation in medulloblastoma. J Biol Chem. 275:565–570. 2000.


4. Davidson B, Reich R, Lazarovici P, Nesland JM, Skrede M, Risberg B, et al. Expression and activation of the nerve growth factor receptor TrkA in serous ovarian carcinoma. Clin Cancer Res. 9:2248–2259. 2003.
5. Friedman WJ. Proneurotrophins, seizures, and neuronal apoptosis. Neuroscientist. 16:244–252. 2010.


6. George DJ, Suzuki H, Bova GS, Isaacs JT. Mutational analysis of the TrkA gene in prostate cancer. Prostate. 36:172–180. 1998.


7. Gu R, Liu JB, Zhang Q, Liu GY, Zhu QS. MRI diagnosis of intradural extramedullary tumors. J Cancer Res Ther. 10:927–931. 2014.


8. Haase G, Pettmann B, Raoul C, Henderson CE. Signaling by death receptors in the nervous system. Curr Opin Neurobiol. 18:284–291. 2008.


9. Hellebrand EE, Varbiro G. Development of mitochondrial permeability transition inhibitory agents: a novel drug target. Drug Discov Ther. 4:54–61. 2010.
10. Hondermarck H. Neurotrophins and their receptors in breast cancer. Cytokine Growth Factor Rev. 23:357–365. 2012.


11. Jeon JH, Hwang HS, Jeong JH, Park SH, Moon JG, Kim CH. Spinal schwannoma; analysis of 40 cases. J Korean Neurosurg Soc. 43:135–138. 2008.


12. Johnson TV, Bull ND, Martin KR. Neurotrophic factor delivery as a protective treatment for glaucoma. Exp Eye Res. 93:196–203. 2011.


13. Khwaja F, Tabassum A, Allen J, Djakiew D. The p75(NTR) tumor suppressor induces cell cycle arrest facilitating caspase mediated apoptosis in prostate tumor cells. Biochem Biophys Res Commun. 341:1184–1192. 2006.


14. Krygier S, Djakiew D. The neurotrophin receptor p75NTR is a tumor suppressor in human prostate cancer. Anticancer Res. 21:3749–3755. 2001.
15. Lagadec C, Meignan S, Adriaenssens E, Foveau B, Vanhecke E, Romon R, et al. TrkA overexpression enhances growth and metastasis of breast cancer cells. Oncogene. 28:1960–1970. 2009.


16. Livak KJ, Schmittgen TD. Analysis of relative gene expression data using real-time quantitative PCR and the 2−ΔΔCT method. Methods. 25:402–408. 2001.


17. Makkerh JP, Ceni C, Auld DS, Vaillancourt F, Dorval G, Barker PA. p75 neurotrophin receptor reduces ligand-induced Trk receptor ubiquitination and delays Trk receptor internalization and degradation. EMBO Rep. 6:936–941. 2005.


18. Martinez-Glez V, Franco-Hernandez C, Alvarez L, de Campos JM., Isla A, Vaquero J, et al. Meningiomas and schwannomas: molecular subgroup classification found by expression arrays. Int J Oncol. 34:493–504. 2009.


19. McGregor LM, McCune BK, Graff JR, McDowell PR, Romans KE, Yancopoulos GD, et al. Roles of trk family neurotrophin receptors in medullary thyroid carcinoma development and progression. Proc Natl Acad Sci U S A. 96:4540–4545. 1999.


20. Miknyoczki SJ, Dionne CA, Klein-Szanto AJ, Ruggeri BA. The novel Trk receptor tyrosine kinase inhibitor CEP-701 (KT-5555) exhibits antitumor efficacy against human pancreatic carcinoma (Panc1) xenograft growth and in vivo invasiveness. Ann N Y Acad Sci. 880:252–262. 1999.


21. Mitsiadis TA, Pagella P. Expression of nerve growth factor (NGF), TrkA, and p75(NTR) in developing human fetal teeth. Front Physiol. 7:338. 2016.


22. Mizumura K, Murase S. Role of nerve growth factor in pain. Handb Exp Pharmacol. 227:57–77. 2015.


23. Murray SS, Perez P, Lee R, Hempstead BL, Chao MV. A novel p75 neurotrophin receptor-related protein, NRH2, regulates nerve growth factor binding to the TrkA receptor. J Neurosci. 24:2742–2749. 2004.


24. Nakagawara A, Arima-Nakagawara M, Scavarda NJ, Azar CG, Cantor AB, Brodeur GM. Association between high levels of expression of the TRK gene and favorable outcome in human neuroblastoma. N Engl J Med. 328:847–854. 1993.


25. Ricci A, Greco S, Mariotta S, Felici L, Bronzetti E, Cavazzana A, et al. Neurotrophins and neurotrophin receptors in human lung cancer. Am J Respir Cell Mol Biol. 25:439–446. 2001.


26. Salehi AH, Xanthoudakis S, Barker PA. NRAGE, a p75 neurotrophin receptor-interacting protein, induces caspase activation and cell death through a JNK-dependent mitochondrial pathway. J Biol Chem. 277:48043–48050. 2002.


27. Skaper SD. The neurotrophin family of neurotrophic factors: an overview. Methods Mol Biol. 846:1–12. 2012.


29. Tsunoda S, Okumura T, Ito T, Mori Y, Soma T, Watanabe G, et al. Significance of nerve growth factor overexpression and its autocrine loop in oesophagealsquamous cell carcinoma. Br J Cancer. 95:322–330. 2006.


30. Ullrich A, Gray A, Berman C, Dull TJ. Human β-nerve growth factor gene sequence highly homologous to that of mouse. Nature. 303:821–825. 1983.


31. Varon S, Nomura J, Shooter EM. The isolation of the mouse nerve growth factor protein in a high molecular weight form. Biochemistry. 6:2202–2209. 1967.


32. Wadhwa S, Nag TC, Jindal A, Kushwaha R, Mahapatra AK, Sarkar C. Expression of the neurotrophin receptors Trk A and Trk B in adult human astrocytoma and glioblastoma. J Biosci. 28:181–188. 2003.


33. Wang X, Ying H, Zhou Z, Hu C, Eisbruch A. Successful treatment of radiation-induced temporal lobe necrosis with mouse nerve growth factor. J Clin Oncol. 29:e166–e168. 2011.


34. Yang T, Wu L, Deng X, Yang C, Xu Y. Clinical features and surgical outcomes of intramedullary schwannomas. Acta Neurochir (Wien). 156:1789–1797. 2014.


35. Zhang K, Chen S, Lin J, Zhang Y, Yu W, Liu S, et al. Primary culture of human interspinal schwannoma. J Biomater Tissue Eng. 6:263–269. 2016.


36. Zhu ZW, Friess H, Wang L, Bogardus T, Korc M, Kleeff J, et al. Nerve growth factor exerts differential effects on the growth of human pancreatic cancer cells. Clin Cancer Res. 7:105–112. 2001.
Fig. 1.
MRI and pathological characteristics of IS. A : Contast enhanced sagittal T1A weighted MRI showing the tumor. B : Tumor tissue was stained by HE staining (×100). C : Tumor tissue was stained for S-100 (brown; ×100). D : Tumor tissue was stained for GFAP (brown; ×100). MRI : magnetic resonance imaging, IS : interspinal schwannoma, HE : hematoxylin-eosin, GFAP : glial fbrillary acidic protein.

Fig. 2.
Morphological characteristics of the primary cultured ISCs. A : ISCs were isolated from tumor tissue blocks after 48 hours of primary culture and demonstrated typical bipolar or tripolar morphologies (×100). B : ISCs were brightly stained for S-100 (green; ×100). C : ISCs and fibroblasts (white arrow) nuclei counterstained by DAPI (blue; ×100). D : Merged image and fibroblasts were not stained for S-100 (white arrow). Scale bars, 100 μm. ISCs : interspinal schwannoma cells, DAPI : 4',6-diamidino-2-phenylindole, dihydrochloride.

Fig. 3.
mNGF can accelerate the growth of ISCs. A : The effect of different mNGF concentrations on ISCs was dose-dependent, and the peak effect was observed for the concentration of 160 ng/mL. B : ISC growth curve studies using crystal violet with and without daily 160 ng/mL mNGF stimulation for 12, 24, 36, and 48 hours. The data represent the mean±standard error. *p<0.001. †p<0.05. ‡p<0.01. mNGF : mouse nerve growth factor, ISCs : interspinal schwannoma cells.
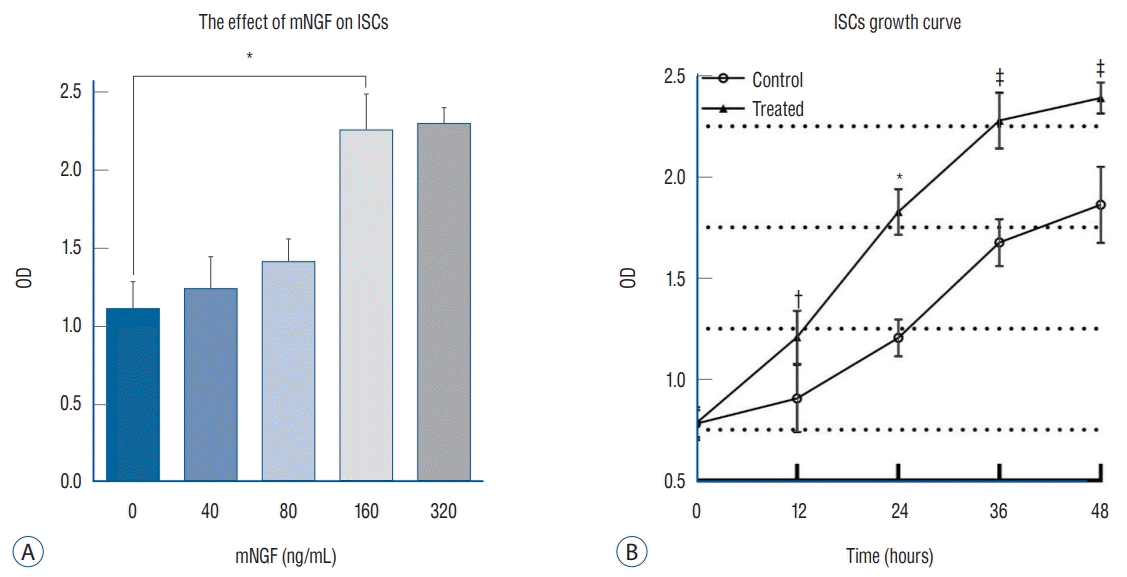
Fig. 4.
mNGF can promote the expression of TrkA. A : Western blot for evaluating TrkA and p75NTR expression in ISCs at 24 hours. TrkA gradually increased with increasing concentrations of mNGF, especially for the 160 ng/mL condition, compared with the control group (PBS), whereas the p75NTR did not significantly change between the treated group and control group. B : The statistical analysis of the TrkA and p75NTR level. The data represent the mean±standard error. *p<0.01. mNGF : mouse nerve growth factor, TrkA : tyrosine kinase A, GAPDH : glyceraldehyde-3-phosphate dehydrogenase, p75NTR : p75 neurotrophin receptor, ns : nonsignificant, PBS : phosphate buffered saline.
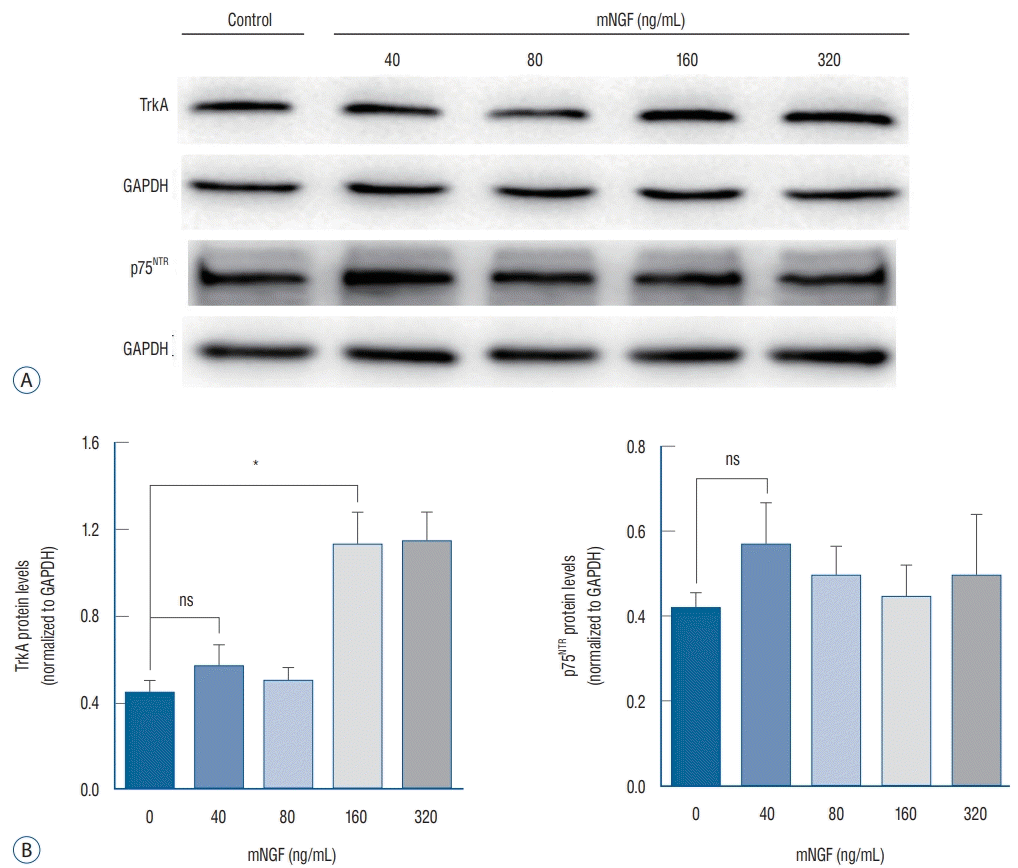
Fig. 5.
Real-time TaqMan qPCR for the mRNA expression levels in the ISCs at 24 hours. Each group normalized to GAPDH and calibrated to the sham for increasing mNGF concentrations. The mRNA levels of TrkA were significantly increased for the 160 ng/mL condition compared to the control group (PBS), but the mRNA levels of p75NTR did not significantly differ among groups, which were consistent with the results of the western blot. The data represent the mean±standard error. *p<0.05. †p< 0.01. TrkA : tyrosine kinase A, ISC : interspinal schwannoma cell, p75NTR : p75 neurotrophin receptor, ns : nonsignificant, qPCR : quantitative polymerase chain reaction, mRNA : messenger-ribonucleic acid, GAPDH : glyceraldehyde-3-phosphate dehydrogenase, mNGF : mouse nerve growth factor, PBS : phosphate buffered saline.
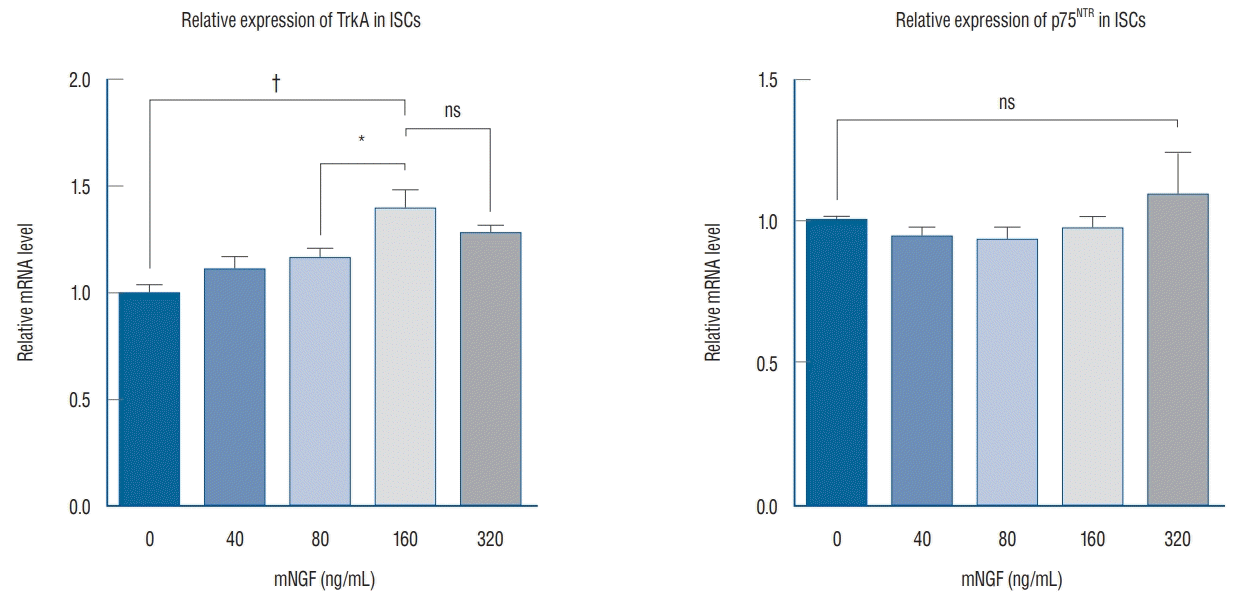
Table 1.
The sequences of gene-specific primers