Abstract
Background
Methods
Results
Notes
ACKNOWLEDGMENTS
REFERENCES













































Fig. 1.
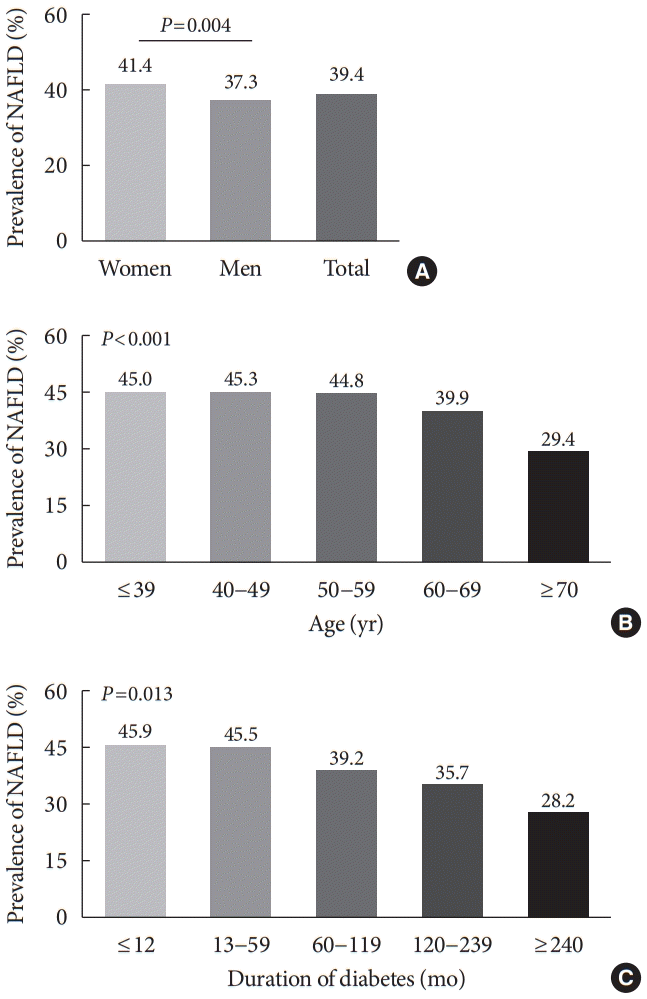
Fig. 2.
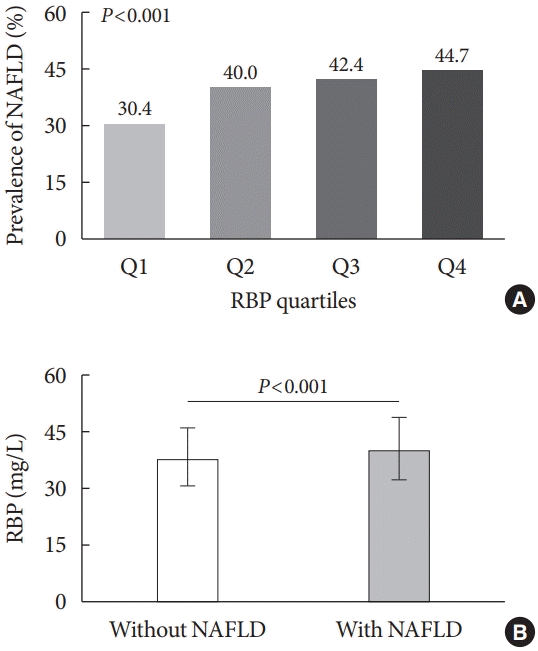
Fig. 3.
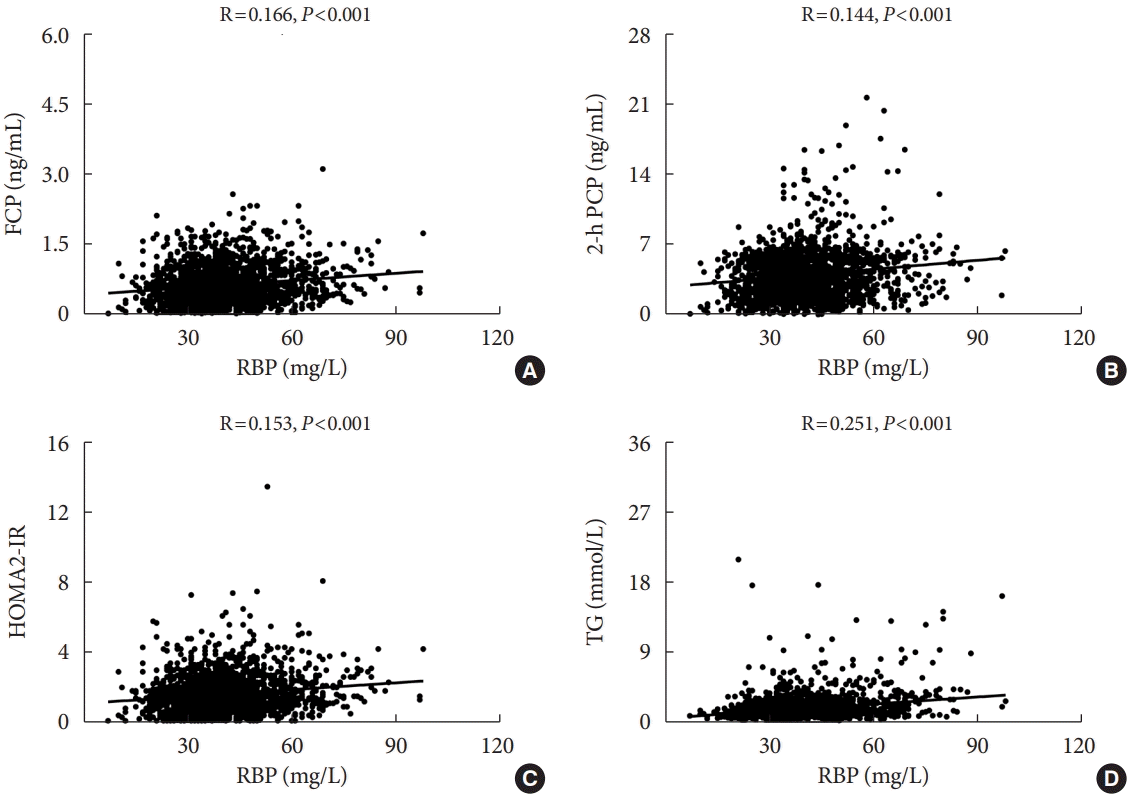
Table 1.
Variable | Q1 (n=560) | Q2 (n=545) | Q3 (n=576) | Q4 (n=582) | P value | P valuea |
---|---|---|---|---|---|---|
RBP, mg/L | <32 | 32–38 | 39–46 | ≥47 | - | - |
Male sex | 296 (52.9) | 263 (48.3) | 259 (45.0) | 265 (45.5) | 0.032 | 0.024 |
Age, yr | 61±13 | 60±13 | 60±12 | 61±12 | 0.541 | 0.355 |
DD, mo | 96 (24–144) | 84 (36–144) | 84 (24–144) | 87 (24–144) | 0.919 | 0.985 |
Hypertension | 282 (50.4) | 279 (51.2) | 313 (54.3) | 349 (60.0) | 0.004 | 0.005 |
Obesity | 231 (41.3) | 260 (47.7) | 273 (47.4) | 280 (48.1) | 0.066 | 0.066 |
Smoking | 114 (20.4) | 116 (21.3) | 111 (19.3) | 112 (19.2) | 0.800 | 0.775 |
IIA | 431 (77.0) | 389 (71.4) | 386 (67.0) | 407 (69.9) | 0.002 | 0.002 |
LLD | 134 (23.9) | 150 (27.5) | 172 (29.9) | 221 (38.0) | <0.001 | <0.001 |
Metformin | 297 (53.0) | 318 (58.3) | 326 (56.6) | 320 (55.0) | 0.325 | 0.412 |
SBP, mm Hg | 130±17 | 133±18 | 133±18 | 134±18 | 0.005 | 0.007 |
DBP, mm Hg | 79±10 | 80±10 | 80±9 | 80±10 | 0.061 | 0.065 |
WC, cm | 87.9±10.4 | 88.9±10.3 | 88.7±9.8 | 89.9±10.3 | 0.016 | 0.006 |
WHR | 0.9±0.06 | 0.91±0.06 | 0.91±0.06 | 0.91±0.06 | 0.044 | 0.009 |
BMI, kg/m2 | 24.5±3.4 | 24.8±3.5 | 24.9±3.5 | 25.0±3.5 | 0.035 | 0.036 |
WBC, ×109/L | 6 (5.2–7.3) | 6.1 (5.1–7.2) | 6.1 (5.2–7.3) | 6.2 (5.3–7.4) | 0.213 | 0.149 |
NEUT, % | 63.03±9.03 | 62.49±8.82 | 62.44±9.15 | 62.58±8.77 | 0.686 | 0.887 |
FPG, mmol/L | 7.6 (6.04–9.72) | 7.55 (6.07–9.39) | 7.64 (6.18–9.5) | 7.78 (6.2–9.48) | 0.806 | 0.875 |
2-hr PPG, mmol/L | 13.82 (10.32–17.14) | 13.29 (9.68–16.44) | 13.26 (9.84–16.8) | 13.18 (9.91–16.62) | 0.251 | 0.335 |
HbA1c, % | 9.31±2.35 | 8.89±2.26 | 9.04±2.39 | 8.81±2.24 | 0.003 | 0.003 |
FCP, ng/mL | 1.44 (0.85–2.12) | 1.65 (0.99–2.39) | 1.66 (1.09–2.53) | 1.86 (1.24–2.9) | <0.001 | <0.001 |
2-hr PCP, ng/mL | 3.29 (1.78–5.08) | 3.73 (2.19–5.34) | 3.92 (2.19–5.53) | 4.07 (2.41–5.67) | <0.001 | <0.001 |
HOMA2-IR | 1.30 (0.80–1.90) | 1.4 (0.9–2.1) | 1.4 (0.9–2.2) | 1.6 (1–2.4) | <0.001 | <0.001 |
TG, mmol/L | 1.22 (0.86–1.74) | 1.35 (0.97–1.94) | 1.47 (1.02–2.08) | 1.68 (1.16–2.58) | <0.001 | <0.001 |
TC, mmol/L | 4.53±1.05 | 4.65±0.98 | 4.7±1.14 | 4.92±1.12 | <0.001 | <0.001 |
HDL-C, mmol/L | 1.13±0.33 | 1.13±0.3 | 1.13±0.3 | 1.1±0.27 | 0.198 | 0.123 |
LDL-C, mmol/L | 3.04±0.92 | 3.12±0.84 | 3.13±0.99 | 3.2±0.98 | 0.044 | 0.074 |
Lp(a) | 8.1 (4.7–17.1) | 9.6 (5.3–17.7) | 9.8 (5.63–18.88) | 11.75 (6.37–23.33) | <0.001 | <0.001 |
ALT, U/L | 16 (12–26) | 17 (12–26) | 19 (14–29) | 19.5 (14–31) | <0.001 | <0.001 |
γ-GT, U/L | 21 (15–34) | 21 (15–31) | 23 (16–34) | 24 (17–36) | <0.001 | <0.001 |
Scr, μmol/L | 64 (53–79) | 65 (54–78) | 65 (54–78) | 67 (55–86) | 0.014 | <0.001 |
SUA, μmol/L | 295 (242–355) | 307 (257–366) | 313 (254–374) | 325 (277–383) | <0.001 | <0.001 |
UAE, mg/day | 10.73 (6.28–23.28) | 11.15 (6.6–26.11) | 10.77 (6.35–29.1) | 12.32 (7.02–50.23) | 0.001 | <0.001 |
eGFR, mL/min/1.73 m2 | 112 (91–137) | 109 (92–131) | 108 (90–131) | 104 (81–129) | <0.001 | <0.001 |
CRP, mg/L | 1.09 (0.44–3.39) | 1.1 (0.48–2.96) | 1.1 (0.5–2.72) | 1.22 (0.54–3.01) | 0.727 | 0.781 |
Values are presented as number (%), mean±standard deviation, or median (interquartile range). The P values were not adjusted for sex for the trend.
RBP, retinol-binding protein; DD, diabetes duration; IIA, insulin or insulin analogue; LLD, lipid-lowering drug; SBP, systolic blood pressure; DBP, diastolic blood pressure; WC, waist circumference; WHR, waist-to-hip ratio; BMI, body mass index; WBC, white blood cell; NEUT, neutrophils; FPG, fasting plasma glucose; PPG, postprandial plasma glucose; HbA1c, glycated hemoglobin A1c; FCP, fasting C-peptide; PCP, postprandial C-peptide; HOMA2-IR, homeostasis model assessment of insulin resistance; TG, triglycerides; TC, total cholesterol; HDL-C, high-density lipoprotein cholesterol; LDL-C, low-density lipoprotein cholesterol; Lp(a), lipoprotein(a); ALT, alanine aminotransferase; γ-GT, γ-glutamyltransferase; Scr, serum creatinine; SUA, serum uric acid; UAE, urinary albumin excretion; eGFR, estimated glomerular filtration rate; CRP, C-reactive protein.
Table 2.
Model 1: adjusted for age, sex, diabetes duration, smoking, hypertension, and obesity. Model 2: further adjusted for the use of lipid-lowering drugs, metformin, and insulin or insulin analogues. Model 3: further adjusted for systolic blood pressure, diastolic blood pressure, waist circumference, waist-to-hip ratio, and body mass index. Model 4: further adjusted for white blood cell, neutrophils, total cholesterol, triglycerides, low-density lipoprotein cholesterol, high-density lipoprotein cholesterol, lipoprotein(a), serum creatinine, estimated glomerular filtration rate, serum uric acid, urinary albumin excretion, C-reactive protein, glycated hemoglobin A1c, fasting plasma glucose, 2-hour postprandial plasma glucose, fasting C-peptide, 2-hour postprandial C-peptide, and homeostasis model assessment of insulin resistance.
RBP, retinol-binding protein; NAFLD, nonalcoholic fatty liver disease; OR, odds ratio; CI, confidence interval.
Table 3.
B statistic | OR | 95% CI | P value | |
---|---|---|---|---|
Model 1 | 0.224 | 1.251 | 1.136–1.377 | <0.001 |
Model 2 | 0.197 | 1.218 | 1.103–1.344 | <0.001 |
Model 3 | 0.194 | 1.215 | 1.091–1.353 | <0.001 |
Model 4 | 0.144 | 1.155 | 1.012–1.318 | 0.033 |
Model 1: adjusted for age, sex, diabetes duration, smoking, hypertension, and obesity. Model 2: further adjusted for the use of lipid-lowering drugs, metformin, and insulin or insulin analogues. Model 3: further adjusted for systolic blood pressure, diastolic blood pressure, waist circumference, waist-to-hip ratio, and body mass index. Model 4: further adjusted for white blood cell, neutrophils, total cholesterol, triglycerides, low-density lipoprotein cholesterol, high-density lipoprotein cholesterol, lipoprotein(a), serum creatinine, estimated glomerular filtration rate, serum uric acid, urinary albumin excretion, C-reactive protein, glycated hemoglobin A1c, fasting plasma glucose, 2-hour postprandial plasma glucose, fasting C-peptide, 2-hour postprandial C-peptide, and homeostasis model assessment of insulin resistance.
RBP, retinol-binding protein; NAFLD, nonalcoholic fatty liver disease; OR, odds ratio; CI, confidence interval.