Abstract
Experimental autoimmune uveitis (EAU), an animal model of human uveitis, is characterized by infiltration of autoimmune T cells in the uvea as well as in the retina of susceptible animals. EAU is induced by the immunization of uveitogenic antigens, including either retinal soluble-antigen or interphotoreceptor retinoid-binding proteins, in Lewis rats. The pathogenesis of EAU in rats involves the proliferation of autoimmune T cells in peripheral lymphoid tissues and breakdown of the blood-retinal barrier, primarily in the uvea and retina, finally inducing visual dysfunction. In this review, we describe recent EAU studies to facilitate the design of a therapeutic strategy through the interruption of uveitogenic factors during the course of EAU, which will be helpful for controlling human uveitis.
Uveitis is an inflammatory eye disease related to visual dysfunction in humans [1] and animals [2]. Uveitis is classified into anterior uveitis (iritis), intermediate uveitis (cyclitis), posterior uveitis (choroiditis and retinitis), and pan-uveitis (all layers of the uvea are inflamed) by anatomical structure [3]. Human non-infectious uveitis is strongly associated with human leukocyte antigen (HLA) genes and autoimmune T cells in the protein of retina [1, 4]. Autoimmune uveitis association of HLA genes causes birdshot retinochoroidopathy, Behcet’s disease, anterior uveitis in ankylosing spondylitis, Vogt-Koyanagi-Harada disease, sympathetic ophthalmia, intermediate uveitis and pars planitis [4]. Experimental autoimmune uveitis (EAU) is an animal model of inflammatory autoimmune uveitis, and is characterized by infiltration of autoimmune T cells in the retina and uvea in susceptible animals, including rats [5, 6] and mice [7]. EAU-induced rats are showed the monophasic and spontaneous pan-uveitis [8]. Furthermore, HLA transgenic mice indicate the pan-uveitis, vasculitis, granuloma formation, photoreceptor damage, and retinal folding and/or detachment [4, 9]. The neuropathogenesis of EAU is complex and involves multiple factors, including proliferation of autoimmune T cells, activation of antigen-presenting cells, homing of autoimmune T cells to the eyes with concurrent activation of adhesion molecules, inflammation in the uvea and retina with glial activation, and finally disruption of visual function and occasionally blindness [5-7].
Here we summarize key aspects of the pathogenesis of EAU in a rat model [5, 8, 10], which involves T-cell proliferation, pro-inflammatory mediators, migration of inflammatory cells through the blood-retina barrier (BRB), and tissue damage in the uvea and retina after immunization with a uveitogenic antigen.
Bovine retinal homogenate including a variety of retinal antigens is associated with the generation of uveitogenic autoimmune T cells in Lewis rats [11]. The susceptibility of inbred rat strains and pathogenic peptides has been analyzed in Lewis rats [10, 12].
In retinal tissues, two different uveitognenic antigens have been reported in Lewis rats; namely, retinal soluble-antigen (SAg) peptide and interphotoreceptor retinoid-binding protein (IRBP)-peptide R14 (common amino acids [aa] 1169–1191) or R16 (aa 1177–1191) [8, 10]. SAg is closely associated with phototransduction, whereas IRBP is a glycoprotein that transports retinoids [13]. Retinal SAg peptide-responsive T cells infiltrate the uvea and destroy the retinal pigment epithelium (RPE), whereas IRBP-reactive T cells preferentially infiltrate the retina via the BRB and destroy photoreceptors, suggesting that T cells responsive to the two antigens invade the retina via different routes [8]. Thus, SAg-derived peptide (aa 341–354)-induced EAU in Lewis rat shows monophasic clinical signs, whereas IRBP R14-immunized Lewis rat re-induce ocular inflammation after prior disease inoculation with IRBP R14 or adoptive transfer of R14-specific T cells [8, 14].
As in other autoimmune diseases in Lewis rats, T cells expressing CD4+, Th17, or CD8+ are generated in the peripheral immune system and localize to the eyes [15]. Cytokines secreted by CD4+ T cells are important in the induction of EAU, and restoration of the Th17-to-regulatory T cell (Treg) balance leads to recovery of EAU [15, 16]. Regarding whether the proliferation of autoimmune T cells is involved in the initiation of autoimmune uveitis, suppression of T-cell proliferation is one method of disease prevention. Therefore, reducing the levels of pro-inflammatory mediators is associated with the amelioration of EAU.
Following clonal expansion of T cells in response to IRBP, Vβ8.2+ T cells predominate [13], as is the case in EAE [17]. Furthermore, T-cell receptor (TCR) Vβ8.2+ and TCR Vα2+ cells predominantly infiltrate the anterior chamber, including the iris/ciliary body, in myelin basic protein-immunized anterior uveitis models [18]. Clonal expansion of Vβ8.2+ T cells is important in EAE and EAU in Lewis rats, despite them accounting for a small proportion of the total T-cell population.
In SAg-induced EAU in rats, modification of uveitis via the inhibition of T-cell proliferation is acquired by a variety of disease modifying factors, including dexamethasone, cyclosporine A, gallium nitrate, fenofibrate, and poly-nanoparticles encapsulating betamethasone phosphate [19-24]. In brief, gallium nitrate, which is used to treat hypercalcemia, mitigates the infiltration of inflammatory cells into the retina and the destruction of photoreceptor cells by suppressing the proliferation of lymphocytes [20], similar to immunosuppressive drugs, including dexamethasone, cyclosporine A [19], and fenofibrate [24]. These findings suggest that suppression of autoimmune T-cell proliferation is a primary target for the amelioration of EAU as far as an EAU model is involved.
Regarding the vascular supply in the eyes, immune cells (T cells and macrophages) infiltrate the ciliary body, iris, and uvea via the short- and long-posterior ciliary arteries after the generation of autoimmune T cells even though the BRB is tightly formed (Fig. 1) [25]. Thus, blocking the passage of inflammatory cells through the BRB in the eyes would be an alternative choice for the suppression of EAU.
In brief, immunization with uveitogenic antigens (e.g., SAg and IRBP) activates CD4+ T cells in peripheral lymph nodes, finally infiltrating the eyes via the BRB [26]. EAU in Lewis rats is characterized by the infiltration of CD4+ Th1 cells and intraocular inflammation in the anterior and posterior chambers, leading to retinal destruction. Autoreactive T cells infiltrate the uvea and retina as follows: peripheral induction of cross-reactive immune responses in peripheral lymph nodes, infiltration of cross-reactive T cells into the eye, and recruitment of inflammatory cells, including T cells and macrophages [5].
In the induction stages of EAU, lymphocytic and monocytic infiltration occurs predominantly in the anterior and posterior chambers of the eye, followed by the retina [27]. Activated microglia as well as mononuclear cells are frequently found in the perivascular cuffing region and outer layer of the retina [28], which secrete pro-inflammatory cytokines such as tumor necrosis factor (TNF)-α [28].
The BRB consists of the retinal vascular endothelium and the RPE [29]. In SAg-induced EAU, the BRB is destroyed in the posterior retina and in the peripheral retina 8 to 10 days after immunization [30]. Pro-inflammatory cytokines, including TNF-α and interleukin (IL)-1β, are strongly associated with ocular inflammation [30]. SAg-specific T cells were detected in the iris, ciliary body, and aqueous humor 2 days after cell transfer [31]. Therefore, activated T cells sequentially infiltrate the iris, ciliary body, aqueous humor, and retina in rats with SAg-induced EAU.
In IRBP-induced uveitis, inflammatory cells infiltrate the retina via retinal blood vessels, which are derived from the ophthalmic artery, and destroy photoreceptors (Fig. 1). CD4+ T cells infiltrate the choroid adjacent to the RPE in IRBP R14-induced EAU rats [14]. Furthermore, the CD4+/CD8+ and Th17/Treg ratios were elevated on day 12 after immunization of IRBP-induced EAU rats with severe ocular inflammation [15]. It has been postulated that either IRBP and/or SAg generate autoimmune uveitogenic T cells; IRBP-reactive T cells preferentially infiltrate the neural retina while Sag-responsive T cells migrate to the uvea as far as the effector phage of EAU pathogenesis. Irrespective of preferential infiltration into either the neural retina or uvea, retinal involvement of autoimmune damage is similar as far as rat EAU is involved.
The BRB comprises the inner and outer BRB and disruption of its permeability triggers ocular inflammation. The outer BRB, a single layer of cuboidal RPE cells, is located between the optic part of the retina and the finest capillaries in the choroid capillary lamina [32]. The choroidal capillaries are highly permeable, allowing infiltration of inflammatory cells—including autoreactive T cells, macrophages, and neutrophils—into the posterior and peripheral retina [30]. Furthermore, the RPE releases pro-inflammatory cytokines (TNF-α, IL-6, and IL-8), which induce uveitis by increasing mitochondrial oxidative stress in the photoreceptors of EAU-induced rats [33]. Degeneration of RPE cells is associated with breakdown of the retinal structure, such as photoreceptor cell death, resulting in visual dysfunction [34]. The proliferation and phenotype switching of RPE cells, i.e., the epithelial–mesenchymal transition (EMT), is a feature of retinal disorders [29]. The EMT in RPE cells is increased by treatment with diesel particulate matter and transforming growth factor (TGF)-β is upregulated [35]. The EMT promotes retinal detachment by upregulating matrix metalloproteinases (MMPs), which degrade tight-junction proteins, e.g., zonula occludens-1 [36]. We postulate that EMT of RPE cells promotes retinal detachment, leading to loss of visual acuity in EAU.
Therapeutic strategies for EAU comprise: (1) inhibition of lymphocyte proliferation, (2) suppression of TNF and cytokines, (3) downregulation of oxidative stress/radicals, and (4) inhibition of cell migration (Table 1).
Because autoimmune T cells primarily infiltrate eyes with EAU, it is plausible that the suppression of clonal expansion of uveitogenic T cells and/or broad suppression of T-cell proliferation is a primary therapeutic target. Among the V beta genes, TCR Vβ genes, including TCR Vβ 8.3, are targets because immunization with its peptides is associated with the prevention of ocular inflammation and suppression of lymphocyte proliferation, with concurrent increased levels of the anti-inflammatory cytokines IL-4 and IL-10 [37]. Furthermore, high-dose dexamethasone hydrogel downregulates Th1 and Th17 responses, such as the release of TNF-α, IL-6, and IL-17 [38]. In addition, autoreactive T cells and other inflammatory cells release pro-inflammatory cytokines, including ILs, interferons (IFN), and cytokine (C-C motif) ligand, in animal models of EAU [8, 15, 30]. Therefore, inhibition of lymphocytes such as T cells has therapeutic potential for EAU.
These findings indicate that suppression of T-cell proliferation is closely linked to the amelioration of ocular inflammation with the suppression of pro-inflammatory mediators.
TNF signaling and pro- and anti-inflammatory cytokines are associated with intraocular inflammation, macrophage infiltration, apoptosis in the retina, and Müller cell activation [39-41]. A soluble fusion protein of TNF p55 receptor suppressed tissue damage without impeding myeloid cell infiltration or macrophage infiltration [39]. Suppression of TNF signaling prevented apoptosis and production of nitric oxide (NO), and promoted synthesis of TGF-β, in the retina [39]. In addition, adalimumab and infliximab, anti-TNF-α agents, suppressed uveitis by blocking interactions with TNF receptor and exerted a cytotoxic effect on TNF-α-expressing cells in endotoxin-induced disruption of the blood–aqueous barrier [42]. Inhibition of TNF signaling mitigated uveitis by suppressing the infiltration of inflammatory cells, apoptosis, and oxidative stress.
Once inflammation is initiated in the ocular tissues, oxidative stress follows because a variety of inflammatory cells and resident cells react to exogenous insults, possibly the generation of radicals [33, 43, 44]. This non-specific generation of radicals, in excess, damages target organs, including the eyes. Thus, the generation of radical scavenging enzymes, including superoxide dismutase and catalase, is an alternative choice to mitigate injury [45].
Allopurinol, a xanthine oxidase inhibitor used to treat gout and kidney stones, inhibited inflammatory changes, including thinning, loss of retinal ganglion cells, and retinal detachment, by suppressing lipid peroxidation and myeloperoxidase activity [46]. 4-Hydroxy-tempo, also known as nitroxide 4-hydroxy-2,2,6,6,-tetramethylpiperidine-1-N-oxyl (TPL), has superoxide dismutase activity [47]. In human SAg-induced EAU, TPL suppressed the inflammatory changes and reduced the protein and retinal-conjugated malondialdehyde levels in the aqueous humor [48]. Fidarestat, an aldose reductase inhibitor, inhibited the increased levels of inducible NO synthase and cyclooxygenase, thereby suppressing EAU-induced ocular inflammatory changes, including upregulation of cytokines and chemokines [49]. Furthermore, NO inhibitors such as molsidomine improved the histopathological changes and clinical signs by increasing the levels of anti-inflammatory cytokines such as IL-10 and decreasing IFN-γ levels [40]. Oxidative stress in mitochondria of photoreceptor inner segments triggers retinal inflammation at an early stage in SAg-induced EAU Lewis rats [44]. RPE releases pro-inflammatory cytokines, including TNF-α, IL-6, and IL-8, which are uveitogenic [33]. We postulate that reducing oxidative stress or free radical levels in retinal pigment cells mitigates ocular inflammation in the early phase of EAU.
Infiltration of autoimmune T cells into the target organ is important because cells in the bloodstream itself do not induce target specific disease. Blocking cell migration is a choice because blocking cell adhesion molecules, including VLA-4, abolishes autoimmune central nervous system inflammation [50]. If this is the case, it is highly possible that blocking adhesion molecules is a possibility. Fidarestat prevented ocular inflammation by preventing the induction of intercellular adhesion molecule-1 (ICAM-1) expression in the retinal endothelium [49]. A monoclonal antibody to ICAM-1 completely inhibited its upregulation in the ciliary body, iris, and retinal pigment epithelium, and mitigated the inflammatory responses [51]. In addition, a neutralizing monoclonal antibody to macrophage migration inhibitory factor inhibited clinical signs of EAU by suppressing T-cell proliferation [52]. The levels of MMPs and neutrophil collagenase are increased in EAU-induced retina, and inhibition of MMPs by BB-1101 reduced the incidence of disease and abolished leukocyte infiltration and retinal tissue damage [53]. ICAM-1, the ligand for lymphocyte function-associated antigen-1, and vascular cell adhesion molecule-1 were upregulated in superficial retinal vessels before T-cell infiltration EAU-induced rats [33]. Therefore, we postulate that suppression of adhesion molecules, including ICAM-1, prevents induction of EAU.
This review summarizes that the pathogenesis of EAU involves the proliferation of autoimmune T cells, with concurrent production of proinflammatory cytokines and migration of CD4+ T-cells into eyes. Disruption of both T-cell proliferation and migration would be a primary target for the modulation of EAU. Once the inflammatory response in the eyes occurs in EAU, radical scavenging is an alternative choice for the treatment of EAU. Importantly, it is postulated that the RPE is an important barrier in the BRB as far as EAU is concerned, because it controls the trans-passage of inflammatory cells in addition to contributing to retinal homeostasis between the choroid and outer segment of the retina.
Acknowledgements
This research was supported by the National Research Foundation of Korea (Grant number: NRF- 2019R1A2C1087753) and a grant from Kosin University College of Medicine (2021).
Notes
References
1. Jabs DA. 2018; Immunosuppression for the uveitides. Ophthalmology. 125:193–202. DOI: 10.1016/j.ophtha.2017.08.007. PMID: 28942074. PMCID: PMC5794515.


2. Chmielewski NT, Brooks DE, Smith PJ, Hendrix DV, Whittaker C, Gelatt KN. 1997; Visual outcome and ocular survival following iris prolapse in the horse: a review of 32 cases. Equine Vet J. 29:31–9. DOI: 10.1111/j.2042-3306.1997.tb01633.x. PMID: 9031861.


3. de Smet MD, Taylor SR, Bodaghi B, Miserocchi E, Murray PI, Pleyer U, Zierhut M, Barisani-Asenbauer T, LeHoang P, Lightman S. 2011; Understanding uveitis: the impact of research on visual outcomes. Prog Retin Eye Res. 30:452–70. DOI: 10.1016/j.preteyeres.2011.06.005. PMID: 21807112.


4. Mattapallil MJ, Silver PB, Mattapallil JJ, Horai R, Karabekian Z, McDowell JH, Chan CC, James EA, Kwok WW, Sen HN, Nussenblatt RB, David CS, Caspi RR. 2011; Uveitis-associated epitopes of retinal antigens are pathogenic in the humanized mouse model of uveitis and identify autoaggressive T cells. J Immunol. 187:1977–85. DOI: 10.4049/jimmunol.1101247. PMID: 21765017. PMCID: PMC3150271.


5. Wildner G, Diedrichs-Mohring M, Thurau SR. 2008; Rat models of autoimmune uveitis. Ophthalmic Res. 40:141–4. DOI: 10.1159/000119865. PMID: 18421228.


6. Caspi RR. 2014; Understanding autoimmunity in the eye: from animal models to novel therapies. Discov Med. 17:155–62. PMID: 24641958. PMCID: PMC4573559.
7. Klaska IP, Forrester JV. 2015; Mouse models of autoimmune uveitis. Curr Pharm Des. 21:2453–67. DOI: 10.2174/1381612821666150316122928. PMID: 25777760.


8. Diedrichs-Möhring M, Kaufmann U, Wildner G. 2018; The immunopathogenesis of chronic and relapsing autoimmune uveitis - lessons from experimental rat models. Prog Retin Eye Res. 65:107–26. DOI: 10.1016/j.preteyeres.2018.02.003. PMID: 29496590.


9. Kyger M, Worley A, Huan J, McDowell H, Smith WC, Burrows GG, Mattapallil MJ, Caspi RR, Adamus G. 2013; Effective arrestin-specific immunotherapy of experimental autoimmune uveitis with RTL: a prospect for treatment of human uveitis. Transl Vis Sci Technol. 2:1. DOI: 10.1167/tvst.2.2.1. PMID: 24049712. PMCID: PMC3763891.


10. Agarwal RK, Silver PB, Caspi RR. 2012; Rodent models of experimental autoimmune uveitis. Methods Mol Biol. 900:443–69. DOI: 10.1007/978-1-60761-720-4_22. PMID: 22933083. PMCID: PMC3810964.


11. Kang J, Ahn M, Moon C, Min DS, Matsumoto Y, Shin T. 2005; Phospholipase D1 is up-regulated in the retina of Lewis rats with experimental autoimmune uveoretinitis. Immunol Invest. 34:27–36. DOI: 10.1081/imm-200047381. PMID: 15773570.


12. Caspi RR. 2003; Experimental autoimmune uveoretinitis in the rat and mouse. Curr Protoc Immunol. Chapter 15:Unit 15.6. DOI: 10.1002/0471142735.im1506s53. PMID: 18432901.


13. Egwuagu CE, Mahdi RM, Nussenblatt RB, Gery I, Caspi RR. 1993; Evidence for selective accumulation of V beta 8+ T lymphocytes in experimental autoimmune uveoretinitis induced with two different retinal antigens. J Immunol. 151:1627–36. PMID: 8393049.
14. Diedrichs-Möhring M, Hoffmann C, Wildner G. 2008; Antigen-dependent monophasic or recurrent autoimmune uveitis in rats. Int Immunol. 20:365–74. DOI: 10.1093/intimm/dxm148. PMID: 18203685.


15. Yin X, Liu B, Wei H, Wu S, Guo L, Xu F, Liu T, Bi H, Guo D. 2019; Activation of the Notch signaling pathway disturbs the CD4+/CD8+, Th17/Treg balance in rats with experimental autoimmune uveitis. Inflamm Res. 68:761–74. DOI: 10.1007/s00011-019-01260-w. PMID: 31209505.


16. Jia X, Hu M, Wang C, Wang C, Zhang F, Han Q, Zhao R, Huang Q, Xu H, Yuan H, Ren H. 2011; Coordinated gene expression of Th17- and Treg-associated molecules correlated with resolution of the monophasic experimental autoimmune uveitis. Mol Vis. 17:1493–507. PMID: 21686325. PMCID: PMC3115744.
17. Kim G, Kohyama K, Tanuma N, Arimito H, Matsumoto Y. 1998; Persistent expression of experimental autoimmune encephalomyelitis (EAE)-specific Vbeta8.2 TCR spectratype in the central nervous system of rats with chronic relapsing EAE. J Immunol. 161:6993–8. PMID: 9862735.
18. Buenafe AC, Offner H, Machnicki M, Elerding H, Adlard K, Jacobs R, Vandenbark AA, Adamus G. 1998; EAE TCR motifs and antigen recognition in myelin basic protein-induced anterior uveitis in Lewis rats. J Immunol. 161:2052–9. PMID: 9712079.
19. Chan CC, Caspi R, Mochizuki M, Diamantstein T, Gery I, Nussenblatt RB. 1987; Cyclosporine and dexamethasone inhibit T-lymphocyte MHC class II antigens and IL-2 receptor expression in experimental autoimmune uveitis. Immunol Invest. 16:319–31. DOI: 10.3109/08820138709087087. PMID: 3123380.


20. Lobanoff MC, Kozhich AT, Mullet DI, Gerber N, Gery I, Chan CC, Whitcup SM. 1997; Effect of gallium nitrate on experimental autoimmune uveitis. Exp Eye Res. 65:797–801. DOI: 10.1006/exer.1997.0395. PMID: 9441703.


21. Toriyama S. 2000; Effects of leukotriene B(4) receptor antagonist on experimental autoimmune uveoretinitis in rats. Jpn J Ophthalmol. 44:695. DOI: 10.1016/s0021-5155(00)00262-8. PMID: 11094206.


22. Liang W, Karabekian Z, Mattapallil M, Xu Q, Viley AM, Caspi R, Scott DW. 2006; B-cell delivered gene transfer of human S-Ag-Ig fusion protein protects from experimental autoimmune uveitis. Clin Immunol. 118:35–41. Erratum in: Clin Immunol 2006;120:357. DOI: 10.1016/j.clim.2005.08.007. PMID: 16168712.


23. Sakai T, Kohno H, Ishihara T, Higaki M, Saito S, Matsushima M, Mizushima Y, Kitahara K. 2006; Treatment of experimental autoimmune uveoretinitis with poly(lactic acid) nanoparticles encapsulating betamethasone phosphate. Exp Eye Res. 82:657–63. DOI: 10.1016/j.exer.2005.09.003. PMID: 16360654.


24. Osada M, Sakai T, Kuroyanagi K, Kohno H, Tsuneoka H. 2014; Treatment of experimental autoimmune uveoretinitis with peroxisome proliferator-activated receptor α agonist fenofibrate. Mol Vis. 20:1518–26. PMID: 25489225. PMCID: PMC4225135.
25. Kim J, Ahn M, Choi Y, Chun J, Jung K, Tanaka A, Matsuda H, Shin T. 2022; Osteopontin is a biomarker for early autoimmune uveoretinitis. Neural Regen Res. 17:1604–8. DOI: 10.4103/1673-5374.330614. PMID: 34916447. PMCID: PMC8771122. PMID: e6f5553cb10f43f9a532d74599473734.


26. Dick AD, Cheng YF, Liversidge J, Forrester JV. 1994; Immunomodulation of experimental autoimmune uveoretinitis: a model of tolerance induction with retinal antigens. Eye (Lond). 8(Pt 1):52–9. DOI: 10.1038/eye.1994.10. PMID: 8013720.


27. Greenwood J, Howes R, Lightman S. 1994; The blood-retinal barrier in experimental autoimmune uveoretinitis. Leukocyte interactions and functional damage. Lab Invest. 70:39–52. PMID: 8302017.
28. Rao NA, Kimoto T, Zamir E, Giri R, Wang R, Ito S, Pararajasegaram G, Read RW, Wu GS. 2003; Pathogenic role of retinal microglia in experimental uveoretinitis. Invest Ophthalmol Vis Sci. 44:22–31. DOI: 10.1167/iovs.02-0199. PMID: 12506051.


29. Chiba C. 2014; The retinal pigment epithelium: an important player of retinal disorders and regeneration. Exp Eye Res. 123:107–14. DOI: 10.1016/j.exer.2013.07.009. PMID: 23880527.


30. Luna JD, Chan CC, Derevjanik NL, Mahlow J, Chiu C, Peng B, Tobe T, Campochiaro PA, Vinores SA. 1997; Blood-retinal barrier (BRB) breakdown in experimental autoimmune uveoretinitis: comparison with vascular endothelial growth factor, tumor necrosis factor alpha, and interleukin-1beta-mediated breakdown. J Neurosci Res. 49:268–80. DOI: 10.1002/(sici)1097-4547(19970801)49:3<268::aid-jnr2>3.0.co;2-a. PMID: 9260738.
31. Prendergast RA, Iliff CE, Coskuncan NM, Caspi RR, Sartani G, Tarrant TK, Lutty GA, McLeod DS. 1998; T cell traffic and the inflammatory response in experimental autoimmune uveoretinitis. Invest Ophthalmol Vis Sci. 39:754–62. PMID: 9538882.
32. Tyler NK, Burns MS. 1991; Comparison of lectin reactivity in the vessel beds of the rat eye. Curr Eye Res. 10:801–10. DOI: 10.3109/02713689109013875. PMID: 1790711.


33. Dick AD, Forrester JV, Liversidge J, Cope AP. 2004; The role of tumour necrosis factor (TNF-alpha) in experimental autoimmune uveoretinitis (EAU). Prog Retin Eye Res. 23:617–37. DOI: 10.1016/j.preteyeres.2004.06.005. PMID: 15388077.
34. Pavan B, Dalpiaz A. 2018; Retinal pigment epithelial cells as a therapeutic tool and target against retinopathies. Drug Discov Today. 23:1672–9. DOI: 10.1016/j.drudis.2018.06.009. PMID: 29908265.


35. Lee H, Hwang-Bo H, Ji SY, Kim MY, Kim SY, Park C, Hong SH, Kim GY, Song KS, Hyun JW, Choi YH. 2020; Diesel particulate matter2.5 promotes epithelial-mesenchymal transition of human retinal pigment epithelial cells via generation of reactive oxygen species. Environ Pollut. 262:114301. DOI: 10.1016/j.envpol.2020.114301. PMID: 32155554.


36. Giebel SJ, Menicucci G, McGuire PG, Das A. 2005; Matrix metalloproteinases in early diabetic retinopathy and their role in alteration of the blood-retinal barrier. Lab Invest. 85:597–607. DOI: 10.1038/labinvest.3700251. PMID: 15711567.


37. Zhang R, Yang PZ, Wu CY, Jin HL, Li B, Huang XK, Zhou HY, Gao Y, Zhu LX, Kijlstra A. 2006; Role of T-cell receptor V beta 8.3 peptide vaccine in the prevention of experimental autoimmune uveoretinitis. Chin Med J (Engl). 119:740–8. DOI: 10.1097/00029330-200605010-00006. PMID: 16701014.


38. Wu W, Zhang Z, Xiong T, Zhao W, Jiang R, Chen H, Li X. 2017; Calcium ion coordinated dexamethasone supramolecular hydrogel as therapeutic alternative for control of non-infectious uveitis. Acta Biomater. 61:157–68. DOI: 10.1016/j.actbio.2017.05.024. PMID: 28501709.


39. Robertson M, Liversidge J, Forrester JV, Dick AD. 2003; Neutralizing tumor necrosis factor-alpha activity suppresses activation of infiltrating macrophages in experimental autoimmune uveoretinitis. Invest Ophthalmol Vis Sci. 44:3034–41. DOI: 10.1167/iovs.02-1156. PMID: 12824249.


40. Kwak HJ, Yang YS, Pae HO, Kim YM, Chung HT. 2001; Exogenous nitric oxide inhibits experimental autoimmune uveoretinitis development in Lewis rats by modulation of the Th1-dependent immune response. Mol Cells. 12:178–84. PMID: 11710518.
41. Sakai T, Ishihara T, Higaki M, Akiyama G, Tsuneoka H. 2011; Therapeutic effect of stealth-type polymeric nanoparticles with encapsulated betamethasone phosphate on experimental autoimmune uveoretinitis. Invest Ophthalmol Vis Sci. 52:1516–21. DOI: 10.1167/iovs.10-5676. PMID: 21178146.


42. Johnsen-Soriano S, Sancho-Tello M, Arnal E, Díaz-Llopis M, Navea A, Miranda M, Bosch-Morell F, Romero FJ. 2010; Comparison of the acute effects of anti-TNF-alpha drugs on a uveitis experimental model. Ocul Immunol Inflamm. 18:208–15. DOI: 10.3109/09273940903521964. PMID: 20482400.


43. Crupi R, Impellizzeri D, Gugliandolo E, Cordaro M, Siracusa R, Britti D, Cuzzocrea S, Di Paola R. 2019; Effect of Tempol, a membrane-permeable free radical scavenger, on in vitro model of eye inflammation on rabbit corneal cells. J Ocul Pharmacol Ther. 35:571–7. DOI: 10.1089/jop.2019.0016. PMID: 31825758.
44. Rajendram R, Saraswathy S, Rao NA. 2007; Photoreceptor mitochondrial oxidative stress in early experimental autoimmune uveoretinitis. Br J Ophthalmol. 91:531–7. DOI: 10.1136/bjo.2006.101576. PMID: 17035279. PMCID: PMC1994769.


45. Harzallah O, Kerkeni A, Baati T, Mahjoub S. 2008; Oxidative stress: correlation with Behçet’s disease duration, activity and severity. Eur J Intern Med. 19:541–7.


46. Augustin AJ, Loeffler KU, Sekundo W, Grus FH, Lutz J. 1999; Effects of systemically applied allopurinol and prednisolone on experimental autoimmune uveitis. Graefes Arch Clin Exp Ophthalmol. 237:508–12. DOI: 10.1007/s004170050270. PMID: 10379613.


47. Sasaki H, Lin LR, Yokoyama T, Sevilla MD, Reddy VN, Giblin FJ. 1998; TEMPOL protects against lens DNA strand breaks and cataract in the x-rayed rabbit. Invest Ophthalmol Vis Sci. 39:544–52. PMID: 9501865.
48. Zamir E, Zhang R, Samuni A, Kogan M, Pe’er J. 1999; Nitroxide stable radical suppresses autoimmune uveitis in rats. Free Radic Biol Med. 27:7–15. DOI: 10.1016/s0891-5849(99)00026-x. PMID: 10443914.


49. Yadav UC, Shoeb M, ivastava SK Sr, Ramana KV. 2011; Amelioration of experimental autoimmune uveoretinitis by aldose reductase inhibition in Lewis rats. Invest Ophthalmol Vis Sci. 52:8033–41. DOI: 10.1167/iovs.11-7485. PMID: 21900376. PMCID: PMC3208000.


50. Pluchino S, Zanotti L, Rossi B, Brambilla E, Ottoboni L, Salani G, Martinello M, Cattalini A, Bergami A, Furlan R, Comi G, Constantin G, Martino G. 2005; Neurosphere-derived multipotent precursors promote neuroprotection by an immunomodulatory mechanism. Nature. 436:266–71. DOI: 10.1038/nature03889. PMID: 16015332.


51. Uchio E, Kijima M, Tanaka S, Ohno S. 1994; Suppression of experimental uveitis with monoclonal antibodies to ICAM-1 and LFA-1. Invest Ophthalmol Vis Sci. 35:2626–31. PMID: 7909311.
52. Kitaichi N, Matsuda A, Kotake S, Namba K, Tagawa Y, Sasamoto Y, Ogasawara K, Iwabuchi K, Onoé K, Matsuda H, Nishihira J. 2000; Inhibition of experimental autoimmune uveoretinitis with anti-macrophage migration inhibitory factor antibodies. Curr Eye Res. 20:109–14. DOI: 10.1076/0271-3683(200002)2021-dft109. PMID: 10617911.


53. Wallace GR, Whiston RA, Stanford MR, Wells GM, Gearing AJ, Clements JM. 1999; The matrix metalloproteinase inhibitor BB-1101 prevents experimental autoimmune uveoretinitis (EAU). Clin Exp Immunol. 118:364–70. DOI: 10.1046/j.1365-2249.1999.01066.x. PMID: 10594553. PMCID: PMC1905444.


Fig. 1
A schematic drawing of experimental autoimmune uveitis (EAU) pathogenesis. Modified from Kim et al. Neural Regen Res 2022;17:1604-8 [25].
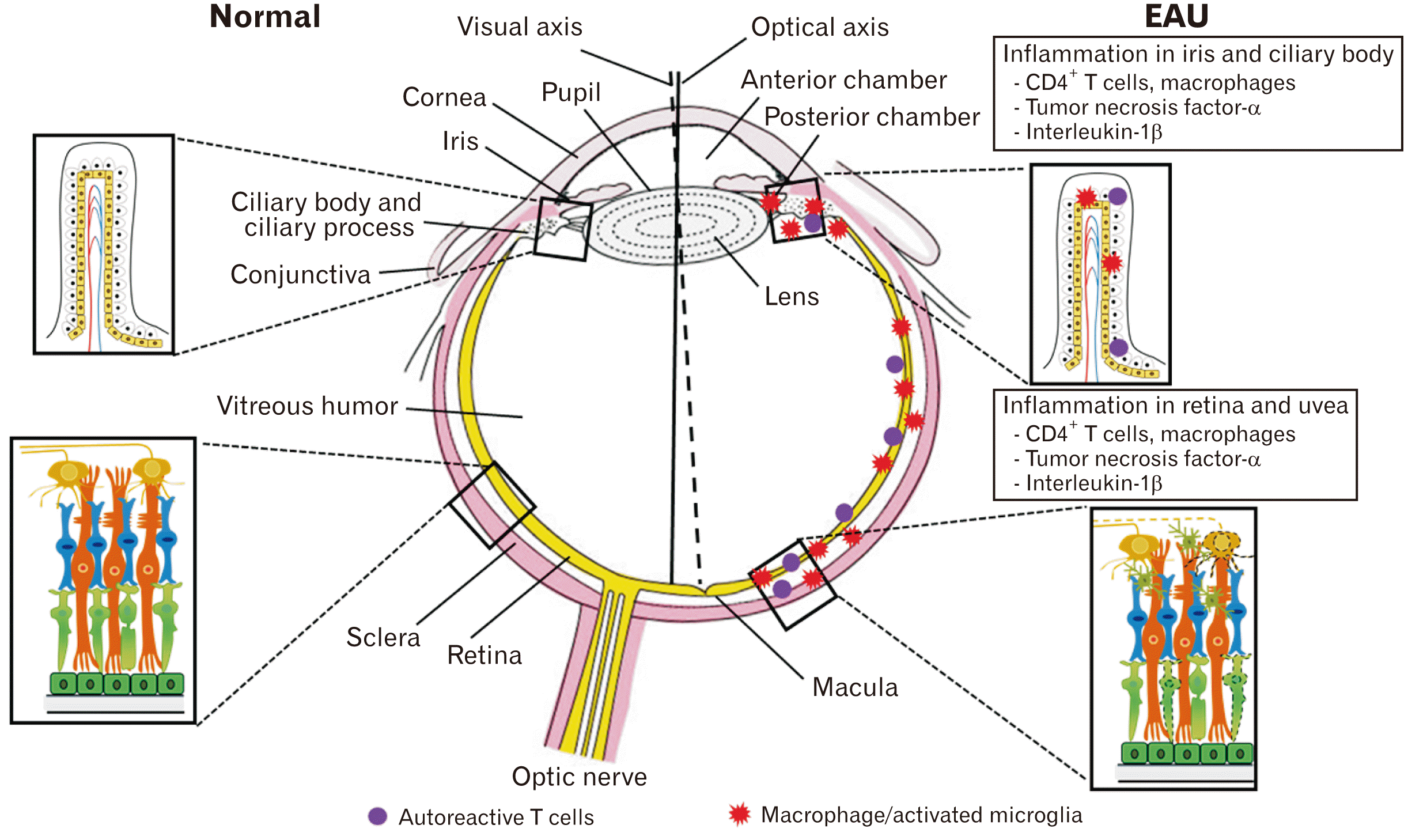
Table 1
Therapeutic effects in experimental autoimmune uveitis (EAU)
Targets of therapeutics | Therapeutic agents | Immunogen | Therapeutic outcomes | References |
---|---|---|---|---|
Lymphocytes | T cell receptor (TCR) Vβ 8.3 peptide vaccination | IRBP;1177–1191 | [33] | |
Dex sodium phosphate solution and Dex supramolecular hydrogel | Human IRBP161–180 | [34] | ||
TNF and cytokines | Soluble fusion protein of TNFp55 receptor | Soluble retina extract | [35] | |
Molsidomine (nitric oxide inhibitor) | IRBP 1169–1191 (R14) | [36] | ||
Stealth nanosteroids | SAg peptide 1–20 | [37] | ||
Adalimumab and infliximab (Anti-TNF alpha drug) | Endotoxin | [38] | ||
Oxidative stress | Allopurinol | Crude retina extract of calf | [42] | |
Nitroxide 4-hydroxy-2,2,6,6,-tetramethylpiperidine-1-N-oxyl | Human SAg | [44] | ||
Fidarestat | Bovine IRBP peptide 1169–1191 | [45] | ||
Cell migration | Fidarestat | Bovine IRBP peptide 1169–1191 | [45] | |
Monoclonal antibody to ICAM-1 (1A29) | SAg | [47] | ||
Neutralizing monoclonal antibody to macrophage migration inhibitory factor | IRBP peptide | [48] | ||
BB-1101 (MMP inhibitor) | SAg | [49] |
IRBP, interphotoreceptor retinoid-binding protein; Dex, dexamethasone; IL, interleukin; IFN, interferon; Th, helper T cell; Tx, treatment; TNF, tumor necrosis factor; TGF, tumor growth factor; SAg, soluble antigen; LPO, lipid peroxidation; MPO, myeloperoxidase; NOS, nitric oxide synthase; COX, cycloxygenase; MMP, matrix metalloproteinase.